Abstract
Much attention has been paid to the relationship between atypical femoral fractures (AFF) and use of bisphosphonates (BPs). While a significant cause-effect relationship was not established in earlier studies, more recent data shows a growing relationship between AFF and BPs use. The definition of an 'AFF' has also undergone significant changes. This review briefly summarizes the definition, pathogenesis, and management of AFF.
Recently, great attention has been paid to the possible relation between the prolonged use of bisphosphonates (BPs) and low-energy femoral subtrochanter and shaft fractures. Those fractures were named "atypical fractures" to distinguish them from "typical fractures" occurring at the femoral neck and trochanteric area from low-energy trauma. Most typical femoral shaft fractures occur from major high-energy trauma, such as traffic accidents or falls from heights. Unlike another complication of BP use, osteonecrosis of the jaw (ONJ), the atypical femoral fractures (AFF) is not associated with high doses of BPs.[1]
The first report on a possible relation between prolonged BP use and "atypical fractures" of femoral shaft was from Odvina et al.[2] They described nine patients who had fractures of the femoral shaft, proximal femur, sacrum, ischium, pubis, and ribs. Most of the patients showed delayed healing accompanied by suppressed bone turnover. All patients were treated with alendronate, some of them had received estrogen and glucocorticoids: all are known bone turnover-suppressing agents. After their first article, numerous sporadic case reports and case reviews followed and the AFF was characterized by clinical and radiological findings. Several retrospective case-controlled, and larger epidemiological studies were subsequently published.[1,3]
As a systemized approach to further define AFF, the American Society for Bone and Mineral Research (ASBMR) organized a multidisciplinary task force in 2009 and published a position paper in 2010. After reviewing published articles on the epidemiology, risk factors, imaging, and clinical managements, the task force defined AFF and concluded that the incidence of AFF was very low, particularly considering the number of spine and hip fractures that could be prevented by BP use. They also noted that a causal relationship between BP and AFF had not been established, although statistical power was lacking. According to 2010 Task Force report, AFF was defined as atraumatic or low-trauma fractures located in the subtrochanteric region or the femoral shaft. High-trauma fractures, femoral neck fractures, intertrochanteric fractures with spiral subtrochanteric extension, pathological fractures associated with primary or metastatic bone tumors, and periprosthetic fractures were excluded from the diagnosis of AFF. Other major features of AFF included transverse or short oblique configuration, non-comminuted incomplete fractures involving only the lateral cortex, while complete fractures extend through both cortices and may have a medial spike. Minor features comprise localized periosteal reaction or beaking of the lateral cortex, generalized cortical thickening of the femoral shaft, a history of prodromal pain, bilateral fractures and symptoms, and delayed healing in association with certain medication and medical conditions. All major features are needed to define a fracture as "atypical" while minor features may not be present in some cases.[1] The Food and Drug Administration (FDA) addressed the issue in September 2011. The conclusion of the report was that atypical fractures were very rare (http://www.fda.gov/downloads/AdvisoryCommittees/CommitteesMeetingMaterials/drugs/DrugSafetyandRiskManagementAdvisoryCommittee/ucm270958.pdf). The FDA report also discussed the available evidence supporting the relationship between long-term BP treatment and the risk of atypical fracture. The mounted data suggest an association between BP use and atypical fractures, although causality has not been determined.
Recently, ASBMR published an updated version of previous 2013 report that included revised criteria for AFF.[3] According to the new definition, four of the five major criteria (versus all) should be present to define an AFF. The absence of comminution was changed to "non-comminuted" or minimally comminuted, and the transverse or short oblique orientation criterion was changed to "the fracture line originates at the lateral cortex and is substantially transverse, although it may become oblique as it progresses medially across the femur." A minor criterion in the 2010 version, "localized periosteal reaction of the lateral cortex" was incorporated as a major criterion: "localized periosteal or endosteal thickening of the lateral cortex is present at the fracture site (beaking or flaring)". Reasons for changes were a positive correlation between BP use and signs of fatigue fractures, including transverse fracture lines on the lateral cortex, periosteal reactions, and a medial spike.[4] "Short oblique fracture line" was also deleted from the new criteria because the definition of short oblique may differ from one physician to another and most orthopedic surgeons consider a fracture short oblique when the angle between the transverse axis and the fracture is less than 30° (Fig. 1).[4] When "short oblique" is used to define an angle between 30 and 60°, the positive association between BP use and AFF falls dramatically.[5] Compared with the 2010 position, the 2013 ASBMR position statement further clarified the relationship between AFF and BP exposure, reporting a positive correlation between exposure time and the risk of atypical fractures. The absolute risk reported varies between studies and ranges from 3.2 to 50 cases per 100,000 person-years; longer exposure is associated with higher risk (more than 100 cases per 100,000 person-years) (Table 1).[3]
Although the pathogenesis of AFF remains largely unclear, several pathomechanisms have been proposed. The main radiological features are a transverse orientation and a lack of comminution, which are characteristics of brittle fractures. Localized cortical thickening gives clues as to "fatigue fractures." The term "fatigue fracture" means a fracture caused by unusual, excessive repetitive loading on a normal bone while an "insufficiency fracture" indicates normal loading of an abnormal or insufficient bone. In a fatigue fracture, fatigue damages, which are microcracks, are not repaired and accumulate, and ultimately coalesce to grow to a critical-size defect, precipitating complete fracture.[1,3]
One difference between AFF and exercise-induced femoral fatigue fractures is that AFF starts on the lateral aspect of the femur while the other often do on the medial side. Exercise-induced fatigue fractures result in a more oblique fracture surface than do AFF. In contrast, AFF have a smooth transverse fracture surface on lateral side, more characteristic of a brittle material.[3]
The toughness of bone, the intrinsic energy absorption capacity, is determined principally by the organic matrix, which consists chiefly of collagen.[6,7,8] Enzymatic and nonenzymatic collagen cross-links exist in bone collagen, stabilizing the bone matrix. Enzymatic cross-links are trivalent cross-links, between pyridinoline (PYD), deoxypyridinoline (DPD), and pyrroles, compared with nonenzymatic cross-links are formed through the interaction of collagen and sugars via oxidation reactions. Disturbances in these links significantly affect the bone's mechanical properties. BPs have both positive and negative effects on bone's organic matrix by altering both collagen maturity and cross-linking. An increased PYD/DPD ratio is associated with increased strength and stiffness of bone.[9] The PYD/DPD ratio was increased significantly, along with mechanical strength, in vertebral cancellous bone and tibial cortical bone from dogs treated with BPs for 1 year, compared with untreated controls.[6,10] On the other hand, reducing bone turnover also increases pentosidine levels, which are markers for advanced glycation end products (AGEs). AGEs are associated with tissue that is more brittle.[10] Accumulation of pentosidine in bone has been reported to reduce post-yield deformation[9,11] and toughness.[6,12] Tissue from both vertebral[13] and tibial[10] bone from BP-treated animals was less tough than bone from animals not treated with BPs.
There are few data on collagen cross-links in humans treated with BPs, whereas Fourier-transform infrared spectroscopy (FTIR) data show that BP treatment prevented the maturation of collagen and reduced collagen maturity in newly formed bone.[14] Another report[15] reported no change in collagen maturity in women treated with alendronate.[16]
Any agent that suppresses angiogenesis may inhibit the repair of an impending fatigue fracture. The effects of BPs on the repair of fatigue fracture could be worsened if BPs are antiangiogenic.[17] Several studies in nonskeletal tissues have shown that BPs reduce angiogenesis.[18] While direct suppression of angiogenesis by BPs was reported previously,[19] it is difficult to make a distinction between the inhibition of new vessel growth and the suppression of osteoclastic activity because these two phenomena are usually coupled to each other. However, studies of growing animals during skeletal development demonstrated no apparent antiangiogenic effect of clodronate.[20]
Animal studies show that treatment with BPs is associated with reduced bone toughness.[21,22,23] Following 1-3 years of BP treatment at doses similar to or greater than those used in postmenopausal women, toughness was 20-30% lower than in control animals.[21,22] Toughness continues to decline in animals with long-term BP treatment without an increase in microdamage accumulation or a further increase in secondary mineralization.[13] After feeding using various doses of alendronate or risedronate for 1 year, there was minimal correspondence between changes in microdamage accumulation and material-level toughness in vertebrae from several groups of BP-treated dogs.[21] Animals not treated with BPs show an age-related three-fold increase in microdamage accumulation without any apparent change in bone toughness.[13] Neither microdamage nor increased secondary mineralization is solely responsible for the change in bone material properties with BP therapy, leaving changes in collagen or interactions among all these properties as likely reasons for the progressive decline in toughness.[1,3]
Decreased remodeling is also not solely responsible for reduced toughness, indicating a specific effect of BPs that is independent of reduced turnover. The mechanical effect of BPs in decreasing tissue toughness is countered by their capacity to increase bone mass and mineralization, promote collagen matrix maturation, and prevent microarchitectural deterioration of bone.[3] These factors lead to increases in bone strength and stiffness that offset reduced toughness and make bone stronger at the structural level. When FTIR was used to compare the physical properties of cortical and cancellous bone of the proximal femur, there was no difference in mineralization, crystallinity, or collagen maturity between 19 BP-naïve typical femoral fracture patients and 13 BP-positive femoral fractures patients who had taken BP for an average of 7 years. Those who had taken BPs had significantly more homogenous crystallinity and collagen maturity. Greater uniformity of tissue composition was found in those treated with BP. When material stiffness and toughness were measured by micro-indentation techniques, long-term BP users who did not have fracture did not show significantly deteriorated properties compared with untreated controls. Furthermore, patients with AFF who had been treated with BP for 5.5 years did not have worse properties compared with typical fractures. These results suggest that there is not sufficient evidence that the use of BPs adversely affects the material properties of bone.[3]
When a complete fracture occurs and is not fixed rigidly, endochondral ossification is the main healing mechanism of the fracture. An initial inflammatory response is followed by the formation of a chondroid callus. Remodeling that includes resorption of the chondroid callus and replacement with lamellar bone then continues over a long period. Osteoclasts play an important role during the remodeling phase. BPs do not impair the inflammatory process or the development of a proliferative callus.[24,25] BPs interfere with the remodeling phase, delaying the remodeling of the calcified cartilage callus to mature bone. Bone remodeling is important in the healing of fatigue fractures, and is reduced by BP treatment (Fig. 2).
Animal studies with BP treatment showed that prior alendronate eliminated the adaptive remodeling response, suggesting that BP treatment impaired the healing response to a fatigue fracture.[26] In a process of developing fatigue fracture, reduction of bone remodeling would prevent or delay the healing of the stress reaction without suppressing the appearance of a periosteal callus and eventually result in a complete fracture of the fatigued site.
BPs increase bone strength and decrease fracture risk by suppressing excessive bone remodeling. On the other hand, the reduction of remodeling increases microdamage accumulation because microcracks are not removed efficiently. Even in the absence of BP treatment, age-related reductions in bone turnover result in microdamage accumulation.[13] Damage accumulates significantly in humans after the age of 70 years,[27,28] although there is broad individual variability in the amount.
Small decrease in turnover may induce significant accumulation of microdamage. Animal studies showed that reducing trabecular bone activation frequency by ~40% with risedronate caused a 3-fold increase in microdamage, compared with untreated controls, in the canine vertebra.[21] Suppression by ~20% with raloxifene caused a doubling of microdamage.[29]
There are conflicting data on whether microdamage accumulates with BP treatment in humans. Women treated for an average of 5 years with alendronate showed significant microcrack accumulation in a subsample.[30] Another study did not find any association between BP treatment and damage accumulation in the iliac crest.[31] It is difficult to confirm whether damage accumulates in the cortex of the femoral diaphysis because neither study evaluated samples from the femoral cortex, and the accumulation of microdamage is site-specific.
The histological appearance was investigated from iliac crest biopsies and biopsies from fracture sites of patients who had been treated with BPs. While bone turnover was suppressed, normal osteoblastic bone formation was not suppressed. BPs do not suppress the formation of the initial callus or affect the formation of woven bone. However, incomplete repair of fractures occurs by the normal coupled bone remodeling processes. BP localizes on the site of high turnover, because those sites are associated with increased blood flow. With BP suppressing remodeling, intracortical repair of developing fatigue fractures is hampered and the microcracks can grow to a critical size.[3]
The geometry of the proximal femur partly determines the stress generated on the lateral aspect of the femoral cortex.[32] The site of AFF along the diaphysis is highly correlated with the deviation between the anatomical axis (tibiofemoral angle) and the mechanical axis of the lower limb.[33] Patients with more diaphyseal AFF had larger tibiofemoral angles than those who fractured closer to the lesser trochanter. In a Japanese population, patients who had AFF had significantly greater curvature of the femoral diaphysis than age- and gender matched controls.[33]
Assessment of the benefits and risks before BP treatment is essential to avoid unnecessary complications, such as AFF. Patients at low risk of osteoporosis-related fractures do not need medical treatment. For patients with osteoporosis in the spine plus normal or only moderately reduced bone mineral density (BMD) in proximal femur, alternative treatments for osteoporosis, such as raloxifene or teriparatide, is indicated depending on the severity of the patient's condition.[3]
BP therapy is strongly indicated to protect patients from rapid bone loss and increased fracture rates associated with organ transplantation, endocrine disorders, or chemotherapy for breast or prostate cancer, and when aromatase inhibitors and glucocorticoids are first used. Even in these clinical conditions, long-term BP therapy is not always necessary.[34,35]
The optimal duration of BP treatment is still unclear. While studies with alendronate[36] and risedronate[37,38] shows that patients with osteoporosis will have an antifracture benefit for at least 5 years, continued use of BP therapy over 5 years need annual re-evaluation, assessing factors such as BMD, fracture history, newly diagnosed disorders, and other medications known to affect skeletal status, as well as new research findings.
For patients with moderately elevated fracture risk, continuation of BP therapy should be strongly considered. Recent or multiple fractures suggest assessment for underlying secondary causes and reevaluation of the treatment plan. As these patients are known to be at high risk of future fracture, discontinuation of BP treatment is not advised. It is not certain whether BP treatment beyond 5 years will reduce the risk. The incidence of clinical (not morphometric) vertebral fractures was significantly lower in those on 10 years of continued alendronate versus those who stopped after 5 years [36] while a reduction in nonvertebral fracture incidence was limited to women without a fracture history but with femoral neck T scores that were 2.5 or less.[39] With risedronate, 7 years of therapy did not further reduce the incidence of vertebral fractures, compared with 3 and 5 years of treatment.[38]
Taking into account the fact that the median BP treatment duration in patients with AFF is 7 years, for patients without a recent fracture and with femoral neck T-scores greater than 2.5 after the initial therapeutic course, consideration may be given to a "drug holiday'' from BPs. Continued BP therapy should be reevaluated, particularly in those deemed to be at low or only modestly elevated fracture risk.[1,3]
Whether abandoning BP treatment after 4-5 years in the lower-risk group will lead to fewer atypical subtrochanteric fractures is uncertain. Restarting osteoporosis therapy, with BPs or with a different class of agent, can be considered in patients who appear to be at increasing fracture risk. As there can be no general rule on "BP holidays", decisions to stop and/or restart therapy must be individualized (Fig. 3).[1,3]
More than half of the patients who suffer AFF have a prodromal thigh or groin pain before frank fracture develops. Thus, it is necessary to educate physicians and patients about this symptom. Physicians should ask patients on BPs and other potent anti-resorptive agents about thigh or groin pain.[1,3]
When cortical thickenings suggestive of fatigue fractures are found in a patient, magnetic resonance imaging (MRI) or computed tomography (CT) is necessary to further investigate the nature of the lesion. MRI detects a cortical fracture line and associated bone marrow edema or hyperemia, which indicates a fatigue fracture and/or associated new bone formation. If cortical lucency is detected from CT or MRI, then the lesion should likely be considered an incomplete AFF. If a stress reaction is detected, BP should be discontinued. Adequate calcium and vitamin D supplementation is recommended. If pain accompanies the lesion, intramedullary nailing is usually necessary. If there is minimal pain, the patient can be observed with limited weight-bearing through use of crutches or a walker for 2-3 months. If symptomatic and radiographic improvement is not achieved after the period, prophylactic nailing should be considered. If periosteal thickening is observed without associated radiolucency, limited weight-bearing may be continued for another 3 months and reassessed (Fig. 4) [1,3].
Teriparatide has been used increasingly for AFFs because of its bone-forming properties. Although there has not been a randomized, placebo-controlled trial to prove its efficacy, teriparatide can be considered for these fractures that do not appear to heal with conservative treatment. Teriparatide could also be used when there is little evidence of healing by 4-6 weeks after surgical intervention.[1,3]
Figures and Tables
Fig. 1
Sixty-eight-year-old woman with left hip pain. In initial radiograph (left side), there is cortical thickening of lateral cortex of left femur subtrochanter area. One day after (right side), occurred left femoral fracture with no trauma. (Provide by Seung-Jae Lim, MD, Samsung Medical Center).
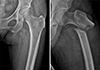
Table 1
The American Society for Bone and Mineral Research task force 2013 revised case definition of atypical femoral fractures
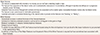
Excludes : fractures of the femoral neck, intertrochanteric fractures with spiral subtrochanteric extension, periprosthetic fractures, and pathological fractures associated with primary or metastatic bone tumors and miscellaneous bone diseases (eg, Paget's disease, fibrous dysplasia).
AFF, atypical femoral fracture.
References
1. Shane E, Burr D, Ebeling PR, et al. Atypical subtrochanteric and diaphyseal femoral fractures: report of a task force of the American Society for Bone and Mineral Research. J Bone Miner Res. 2010; 25:2267–2294.


2. Odvina CV, Zerwekh JE, Rao DS, et al. Severely suppressed bone turnover: a potential complication of alendronate therapy. J Clin Endocrinol Metab. 2005; 90:1294–1301.


3. Shane E, Burr D, Abrahamsen B, et al. Atypical subtrochanteric and diaphyseal femoral fractures: second report of a task force of the American Society for Bone and Mineral Research. J Bone Miner Res. 2014; 29:1–23.


4. Schilcher J, Koeppen V, Ranstam J, et al. Atypical femoral fractures are a separate entity, characterized by highly specific radiographic features. A comparison of 59 cases and 218 controls. Bone. 2013; 52:389–392.


5. Feldstein AC, Black D, Perrin N, et al. Incidence and demography of femur fractures with and without atypical features. J Bone Miner Res. 2012; 27:977–986.


6. Allen MR, Gineyts E, Leeming DJ, et al. Bisphosphonates alter trabecular bone collagen cross-linking and isomerization in beagle dog vertebra. Osteoporos Int. 2008; 19:329–337.


7. Wang X, Shen X, Li X, et al. Age-related changes in the collagen network and toughness of bone. Bone. 2002; 31:1–7.


8. Zioupos P, Currey JD, Hamer AJ. The role of collagen in the declining mechanical properties of aging human cortical bone. J Biomed Mater Res. 1999; 45:108–116.


9. Tang SY, Zeenath U, Vashishth D. Effects of non-enzymatic glycation on cancellous bone fragility. Bone. 2007; 40:1144–1151.


10. Tang SY, Allen MR, Phipps R, et al. Changes in non-enzymatic glycation and its association with altered mechanical properties following 1-year treatment with risedronate or alendronate. Osteoporos Int. 2009; 20:887–894.


11. Vashishth D, Gibson GJ, Khoury JI, et al. Influence of nonenzymatic glycation on biomechanical properties of cortical bone. Bone. 2001; 28:195–201.


12. Viguet-Carrin S, Roux JP, Arlot ME, et al. Contribution of the advanced glycation end product pentosidine and of maturation of type I collagen to compressive biomechanical properties of human lumbar vertebrae. Bone. 2006; 39:1073–1079.


13. Allen MR, Burr DB. Three years of alendronate treatment results in similar levels of vertebral microdamage as after one year of treatment. J Bone Miner Res. 2007; 22:1759–1765.


14. Durchschlag E, Paschalis EP, Zoehrer R, et al. Bone material properties in trabecular bone from human iliac crest biopsies after 3- and 5-year treatment with risedronate. J Bone Miner Res. 2006; 21:1581–1590.


15. Boskey AL, Spevak L, Weinstein RS. Spectroscopic markers of bone quality in alendronate-treated postmenopausal women. Osteoporos Int. 2009; 20:793–800.


16. Donnelly E, Meredith DS, Nguyen JT, et al. Reduced cortical bone compositional heterogeneity with bisphosphonate treatment in postmenopausal women with intertrochanteric and subtrochanteric fractures. J Bone Miner Res. 2012; 27:672–678.


17. Allen MR, Burr DB. Human femoral neck has less cellular periosteum, and more mineralized periosteum, than femoral diaphyseal bone. Bone. 2005; 36:311–316.


18. Fournier P, Boissier S, Filleur S, et al. Bisphosphonates inhibit angiogenesis in vitro and testosterone-stimulated vascular regrowth in the ventral prostate in castrated rats. Cancer Res. 2002; 62:6538–6544.
19. Wood J, Bonjean K, Ruetz S, et al. Novel antiangiogenic effects of the bisphosphonate compound zoledronic acid. J Pharmacol Exp Ther. 2002; 302:1055–1061.


20. Deckers MM, Van Beek ER, Van Der Pluijm G, et al. Dissociation of angiogenesis and osteoclastogenesis during endochondral bone formation in neonatal mice. J Bone Miner Res. 2002; 17:998–1007.


21. Allen MR, Iwata K, Phipps R, et al. Alterations in canine vertebral bone turnover, microdamage accumulation, and biomechanical properties following 1-year treatment with clinical treatment doses of risedronate or alendronate. Bone. 2006; 39:872–879.


22. Allen MR, Reinwald S, Burr DB. Alendronate reduces bone toughness of ribs without significantly increasing microdamage accumulation in dogs following 3 years of daily treatment. Calcif Tissue Int. 2008; 82:354–360.


23. Mashiba T, Turner CH, Hirano T, et al. Effects of suppressed bone turnover by bisphosphonates on microdamage accumulation and biomechanical properties in clinically relevant skeletal sites in beagles. Bone. 2001; 28:524–531.


24. Cao Y, Mori S, Mashiba T, et al. Raloxifene, estrogen, and alendronate affect the processes of fracture repair differently in ovariectomized rats. J Bone Miner Res. 2002; 17:2237–2246.


25. Martinez MD, Schmid GJ, McKenzie JA, et al. Healing of non-displaced fractures produced by fatigue loading of the mouse ulna. Bone. 2010; 46:1604–1612.


26. Barrett JG, Sample SJ, McCarthy J, et al. Effect of short-term treatment with alendronate on ulnar bone adaptation to cyclic fatigue loading in rats. J Orthop Res. 2007; 25:1070–1077.


27. Mori S, Harruff R, Ambrosius W, et al. Trabecular bone volume and microdamage accumulation in the femoral heads of women with and without femoral neck fractures. Bone. 1997; 21:521–526.


28. Schaffler MB, Choi K, Milgrom C. Aging and matrix microdamage accumulation in human compact bone. Bone. 1995; 17:521–525.


29. Allen MR, Iwata K, Sato M, et al. Raloxifene enhances vertebral mechanical properties independent of bone density. Bone. 2006; 39:1130–1135.


30. Stepan JJ, Burr DB, Pavo I, et al. Low bone mineral density is associated with bone microdamage accumulation in postmenopausal women with osteoporosis. Bone. 2007; 41:378–385.


31. Chapurlat RD, Arlot M, Burt-Pichat B, et al. Microcrack frequency and bone remodeling in postmenopausal osteoporotic women on long-term bisphosphonates: a bone biopsy study. J Bone Miner Res. 2007; 22:1502–1509.


32. Crossley K, Bennell KL, Wrigley T, et al. Ground reaction forces, bone characteristics, and tibial stress fracture in male runners. Med Sci Sports Exerc. 1999; 31:1088–1093.


33. Sasaki S, Miyakoshi N, Hongo M, et al. Low-energy diaphyseal femoral fractures associated with bisphosphonate use and severe curved femur: a case series. J Bone Miner Metab. 2012; 30:561–567.


34. Cohen A, Addesso V, McMahon DJ, et al. Discontinuing antiresorptive therapy one year after cardiac transplantation: effect on bone density and bone turnover. Transplantation. 2006; 81:686–691.


35. Hershman DL, McMahon DJ, Crew KD, et al. Prevention of bone loss by zoledronic acid in premenopausal women undergoing adjuvant chemotherapy persist up to one year following discontinuing treatment. J Clin Endocrinol Metab. 2010; 95:559–566.


36. Black DM, Schwartz AV, Ensrud KE, et al. Effects of continuing or stopping alendronate after 5 years of treatment: the Fracture Intervention Trial Long-term Extension (FLEX): a randomized trial. JAMA. 2006; 296:2927–2938.


37. Mellström DD, Sörensen OH, Goemaere S, et al. Seven years of treatment with risedronate in women with postmenopausal osteoporosis. Calcif Tissue Int. 2004; 75:462–468.

