Abstract
Objectives
This study determined the effect of the air-stream application time and the bonding technique on the dentin bond strength of adhesives with different solvents. Furthermore, the content and volatilization rate of the solvents contained in the adhesives were also evaluated.
Materials and Methods
Three adhesive systems with different solvents (Stae, SDI, acetone; XP Bond, Dentsply De Trey, butanol; Ambar, FGM, ethanol) were evaluated. The concentrations and evaporation rates of each adhesive were measured using an analytical balance. After acid-etching and rinsing, medium occlusal dentin surfaces of human molars were kept moist (conventional) or were treated with 10% sodium hypochlorite for deproteinization. After applying adhesives over the dentin, slight air-stream was applied for 10, 30 or 60 sec. Composite cylinders were built up and submitted to shear testing. The data were submitted to ANOVA and Tukey's test (α = 0.05).
Results
Stae showed the highest solvent content and Ambar the lowest. Acetone presented the highest evaporation rate, followed by butanol. Shear bond strengths were significantly affected only by the factors of 'adhesive' and 'bonding technique' (p < 0.05), while the factor 'duration of air-stream' was not significant. Deproteinization of dentin increased the bond strength (p < 0.05). Stae showed the lowest bond strength values (p < 0.05), while no significant difference was observed between XP Bond and Ambar.
Even with the evolution of adhesive systems in recent decades, the establishment of a predictable bonding interface for dentin tissue has remained a challenge for clinicians.1,2,3 Although the self-etching adhesive systems have gained popularity, etch-and-rinse adhesives remain as suitable option for adhesive procedures in clinical practice. For etch-and-rinse adhesives, phosphoric acid is applied before the adhesive system, partially removing the mineral content of the dentin tissue to expose the collagen mesh.4,5 Subsequently, the dentin is kept moist after acid removal to prevent the collapse of the collagen mesh and to allow for the penetration of the adhesive resin.5,6 Volatile organic solvents are added to the primer/adhesive to permit its penetration into the moist collagen mesh to produce hybrid layer.7,8,9 These solvents also facilitate water evaporation from the dentin, allowing for adhesive polymerization.8
Proper adhesive polymerization results in improved mechanical properties of the adhesive layer, increasing the bond strength. In addition to water removal from the dentin tissue, the elimination of solvent from the adhesive layer is also an important step for proper polymerization of the adhesive.10,11 Clinically, an air-stream is used to facilitate solvent removal from the adhesive layer. However, solvents presenting different volatilities can also be added to adhesives in concentrations varying from 30% to 80%.12,13 Thus, it is reasonable to assume a positive correlation between the time of air-stream application and the volatility and concentration of solvents.12,13,14,15 However, few studies have evaluated possible correlations between these factors.
Furthermore, alternative techniques have been proposed to reduce the sensitivity of adhesive procedures to the moisture in demineralized dentin and to solvent content. The dentin deproteinization technique was initially developed to reduce the discrepancy between the depths of acid etching performed and subsequent penetration of the adhesive resin.16 Sodium hypochlorite (NaOCl) solution is applied over the demineralized dentin to remove the non-encapsulated collagen, improving the stability of hybrid layer.16 Recently, it was demonstrated that dentin deproteinization could reduce the dependence on the solvent content of the adhesive in bonding procedures.8 Thus, the aims of this in vitro study were to measure the content and volatilization rate of solvents contained in commercial adhesives, and to evaluate the effects of the duration of air-stream application on bond strength to dentin, using a conventional or deproteinization technique. The hypotheses tested were that adhesive containing more volatile solvents would require shorter durations of air-stream application to obtain higher values of bond strength, and the dentin deproteinization would decrease the dependency on solvent removal to reach proper bond strength values.
This investigation was conducted using a 2 × 3 × 3 factorial study design to evaluate the factors of 'bonding technique' at two levels (conventional or deproteinization), 'duration of air-stream' at three levels (10, 30 or 60 seconds) and 'adhesive system' at three levels (Stae, SDI, Bayswater, Victoria, Australia; XP Bond, Dentsply De Trey, Konstanz, Germany; Ambar FGM, Joinville, SC, Brazil). The manufacturers and compositions of the adhesives are described in Table 1. The solvent content of each adhesive and its evaporation rate were evaluated in a precision balance (n = 3). The bond strength of the adhesive applied to water-wet (conventional technique) or deproteinized dentin was evaluated by the shear bond strength test, followed by failure mode analysis (n = 7). This study was approved by the local Research Ethics Committee (protocol #606.376/2014) of Federal University of Sergipe, Brazil.
One drop of each adhesive was dispensed on an analytical balance (JEX-200, YMC Co. Ltd., Kyoto, Japan) to determine the solvent content of the adhesives and the evaporation rate of each solvent. The mass of an adhesive drop was recorded at 5 seconds intervals until it reached equilibrium at room temperature. Equilibrium was established when no significant mass alteration was measured after 1 minute. In this time, the solvent content was determined as the ratio between the final and initial weights, according to the following formula:
To determine the average evaporation rate, the solvent loss (100% solvent content) was divided by the time required to achieve equilibrium (time when the last mass alteration occurred). The solvent content and evaporation rate were measured in triplicate. No air-stream was applied over the adhesive drop during the measurement that was performed under controlled temperature (25℃) and humidity (around 60%) conditions.
One hundred twenty-six non-carious human third molars, stored in 0.05% thymol saline solution at 4℃, were used in this study (Figure 1). Two parallel sections were created using a slow-speed saw (Extec Corp., Enfield, CT, USA) to expose a flat occlusal dentin surface and to remove the roots. The surfaces were inspected with an optical stereomicroscope at ×40 magnification to ensure the absence of enamel sites. The samples were placed in acrylic resin cylinders to facilitate handling, and the dentin surfaces were wet-polished with 600 grit silicon carbide paper for 1 minute to standardize the smear layer.
The dentin was etched with 37% phosphoric acid for 15 seconds, followed by rinsing with water for 10 seconds. Samples were randomly allocated to one of following bonding techniques:
- Conventional: Excess water was removed using absorbent paper, leaving the dentin surface slightly moist.
- Deproteinization: 5% NaOCl (pH = 12) was applied over the dentin. After 1 minute, the dentin surface was rinsed with water for 10 seconds and then dried with an air-stream.
For each bonding technique, one of the adhesives evaluated were applied on the dentin surface using a micro-brush applicator. An air-stream was placed 10 cm from the dentin surface and was applied for 10, 30 or 60 seconds. Subsequently, the adhesives were light-cured for 25 seconds with a light-emitting diode curing unit (Radii Cal, SDI, Bayswater, Victoria, Australia). Elastomer molds with two cylindrical orifices (1.0 mm in diameter × 2.0 mm in thickness) were placed onto the dentin substrate, and the cylinders were filled with Opallis flow resin (FGM, Joinville, SC, Brazil). The composite was light-cured for 25 seconds, the molds were removed, and the samples were stored in distilled water at 37℃ for 24 hours.
For the shear bond strength test, a stainless steel wire (0.2 mm in diameter) was looped around each cylinder and aligned with the bonded interface. The test was conducted on a mechanical testing machine (Microtensile OM100, Odeme Dental Research, Joacaba, SC, Brazil) at a crosshead speed of 0.7 mm/min until failure. Shear bond strength was calculated considering the area of bonding interface and was expressed in MPa. Fractured specimens were observed under magnifications of up to ×500 on an optical microscope to classify the failure mode into Type I, adhesive failure, Type II, mixed failure with more than 50% adhesive, and Type III, mixed failure with less than 50% adhesive.
Data on solvent content and volatilization rate were individually submitted to one-way analysis of variance (ANOVA) and Tukey's post-hoc test. Data on bond strength were analyzed by three-way ANOVA (adhesive × time of air-stream × bonding technique) and Tukey's post-hoc test. The significance level was set to α = 0.05 for all analyses. Data analysis was performed using the SigmaStat statistical software package version 3.5 (Systat Software Inc., Chicago, IL, USA).
ANOVA showed significant effects for adhesive regarding the solvent content (p < 0.001). Stae showed the highest and Ambar the lowest solvent concentrations, while XP Bond presented intermediate values (Figure 2). Regarding the evaporation rate, ANOVA also showed significant effects for adhesive (p < 0.001). The results of the evaporation rates are presented in Figure 3. Stae (acetone) demonstrated the highest evaporation rate among the adhesives evaluated, followed by XP Bond (butanol). Ambar (ethanol) showed the lowest evaporation rate value.
For shear bond strength, three-way ANOVA showed significant effects only for the factors of 'adhesive' (p < 0.001) and 'bonding technique' (p = 0.013), while the factor 'duration of air-stream' was not significant (p = 0.463). The interaction effects among the factors were not significant. The results are displayed in Table 2. The XP Bond and Ambar adhesives showed similar bond strength values that were superior to that of Stae (p < 0.05). The deproteinization technique showed higher bond strength than the conventional technique (p < 0.05). The failure modes are described in Figure 4.
The Stae acetone-based adhesive presented the highest solvent content, while the Ambar ethanol-based adhesive showed the lowest solvent concentration. Acetone-based adhesives commonly contain elevated solvent content due to the higher volatility of the acetone compared to other solvents used for dentin adhesives. Acetone presents relatively high vapor pressure (184.3 mmHg at 20℃), as confirmed by the highest evaporation rate values measured for Stae.17 Interestingly, the vapor pressure of ethanol (43.7 mmHg at 20℃) is almost 10 folds greater than that of butanol (4.9 mmHg).18 However, the butanol-based adhesive (XP Bond) presented a higher evaporation rate than the ethanol-based adhesive (Ambar). It was demonstrated that the addition of water to ethanol as a co-solvent increased the retention of both solvents due the formation of hydrogen bonding of these solvents with monomers, which could explain the lowest evaporation rate observed for Ambar and, consequently, the reduced solvent content.1
Considering the differences found among the evaporation rates, it was expected that longer durations of air-stream application would result in higher values of bond strength for adhesives presenting less volatile solvents. However, the duration of air-stream application did not affect the bond strength to dentin of any of the adhesives. Thus, the first hypothesis of the study was rejected. The manufacturers of adhesives recommend air-stream application for times shorter than 10 seconds, which was the shortest time used in the present study. It has been demonstrated that longer durations of air-stream application removed greater amount of solvents from adhesive layers.9,19 Based on the results of this study, it seems possible that additional solvent removal by increasing the duration of the air-stream may not affect the immediate bond strength to dentin. However, it is important to emphasize that the reduction of the solvent in the adhesive layer can affect bonding stability, although no aging of the bond interface was evaluated in the present study.9
Another outcome of this study was that Stae presented the lowest values of bond strength to dentin compared to the other adhesives evaluated. The main differences among the adhesives evaluated were related to their solvent content. Stae presented 2 folds and 4 folds more solvent than XP Bond and Ambar, respectively. Similar to Ambar, Stae also contains water as a co-solvent. However, different from ethanol, acetone does not form an azeotropic mixture with water.20 In the azeotropic mixture between ethanol and water, volatile ethanol evaporates more rapidly until reaching its azeotrope, when both ethanol and water present the same speed of volatilization. Thus, in the absence of an azeotropic mixture, the acetone evaporated rapidly, with large amount of water remaining in the adhesive layer. It has been demonstrated that the presence of residual solvent reduces the cohesive strength of the adhesive layer.21 Thus, possible higher water content remaining in the Stae might explain the results observed for bond strength testing.
Finally, dentin deproteinization increased the bond strength to dentin. However, similar to the conventional technique, the duration of air-stream application did not affect the bond strength when dentin deproteinization was performed. Thus, the second hypothesis of the study was also rejected. Interestingly, despite that ANOVA did not show significant effect for interaction between bonding technique and adhesive, only the Ambar showed higher values of bond strength for dentin deproteinization irrespective of the duration of air-stram. A previous study demonstrated that dentin deproteinization improved the values of bond strength for ethanol-based adhesives presenting low solvent content.12 Adhesives with low solvent content (Ambar is around 10%) presents higher viscosity, which might impair their penetration into collagen mesh of etched dentin.12 In the deproteinization technique, the collagen mesh exposed by acid etching is removed with a NaOCl solution, whereas the bond strength seems to be provided mainly by presence of resin tags.22 Due to the large diameter of the tubules, the adhesive penetration into dentin tubules can occur even when more viscous adhesives are used, favoring the values of bond strength for Ambar.12
Despite the highest values of bond strength being observed with dentin deproteinization, the results of the present study demonstrated that increasing the duration of air-stream application did not affect the bond strength of 2-step etch-and-rinse adhesives to dentin, irrespective of the solvent content or volatilization rate. However, the outcomes of present study must be carefully interpreted once that the stability of bonding interface was not evaluated in the present study.
References
1. Yiu CK, Pashley EL, Hiraishi N, King NM, Goracci C, Ferrari M, Carvalho RM, Pashley DH, Tay FR. Solvent and water retention in dental adhesive blends after evaporation. Biomaterials. 2005; 26:6863–6872. PMID: 15964621.


2. Borges FB, Kochhann DE Lima EL, Machado FW, Boscato N, Van De Sande FH, Moraes RR, Cenci MS. Effect of cariogenic challenge on the stability of dentin bonds. J Appl Oral Sci. 2014; 22:68–72. PMID: 24626251.


3. Pomacóndor-Hernández C, Antunes AN, Hipólito Vd, Goes MF. Effect of replacing a component of a self-etch adhesive by chlorhexidine on bonding to dentin. Braz Dent J. 2013; 24:335–339. PMID: 24173251.
4. Nakabayashi N, Kojima K, Masuhara E. The promotion of adhesion by infiltration of monomers into tooth substrates. J Biomed Mater Res. 1982; 16:265–273. PMID: 7085687.
5. Van Meerbeek B, De Munck J, Yoshida Y, Inoue S, Vargas M, Vijav P, Van Landuyt K, Lambrechts P, Vanherle G. Buonocore memorial lecture. Adhesion to enamel and dentin: current status and future challenges. Oper Dent. 2003; 28:215–235. PMID: 12760693.
6. Reis A, Loguercio AD, Azevedo CL, de Carvalho RM, da Julio Singer M, Grande RH. Moisture spectrum of demineralized dentin for adhesive systems with different solvent bases. J Adhes Dent. 2003; 5:183–192. PMID: 14621240.
7. Cadenaro M, Breschi L, Rueggeberg FA, Suchko M, Grodin E, Agee K, Di Lenarda R, Tay FR, Pashley DH. Effects of residual ethanol on the rate and degree of conversion of five experimental resins. Dent Mater. 2009; 25:621–628. PMID: 19111335.


8. Bail M, Malacarne-Zanon J, Silva SM, Anauate-Netto A, Nascimento FD, Amore R, Lewgoy H, Pashley DH, Carrilho MR. Effect of air-drying on the solvent evaporation, degree of conversion and water sorption/solubility of dental adhesive models. J Mater Sci Mater Med. 2012; 23:629–638. PMID: 22210310.


9. Emamieh S, Sadr A, Ghasemi A, Torabzadeh H, Akhavanzanjani V, Tagami J. Effects of solvent drying time and water storage on ultimate tensile strength of adhesives. J Investig Clin Dent. 2014; 5:51–57.


10. Breschi L, Mazzoni A, Ruggeri A, Cadenaro M, Di Lenarda R, De Stefano Dorigo E. Dental adhesion review: aging and stability of the bonded interface. Dent Mater. 2008; 24:90–101. PMID: 17442386.


11. Lima FG, Moraes RR, Demarco FF, Del Pino FA, Powers J. One-bottle adhesives: in vitro analysis of solvent volatilization and sealing ability. Braz Oral Res. 2005; 19:278–283. PMID: 16491256.
12. Faria-e-Silva AL, Araújo JE, Rocha GP, de Oliveira Ada S, de Moraes RR. Solvent content and dentin bond strengths using water-wet, ethanol-wet and deproteinization bonding techniques. Acta Odontol Scand. 2013; 71:710–715. PMID: 22900709.


13. Reis AF, Oliveira MT, Giannini M, De Goes MF, Rueggeberg FA. The effect of organic solvents on one-bottle adhesives' bond strength to enamel and dentin. Oper Dent. 2003; 28:700–706. PMID: 14653283.
14. Abate PF, Rodriguez VI, Macchi RL. Evaporation of solvent in one-bottle adhesives. J Dent. 2000; 28:437–440. PMID: 10856809.


15. Nihi FM, Fabre HS, Garcia G, Fernandes KB, Ferreira FB, Wang L. In vitro assessment of solvent evaporation from commercial adhesive systems compared to experimental systems. Braz Dent J. 2009; 20:396–402. PMID: 20126908.
16. Silva EM, Duarte PB, Poskus LT, Barcellos AA, Guimarães JG. Nanoleakage and microshear bond strength in deproteinized human dentin. J Biomed Mater Res B Appl Biomater. 2007; 81:336–342. PMID: 17022053.


17. Gallo JR, Burgess JO, Xu X. Effect of delayed application on shear bond strength of four fifth-generation bonding systems. Oper Dent. 2001; 26:48–51. PMID: 11203777.
18. Oktavian R, Amidelsi V, Rasmito A, Wibawa G. Vapor pressure measurements of ethanol-isooctane and 1-butanol-isooctane systems using a new ebulliometer. Fuel. 2013; 107:47–51.


19. Sadr A, Shimada Y, Tagami J. Effects of solvent drying time on micro-shear bond strength and mechanical properties of two self-etching adhesive systems. Dent Mater. 2007; 23:1114–1119. PMID: 17113635.


20. Zheng L, Pereira PN, Nakajima M, Sano H, Tagami J. Relationship between adhesive thickness and microtensile bond strength. Oper Dent. 2001; 26:97–104. PMID: 11203783.
21. Emamieh S, Sadr A, Ghasemi A, Torabzadeh H, Akhavanzanjani V, Tagami J. Effects of solvent drying time and water storage on ultimate tensile strength of adhesives. J Investig Clin Dent. 2014; 5:51–57.


22. Pioch T, Kobaslija S, Schagen B, Götz H. Interfacial micromorphology and tensile bond strength of dentin bonding systems after NaOCl treatment. J Adhes Dent. 1999; 1:135–142. PMID: 11725678.
Figure 2
Box-plot graph for the results of solvent content. Different letters indicate significant differences (p < 0.05).
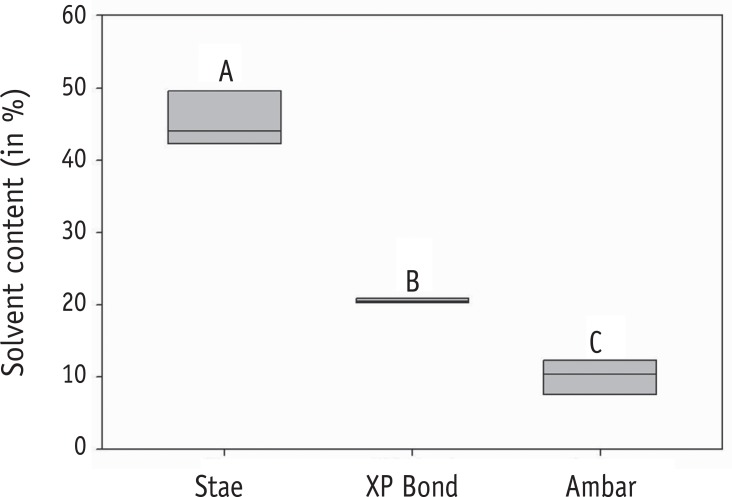
Figure 3
Box-plot graph for the results of evaporation rate. Different letters indicate significant differences (p < 0.05).
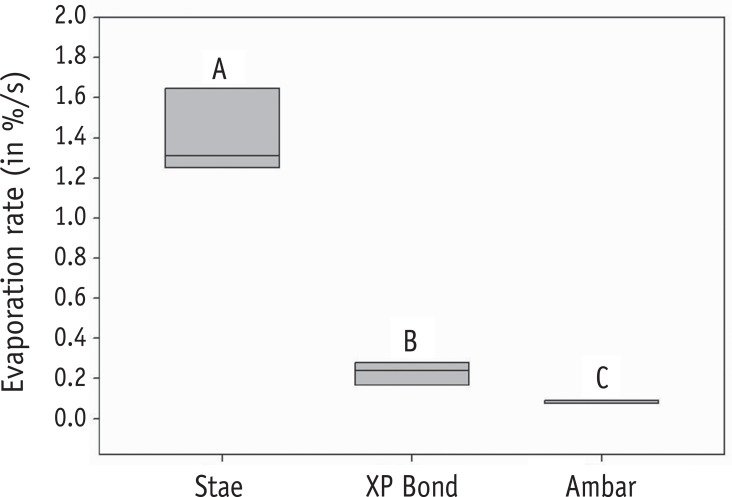
Figure 4
Distribution of failure modes among the experimental conditions. Except for Stae, dentin deproteinization reduced the number of Type I failures and increased the number of Type III failures. Type I, adhesive failure; Type II, mixed failure with more than 50% adhesive; Type III, mixed failure with less than 50% adhesive.
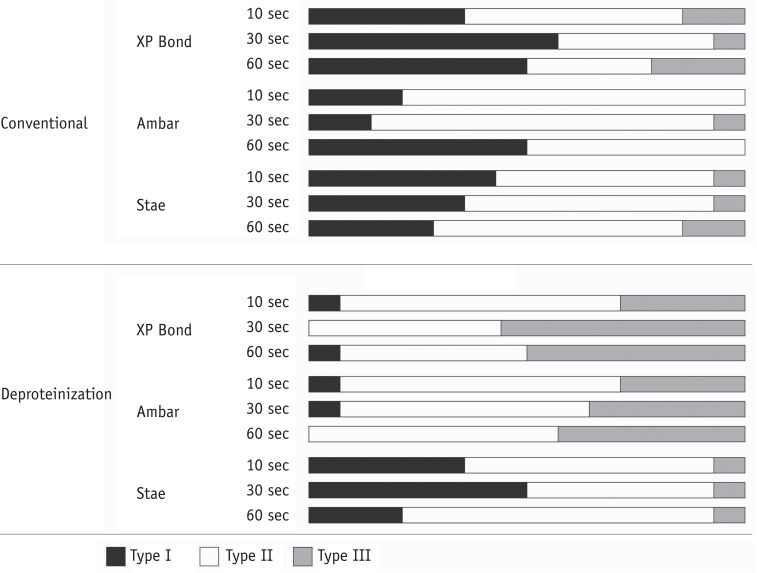
Table 1
Manufacturers and compositions of the adhesives used in this study
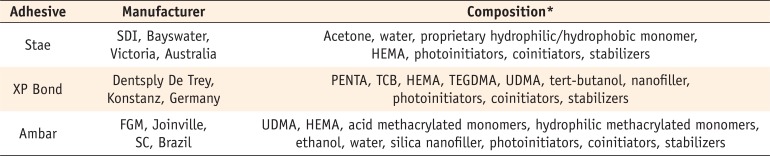
Table 2
Shear bond strength values in MPa
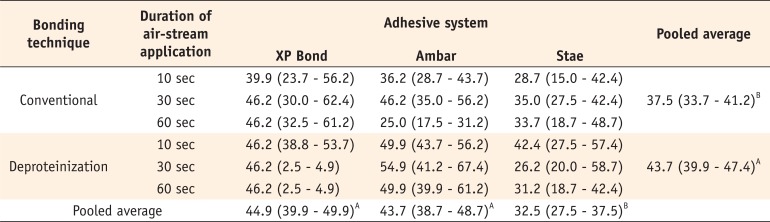