Abstract
Objectives
Materials and Methods
Results
Figures and Tables
Figure 1
Morphology of HDPCs isolated by three different methods. (a) Image of HDPCs-OG. Cells were homogenous with a typical fibroblastic shape; (b) Image of HDPCs-ED. Cells were more heterogeneous in shape, mstly fibroblast-like, although some appeared cuboidal or polygonal; (c) HDPCs-Combined were mixed phenotype with HDPCs-OG and HDPCs-ED. Scale bar: 50 µm. HDPCs, human dental pulp cells; HDPCs-OG, HDPCs were isolated by the outgrowth method; HDPCs-ED, HDPCs were isolated by the enzymatic digestion method (collagenase/dispase/trypsin); HDPCs-Combined, HDPCs were isolated by the combination of both methods.
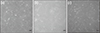
Figure 2
Immunofluorescence analysis of HDPCs. Expression of mesenchymal stem cell (MSC) markers on (a) HDPCs-OG, (b) HDPCs-ED, and (c) HDPCs-Combined, respectively. Each value in the histogram represents an average % mean positivity of the specific marker above the isotype control from three independent experiments. HDPCs, human dental pulp cells; HDPCs-OG, HDPCs were isolated by the outgrowth method; HDPCs-ED, HDPCs were isolated by the enzymatic digestion method (collagenase/dispase/trypsin); HDPCs-Combined, HDPCs were isolated by the combination of both methods.
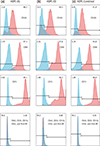
Figure 3
Osteogenic differentiation of HDPCs isolated by three different methods. Alizarin Red S staining of HDPCs-OG (a - d), HDPCs-ED (e - h), and HDPCs-Combined (i - l) cultures at 14 and 28 days after the induction of differentiation. Mineralization progressed at a higher rate in HDPC-ED cultures at 14 days (f) and 28 days (h) after induction than HDPCs-OG (b and d) and HDPCs-Combined (j and l). Scale bar: 50 µm. HDPCs, human dental pulp cells; HDPCs-OG, HDPCs were isolated by the outgrowth method; HDPCs-ED, HDPCs were isolated by the enzymatic digestion method (collagenase/dispase/trypsin); HDPCs-Combined, HDPCs were isolated by the combination of both methods. *Asterisk indicates difference significant between control and treated groups (α = 0.05).
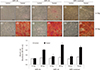
Figure 4
Adipogenic differentiation of HDPCs isolated by three different methods. Oil Red O staining of HDPCs-OG (a - d), HDPCs-ED (e - h), and HDPCs-Combined (i - l) cultures at 14 and 28 days after the induction of differentiation. Accumulated lipid droplets in HDPCs-OG (d), HDPCs-ED (h), and HDPCs-Combined (l) at 28 days after induction. Scale bar: 50 µm. HDPCs, human dental pulp cells; HDPCs-OG, HDPCs were isolated by the outgrowth method; HDPCs-ED, HDPCs were isolated by the enzymatic digestion method (collagenase/dispase/trypsin); HDPCs-Combined, HDPCs were isolated by the combination of both methods. *Asterisk indicates difference significant between control and treated groups (α = 0.05).
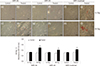
Figure 5
Chondrogenic differentiation of HDPCs isolated by three different methods. Alcian Blue staining of HDPCs-OG (a - d), HDPCs-ED (e - h) and HDPCs-Combined (i - l) at 14 and 28 days after the induction of differentiation. Scale bar: 50 µm. HDPCs, human dental pulp cells; HDPCs-OG, HDPCs were isolated by the outgrowth method; HDPCs-ED, HDPCs were isolated by the enzymatic digestion method (collagenase/dispase/trypsin); HDPCs-Combined, HDPCs were isolated by the combination of both methods.
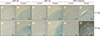
Figure 6
Expression of differentiation markers in induced HDPCs by real-time RT-PCR. HDPCs were induced with (a) osteogenic, (b) adipogenic, or (c) chondrogenic factors for 28 days and the expression levels of typical differentiation markers for each lineage were examined by RT-PCR. Data are presented as means ± standard deviations (n = 10, p < 0.05)*. HDPCs, human dental pulp cells; RT-PCR, real-time quantitative reverse-transcription polymerase chain reaction; OG, HDPCs-OG group; ED, HDPCs-ED group; Combined, HDPCs-Combined group; C, control group which were maintained in medium without inducers; T, treated group which were induced to undergo adipogenic, osteogenic, or chondrogenic differentiation using an MSC functional identification kit; HDPCs-OG, HDPCs were isolated by the outgrowth method; HDPCs-ED, HDPCs were isolated by the enzymatic digestion method (collagenase/dispase/trypsin); HDPCs-Combined, HDPCs were isolated by the combination of both methods; ALP, alkaline phosphatase; BSP2, bone sialoprotein2; COL1A1, collagen type-I alpha-1; OCN, osteocalcin; ON, osteonectin; OPN, osteopontin; OSX, osterix; RUNX2, runt-related transcription factor 2; LPL, lipoprotein lipase; PPAR-γ, peroxisome proliferator-activated receptor-gamma; ACAN, aggrecan; COL2A1, collagen type-II alpha-1; COL10A1, collagen type-X; and SOX9, sex-determining region Y-box 9.
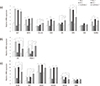
Figure 7
Expression of differentiation markers in induced HDPCs by western blotting. Representative images were shown of HDPCs which were induced with (a) osteogenic, (b) adipogenic, or (c) chondrogenic factors for 28 days and the expression levels of typical differentiation markers for each lineage were examined by western blotting. HDPCs, human dental pulp cells; OG, HDPCs-OG group; ED, HDPCs-ED group; Combined, HDPCs-Combined group; C, control group which were maintained in medium without inducers; T, treated group which were induced to undergo adipogenic, osteogenic, or chondrogenic differentiation using an MSC functional identification kit. HDPCs-OG, HDPCs were isolated by the outgrowth method; HDPCs-ED, HDPCs were isolated by the enzymatic digestion method (collagenase/dispase/trypsin); HDPCs-Combined, HDPCs were isolated by the combination of both methods; ALP, alkaline phosphatase; BSP2, bone sialoprotein2; COL1A1, collagen type-I alpha-1; OCN, osteocalcin; ON, osteonectin; OPN, osteopontin; OSX, osterix; RUNX2, runt-related transcription factor 2; LPL, lipoprotein lipase; PPAR-γ, peroxisome proliferator-activated receptor-gamma; ACAN, aggrecan; COL2A1, collagen type-II alpha-1; COL10A1, collagen type-X; and SOX9, sex-determining region Y-box 9.
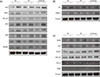
Table 1
Differentiation marker genes for identification of Mesenchymal Stem Cells
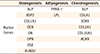
Osteogenesis | Adipogenesis | Chondrogenesis | |
---|---|---|---|
Marker Genes | ALP | PPAR-γ | ALP |
BSP2 | LPL | COLA1 | |
COL1A1 | SOX9 | ||
OCN | COL2A1 | ||
ON | COL10A1 | ||
OPN | ACAN | ||
OSX | |||
RUNX2 |
ACAN, aggrecan; ALP, alkaline phosphatase; BSP2, bone sialoprotein 2; COL1A1, collagen type-I alpha-1; COL2A1, collagen type-II alpha-1; COL10A1, collagen type-X; LPL, lipoprotein lipase; OCN, osteocalcin; ON, osteonectin; OSX, osterix; OPN, osteopontin; PPAR-γ, peroxisome proliferator-activated receptor-gamma; RUNX2, runt-related transcription factor 2; SOX9, sex determining region Y-box 9.
Acknowledgment
References
































