Abstract
Objectives
The aim of this study was to investigate the expression of 7 different sirtuin genes (SIRT1-SIRT7) in human dental pulp cells (HDPCs), and to determine the role of SIRTs in the odontoblastic differentiation potential of HDPCs.
Materials and Methods
HDPCs were isolated from freshly extracted third molar teeth of healthy patients and cultulred in odontoblastic differentiation inducing media. Osteocalcin (OCN) and dentin sialophosphoprotein (DSPP) expression was analyzed to evaluate the odontoblastic differentiation of HDPCs by reverse transcription-polymerase chain reaction (RT-PCR), while alizarin red staining was used for the mineralization assay. To investigate the expression of SIRTs during odontoblastic differentiation of HDPCs, real time PCR was also performed with RT-PCR.
Results
During the culture of HDPCs in the differentiation inducing media, OCN, and DSPP mRNA expressions were increased. Mineralized nodule formation was also increased in the 14 days culture. All seven SIRT genes were expressed during the odontogenic induction period. SIRT4 expression was increased in a time-dependent manner.
The dental pulp is a very dynamic tissue that responds to dental injury and irreversible carious damage. In response to milder stimuli, the surviving odontoblasts contribute to the formation of tertiary dentin. On the other hand, odontoblasts are disrupted as a result of extensive damage in aggressive lesion, and replaced with newly-differentiated odontoblast-like cells derived from the dental pulp. These newly-differentiated dental pulp cells secrete a reparative dentin matrix.1 The intercellular mechanisms controlling the odontoblast differentiation process are still under investigation.
Sirtuins (SIRTs) are the members of the SIR2 (silent information regulator 2) family, and are NAD+-dependent deacetylases.2 Seven isoforms of SIRT proteins have so far been identified in humans. SIRT1 and SIRT2 reside in the nucleus and cytoplasm. SIRT3, SIRT4, and SIRT5 exist in the mitochondria, while SIRT6 and SIRT7 are found in the nucleus.3 SIRT1 plays a critical role in cellular functions ranging from cell cycle regulation, reduction of apoptosis, inhibition of inflammation, and differentiation.456 SIRT1, SIRT3, and SIRT6 are implicated as anti-aging molecules, and their over-expression in both adipose and liver tissue contributes to the development of metabolic inflammation after weight loss.78 A recent study has suggested that overexpression of SIRT2 in lung cancer cell lines regulated cell proliferation, apoptosis, and cell cycle.9
Previous studies reported the effect of SIRT1 and SIRT6 on the odontoblastic differentiation potential of human dental pulp cells (HDPCs), and suggested their potential therapeutic effect in pulp-dentin regeneration.610 Kim et al. reported that over-expression of SIRT1 increased mineralized nodule formation, and upregulated the levels of odontoblastic markers, such as alkaline phosphatase (ALP), osteocalcin (OCN), dentin matrix protein-1, and dentin sialophosphoprotein (DSPP) as well as angiogenic markers, such as vascular endothelial cadherin and platelet endothelial cell adhesion molecule 1, and fibroblast growth factor-2, while inhibition of SIRT1 decreased the expression of those genes.6 Down-regulation of the SIRT6 gene decreased the mineralization effect in HDPCs, and over-expression of SIRT6 increased the mineralization effects such as ALP activity, calcium nodule formation and odontoblast differentiation marker genes.10
However, there are no study of the effect of all 7 isoforms of SIRTs on the odontoblastic differentiation potential of HDPCs. Therefore, the purposes of this study were to investigate the expression 7 different SIRT genes (SIRT1-SIRT7) in HDPCs, and to determine their role during the odontoblastic differentiation of HDPCs.
HDPCs were isolated from freshly extracted third molar teeth of three young male patients. All procedures were conducted after obtaining informed consent. Experimental protocol was approved by the Institutional Review Board of the Chonnam National University Dental Hospital (CNUDH-2013-002). The teeth were kept in phosphate-buffered saline (PBS) immediately after extraction. They were split accessed under the sterile condition to harvest pulp tissues. Obtained pulp tissues were minced with a surgical knife and placed in 6-well cell culture plates containing α-minimum essential medium (α-MEM, Gibco Invitrogen, Grand Island, NY, USA) supplemented with 10% fetal bovine serum (Gibco Invitrogen) and antibiotics (100 U/mL penicillin and 100 mg/mL streptomycin, Gibco Invitrogen) in a humidified atmosphere of 5% CO2 at 37℃. Cell passage numbers from 3 to 4 were used for the following experiment. To induce odontoblastic differentiation, the cells were treated with 50 µg/mL ascorbic acid (Sigma-Aldrich, St Louis, MO, USA), 10 mmol/L β-glycerophosphate (Santa Cruz Biotechnology, Inc., Dallas, TX, USA), and 100 nmol/L dexamethasone (Sigma-Aldrich) from day 0 to 14.
Cells grown in differentiation-inducing media were harvested at 0, 1, 3, 7, and 14 days. Total RNA was isolated with the TRIzol reagent (Invitrogen, Carlsbad, CA, USA) according to the manufacturer's instructions. cDNA was synthesized using Maxime RT PreMix Kit (iNtRON Biotech, Seongnam, Korea). The cDNAs of the target gene were amplified with specific primers as shown in Table 1, using Go-Taq Green Master Mix (Promega, Madison, WI, USA). Initial denaturation (94℃ for 10 minutes) was followed by 40 cycles of PCR (94℃, 30 seconds, denaturation; 55℃, 30 seconds, annealing; 72℃, 30 seconds, extension) and additional incubation for 7 minutes at 72℃. Equal amounts of reverse transcription-polymerase chain reaction (RT-PCR) products were loaded into each well, run through 1.5% agarose gel, and visualized by ethidium bromide staining. RT-PCR results were quantified using image J (Version 1.47, National Institutes of Health, Bethesda, MD, USA). The band density of each gene was normalized by the density of β-actin as a control.
Real-time PCR and data analysis were performed according to the instructions of the MiniOpticon Real-Time PCR system (Bio-Rad Co., Hercules, CA, USA). Duplicate samples were used for data accuracy. Total volume (25 mL) included SYBR Green TOPreal qPCR 2x PreMix (Enzynomics, Daejeon, Korea), cDNA, primers (10 pmol of each primer), and sterile water. Forty thermo cycles of 95℃, 15 seconds, 58℃, 30 seconds, and 72℃, 30 seconds were run after the denaturation of DNA at 95℃ for 10 minutes. The melting curve analysis was conducted in 0.2 seconds interval from 65℃ to 95℃. Primer sequences used were shown in Table 1. All quantified values were normalized to an endogenous control β-actin. The data for gene expression were analyzed by the ΔΔCt method.
For mineralized nodule formation assay, HDPCs were seeded in 24 well plates at a density of 1 × 105 cells. After 14 days, the samples were washed with PBS and fixed with 70% ice-cold ethanol for 1 hour at room temperature. They were rinsed twice with distilled water, and stained with 2% alizarin red stain solution (LIFELINE Cell Tech, Frederick, MD, USA) for 20 minutes and washed 5 times with sterile water. Alizarin red staining was photographed using an Officejet pro L7580 scanner (HP, Palo Alto, CA, USA) and staining density was quantified using Image J (National Institutes of Health).
All experiments were performed in triplicate. Differences between groups were evaluated by analysis of variance (ANOVA), and the Duncan test was used for post hoc analysis with the SPSS 17.0 software program (SPSS, Chicago, IL, USA). A p value of < 0.05 was considered statistically significant.
We assessed the level of mRNA expression of odontoblastic differentiation markers such as OCN and DSPP in induced HDPCs. As shown in Figure 1, there was a time-dependent increase in the mRNA expression levels of the odontogenic differentiation marker genes during 14 days of cultivation.
To investigate the mineralization potential of differentiated HDPCs, we examined mineralized nodule formation by using alizarin red staining after 0, 7 and 14 days. Alizarin red staining for calcium nodule formation showed a gradual increase with passage of time (Figure 2).
RT-PCR showed that all seven SIRT genes were expressed in HDPCs during the odontogenic differentiation induction period. Among the SIRT genes, SIRT1 was strongly expressed in early time and decreased thereafter. SIRT4 expression was increased during the course of culture period. SIRT6 was strongly expressed at day 7, but the expression was decreased at day 14 (Figure 3). To evaluate the quantitative expression of SIRT4, real-time RT-PCR was performed. The mRNA expression level of SIRT4 was significantly increased in a time dependent manner during the course of culture period (Figure 4).
The purpose of the current study was to assess the effect of SIRTs on odontoblastic differentiation of HDPCs. The primary HDPCs were cultured in differentiation inducing media, and the mRNA expressions of OCN and DSPP were assessed as odontoblastic differentiation markers.11 As a result, we observed up-regulation of both markers in a time-dependent manner, which confirmed the odontogenic differentiation of HDPCs induced by differentiation inducing media. In addition, we noticed the formation of calcified nodules in HDPCs, indicating the mineralization ability of differentiated HDPCs.
To determine the mRNA expression levels of SIRT1 - 7 in HDPCs, we performed RT-PCR at 14 days of cultivation. Among the SIRT genes, the level of SIRT4 was significantly increased during culture periods. On the contrary, the SIRT1 gene was strongly expressed early time and thereafter decreased in a time-dependent manner. Previous studies reported that SIRT1 plays an important role in the odontoblastic differentiation potential.6 Kim et al. demonstrated that SIRT1 overexpression increased mineralization nodules and up-regulated the mRNA level of odontoblastic markers, while inhibition of the SIRT1 gene decreased odontoblastic differentiation and down-regulated angiogenic factors in HDPCs.6 They reported that SIRT1 mRNA and protein levels steadily decreased in differentiating HDPCs, which is similar to that in a study on periodontal ligament cells.56 Lee et al. studied the role of SIRT1 in osteoblastic differentiation in human periodontal ligament cells, and found that the mRNA and protein levels of SIRT1 increased until 3 days in odontogenic medium, and then decreased during 14 days.5 Our results are consistent with these previous studies, indicating that the level of SIRT1 protein increased, exerted an influence on the early stage of odontoblastic differentiation process, and then steadily declined.
There is no report on the effect of SIRT4 on the odontogenic differentiation potential of HDPCs. In the present study, the level of SIRT4 was significantly increased as the HDPCs underwent differentiation. Compared to SIRT1, we could infer that SIRT4 may act in the late stage of the differentiation process. SIRT1 is a nuclear sirtuin, and SIRT4 is a mitochondrial sirtuin.12 SIRT1 deacetylates specific transcription factors and histones to influence gene expression.13 SIRT4 regulates the activities of metabolic enzymes and plays important roles in apoptosis and intracellular signaling.14 Interestingly, Nasrin et al. demonstrated an increase in SIRT1 mRNA and protein levels in response to the inhibition of SIRT4 gene, which means mitochondrial SIRT4 shows opposite action to SIRT1.15
Besides SIRT1 and SIRT4, SIRT6 is also essential for odontoblast differentiation and mineralization ability of HDPCs.10 In the study by Sun et al. the endogenous level of SIRT6 in induction media was significantly up-regulated at day 2, and decreased at days 4 and 7.10 They reported that this indicates that SIRT6 may influence odontoblastic differentiation of HDPCs.10 Inhibition of the SIRT6 gene impairs odontoblastic differentiation, and over-expression of SIRT6 promotes the mineralization ability of HDPCs.10 In our study, SIRT6 was strongly expressed at day 7, but the expression was decreased at day 14, which indicates that SIRT6 exerts an influence during the relatively early stage of the differentiation process, and then decrease in a time-dependent manner.
This study identified the expression of seven different SIRTs in during the odontoblastic differentiation of HDPCs, and revealed that SIRT4 could exert an influence at the late stage of the process. As the expression of SIRT4 was increased, the expression of SIRT1 was decreased. Additional experiments are needed to identify the relationship among SIRT1, SIRT4, and SIRT6. Further studies are also needed to determine the effects of other SIRTs on the odontogenic differentiation potential of HDPCs.
Figures and Tables
Figure 1
Expression of OCN and DSPP during the odontogenic differentiation of HDPCs analyzed by RT-PCR. OCN, osteocalcin; DSPP, dentin sialophosphoprotein; HDPC, human dental pulp cell; RT-PCR, reverse transcription-polymerase chain reaction.
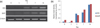
Figure 2
Mineralization during the odontogenic differentiation of HDPCs analyzed by alizarin red staining. HDPC, human dental pulp cell; GM, growth media; OIM, odontogenic induction media.
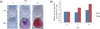
Figure 3
Expression of SIRT genes during the odontogenic differentiation of HDPCs analyzed by RT-PCR. SIRT, sirtuin; HDPC, human dental pulp cell; RT-PCR, reverse transcription-polymerase chain reaction.
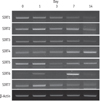
Figure 4
Expression level of SIRT4 mRNA during the odontogenic differentiation of HDPCs analyzed by realtime PCR. SIRT, sirtuin; mRNA, messenger ribonucleic acid; HDPC, human dental pulp cell.
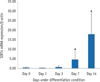
Table 1
Primer sequences for polymerase chain reaction
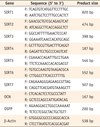
Acknowledgement
This study was supported by the National Research Foundation of Korea (NRF) grant funded by the Korea government (MEST) (No. 2011-0030757).
References
1. Fouad AF, Levin L. Pulpal reactions to caries and dental procedures. In : Hargreaves KM, Cohen S, editors. Pathways of the pulp. 10th ed. St. Louis, MO: Mosby Elsevier;2011. p. 504–528.
2. Imai S, Armstrong CM, Kaeberlein M, Guarente L. Transcriptional silencing and longevity protein Sir2 is an NAD-dependent histone deacetylase. Nature. 2000; 403:795–800.


3. Michishita E, Park JY, Burneskis JM, Barrett JC, Horikawa I. Evolutionarily conserved and nonconserved cellular localizations and functions of human SIRT proteins. Mol Biol Cell. 2005; 16:4623–4635.


4. Haigis MC, Sinclair DA. Mammalian sirtuins: biological insights and disease relevance. Annu Rev Pathol. 2010; 5:253–295.


5. Lee YM, Shin SI, Shin KS, Lee YR, Park BH, Kim EC. The role of sirtuin 1 in osteoblastic differentiation in human periodontal ligament cells. J Periodontal Res. 2011; 46:712–721.


6. Kim JJ, Kim SJ, Kim YS, Kim SY, Park SH, Kim EC. The role of SIRT1 on angiogenic and odontogenic potential in human dental pulp cells. J Endod. 2012; 38:899–906.


7. Kitada M, Kume S, Takeda-Watanabe A, Kanasaki K, Koya D. Sirtuins and renal diseases: relationship with aging and diabetic nephropathy. Clin Sci (Lond). 2013; 124:153–164.


8. Moschen AR, Wieser V, Gerner RR, Bichler A, Enrich B, Moser P, Ebenbichler CF, Kaser S, Tilg H. Adipose tissue and liver expression of SIRT1, 3, and 6 increase after extensive weight loss in morbid obesity. J Hepatol. 2013; 59:1315–1322.


9. Li Z, Xie QR, Chen Z, Lu S, Xia W. Regulation of SIRT2 levels for human non-small cell lung cancer therapy. Lung Cancer. 2013; 82:9–15.


10. Sun HL, Wu YR, Huang C, Wang JW, Fu DJ, Liu YC. The effect of SIRT6 on the odontoblastic potential of human dental pulp cells. J Endod. 2014; 40:393–398.


11. Wei X, Ling J, Wu L, Liu L, Xiao Y. Expression of mineralization markers in dental pulp cells. J Endod. 2007; 33:703–708.


12. Morris BJ. Seven sirtuins for seven deadly diseases of aging. Free Radic Biol Med. 2013; 56:133–171.