Abstract
Objectives
Light-curing of resin-based materials (RBMs) increases the pulp chamber temperature, with detrimental effects on the vital pulp. This in vitro study compared the temperature rise under demineralized human tooth dentin during light-curing and the degrees of conversion (DCs) of three different RBMs using quartz tungsten halogen (QTH) and light-emitting diode (LED) units (LCUs).
Materials and Methods
Demineralized and non-demineralized dentin disks were prepared from 120 extracted human mandibular molars. The temperature rise under the dentin disks (n = 12) during the light-curing of three RBMs, i.e. an Ormocer-based composite resin (Ceram. X, Dentsply DeTrey), a low-shrinkage silorane-based composite (Filtek P90, 3M ESPE), and a giomer (Beautifil II, Shofu GmbH), was measured with a K-type thermocouple wire. The DCs of the materials were investigated using Fourier transform infrared spectroscopy.
Results
The temperature rise under the demineralized dentin disks was higher than that under the non-demineralized dentin disks during the polymerization of all restorative materials (p < 0.05). Filtek P90 induced higher temperature rise during polymerization than Ceram.X and Beautifil II under demineralized dentin (p < 0.05). The temperature rise under demineralized dentin during Filtek P90 polymerization exceeded the threshold value (5.5℃), with no significant differences between the DCs of the test materials (p > 0.05).
Light-cured resin-based composites (RBCs) have a wide range of applications in dentistry.1 However, they might inflict thermal insults on the vital pulp because of the heat generated by the light source, as well as that from the exothermic polymerization of RBCs.1,2 The 5.5℃ threshold reported by various authors for irreversible changes in the pulp tissue remains controversial among authors.3,4,5,6
A pulp-chamber temperature rise or a rise in the temperature under the dentin has been reported during the light-curing of various adhesives and RBCs by using different light-curing units (LCUs).2,4,6,7,8,9,10 There are three principal techniques for monitoring and recording such temperature rises. The first technique involves placing thermocouples within the pulp chamber via resected roots, the second involves placing thermocouples under a specific dentin thickness, and the third involves using a 'split-tooth' technique for directly visualizing and controlling the remaining dentin thickness (RDT) and the thermocouple.6 It has been reported that when similar light energy levels are used, the temperature increase is greater during the light-curing of adhesives than that during the light-curing of RBCs, low-energy light sources result in similar or lower temperature rises.6,7,11
The temperature rise during the light-curing of resin-based dental materials has been measured and evaluated in a large number of in vitro studies using ground or sound dentin substrates.2,3,4,5,9,11,12,13 Nonetheless, sound dentin substrates are not usually encountered in clinical situations, and dental practitioners generally restore carious dentin. Carious dentin usually consists of layers of infected and affected dentin. Affected dentin is not usually removed during restorative procedures.14 As a result, after removal of the carious lesion and preparation of the cavity during adhesive procedures, a large part of the cavity floor contains caries-affected dentin.14
Very few studies have measured the temperature rise during the light-curing of resin-based dental materials under normal and affected dentin substrates.15,16 Fanibunda reported that carious dentin has considerably higher thermal conductivity, which implies that the material is a poor thermal insulator, compared to sound normal dentin.16 Tosun et al. evaluated the temperature rise under normal sound and caries-affected dentin in primary teeth during the light-curing of two adhesives and two resin-based restorative materials.15 They reported that the temperature rise during adhesive material polymerization exceeded 5.5℃ under the caries-affected primary tooth dentin. In addition, adhesive systems exhibited a higher temperature rise during polymerization than did RBMs.
Presently, new classes of highly filled RBCs are available with a filler content and distribution pattern that endows them with different handling characteristics.1,17 A different resin-based material has been manufactured using the Ormocer technology. This newly developed inorganic/organic copolymer characterizes Ormocers, thereby paving the way for modifying its mechanical parameters and properties.6,17 In addition, giomer is a proprietary product composed of inorganic fillers and an organic/resin matrix.18 Rather than incorporating glass or quartz particles, as is the case with traditional fillers, it contains inorganic fillers originating from the complete or partial reaction of ion-leachable glasses.18,19 In recent years, a new silorane-based composite resin characterized by low polymerization shrinkage has been marketed.19 The introduction of a silorane-based composite paves the way for reducing polymerization shrinkage and balancing volumetric stresses due to polymerization shrinkage. Despite preliminary research studies on the silorane-based matrix demonstrating significant decreases in polymerization shrinkage, there is limited research on the influence of silorane monomers on the physical and mechanical properties of polymerized composite materials.19
Several studies have compared the use of light-emitting diode (LED) and quartz tungsten halogen (QTH) LCUs with respect to RBC polymerization, with some evaluating the temperature rise under dentin or caries-affected dentin substrates.2,6,15,16,20,21,22,23,24,25,26,27 However, to date, no study has evaluated temperature variations during the light-curing of a number of newly introduced materials, including low-shrinkage silorane-based composites, Ormocer-based resin composites, and giomers, under artificially demineralized dentin. The present study aimed to achieve the followings, 1) comparison of the efficacy of the LED LCU and halogen LCU in increasing the degree of conversion (DC) of three resin-based materials (RBMs), namely, an Ormocer-based composite resin (Ceram.X, Dentsply DeTrey, Konstanz, Germany), a low-shrinkage silorane-based composite resin (Filtek P90, 3M ESPE, St. Paul, MN, USA), and a giomer (Beautifil II, Shofu GmbH, Ratingen, Germany), and 2) comparison of the temperature rise during the light-curing of the three materials using an LED and a QTH LCU under artificially demineralized dentin. The null hypothesis was that there would be no difference in temperature variations between the three RBMs under mineralized and demineralized dentin substrates during light-curing with QTH or LED LCUs. Furthermore, it was also hypothesized that there was no difference between the DC values of the three abovementioned materials.
The particulars of the LCUs and the RBMs used in this study are summarized in Tables 1 and 2. The QHT LCU, Demetron LC (Kerr GmbH, Rastatt, Germany), was used in the standard mode, which implies a full irradiation intensity of 550 mW/cm2 from the beginning to the end of the procedure. An LED LCU, Bluphase (Kerr GmbH), was used with a low irradiation intensity of 500 mW/cm2 for evaluating the effect of the LCU. An Optilux radiometer and an LED radiometer were used for measuring the output energy levels of the LCUs (Table 1).
The protocol followed in the present study was approved by the Human Ethics Committee of the School of Dentistry, Isfahan University of Medical Sciences. One hundred twenty extracted human mandibular molars without any defects or caries were used in this study. The samples were stored in 0.1% thymol solution and used within four months from extraction. One day before the study procedure was instituted, the teeth were retrieved from the thymol solution, rinsed thoroughly and immersed in distilled water at 37℃.
Dentin disks with a thickness of 1.5 mm and a diameter of 4 mm were prepared from the deep dentin of 120 third molars. To this end, each tooth was mounted in epoxy resin (Triplex Acryl, Ivoclar Vivadent, Schaan, Liechtenstein) and sections parallel to the long axis of each tooth were cut using a low-speed diamond saw (SERVOCUT 301MA, Kemet International Ltd., Maidstone, UK) under water spray. Subsequently, perpendicular cuts were made for preparing dentin disks with a thickness of 1.5 mm (Figure 1). Dentin sample surfaces were wet-ground with 320- and 400-grit silicon carbide abrasive papers under water spray for achieving flat dentin surfaces.
Half of the disks were immersed in a demineralizing solution for obtaining artificially demineralized dentin disks similar to caries-like lesions in dentin. Each disk was immersed in 20 mL of a demineralizing solution for 48 hours at 23℃.14 The solution consisted of 0.002 mole/L CaCl2, 0.002 mole/L KH3PO4, and 0.002 mole/L glacial acetic acid, and the pH of the solution was 4.3. All the dentin disk samples were stored in water until testing to prevent dehydration.
The temperature rise during the 40-second irradiation with each LCU was recorded for each RBM without any dentin disk. The ambient temperature was 23 ± 1℃. For evaluating the influence of the dentin disk's structural variables on temperature variations, the increase in temperature was measured separately under the dentin disks before insertion of the restorative material and during the light-curing of each RBM.
The materials were placed on dentin disks and light-cured according to the manufacturers' instructions to measure temperature variations during curing in the RBMs, including an Ormocer-based composite resin (Ceram.X), a low-shrinkage silorane-based composite resin (Filtek P90), and a giomer (Beautifil II) (Table 2). To this end, a black Teflon mold was prepared to serve as a support for the light guide tip, dentin disks, and the resin material. The resin material was placed in the central aperture of the Teflon cylinder mold and polymerized according to the manufacturers' instructions (Table 1). A digital radiometer (Curing Radiometer, Demetron Inc., Danbury, CT, USA) was used for measuring and recording the output energy level of the LCU before each procedure.
A K-type thermocouple wire with a diameter of 0.1 cm (ST-8891E, Standard Instruments Co., Ltd., Kowloon, Hong Kong) was connected to a data logger (Standard, ST-8891E, Taiwan) during the light-curing of the resin materials (Figure 2).6 The data logger recorded temperature variations at a rate of 1 sample/second from the start of light-curing to almost 120 - 180 seconds until the temperature decreased. The data were recorded and stored in a computer. The mean of ten readings of the temperature rise was calculated at a pre-determined light output energy at a distance of 3.5 mm from the thermocouple when the light guide tip was secured in place on the mold. The difference between the initial and the peak temperature readings was determined, and the 10 recorded temperature changes were averaged to arrive at the mean temperature rise value. In addition, the temperature rise under a dentin disk during irradiation was measured for simulating the temperature rise in the pulp chamber in clinical situations (Figure 2). The LCU tip was adjusted at the center of each resin material with no gaps in between.
The temperature rise during the 40-second exposure was due to both the heat of the polymerization reaction and the heat generated by irradiation. The temperature rise in the 2-mm-thick samples of each material during irradiation, mediated with non-demineralized and demineralized dentin, was recorded. This final thermal rise was the difference between the thermal change due to LCU irradiation and that due to RBM polymerization.
There were 10 samples in each group (Figure 1). Data were analyzed with SPSS 13.5 (SPSS Inc., Chicago, IL, USA) at a 0.05 level of significance. Data were analyzed by three-way ANOVA (dentin type, dental material, and LCU). Paired sample t-test and post hoc Tukey tests were used to evaluate the inter-group differences.
The DCs of the two dimethacrylate-based restorative materials, that is, Ceram.X and Beautifil II, were measured using Fourier transform infrared (FTIR) spectroscopy (EQUINOX 55, Bruker, Ettlingen, Germany). A small piece of the resin composite was placed between two polyethylene layers and pressed to form a very thin film, and the absorbance peaks of the uncured samples were obtained. Subsequently, the samples were light-cured using the same time durations as those used for the temperature rise readings, and the peaks of each light-cured sample were recorded.
The DC (%) was determined from the ratio of the absorbance intensities of aliphatic C=C (peak at 1,638 cm-1) against the internal reference aromatic C=C (peak at 1,608 cm-1) prior and subsequent to light-curing. The DC was calculated as follows,20
For Filtek P90, the DC was calculated using the ratio of absorbance intensities of the reacting epoxy rings C-O-C (peak at 884 cm-1) against the Si-CH3 internal reference (peak at 695 cm-1) prior and subsequent to light-curing.20 The DC was calculated as follows,
The results were analyzed and compared using one-way ANOVA, followed by a post hoc Tukey test at a significance level of 0.05.
Table 3 presents the mean values of temperature variations during the light-curing of the three resin-based restorative materials. During the 40-second irradiation of each LCU without any dentin disk and RBM, the temperature rise values were 21.21℃ and 18.19℃ for the QTH and LED LCUs, respectively. The mean values of the temperature rise under the QTH and LED LCUs were 7.28 ± 0.33℃ and 6.26 ± 1.10℃, respectively, for non-demineralized dentin. The same mean values for demineralized dentin were 7.06 ± 0.35℃ and 7.02 ± 0.43℃, respectively. For both mineralized and non-demineralized dentin, there were no significant differences between the mean temperature values of the two LCUs (p > 0.05).
The Kolmogorov-Smirnov test showed that the temperature rise in all the test groups was normally distributed. Three-way ANOVA was used for analyzing the effect of the three variables, namely, the LCU, dentin condition, and restorative material, on the temperature rise during the photopolymerization of the resin-based restorative materials. The analysis revealed that the temperature rise was affected by the LCU (F = 8.008, p < 0.001), dentin condition (F = 24.782, p < 0.001), and the restorative material (F = 15.472, p < 0.001). Two-way ANOVA showed significant differences in the means of the temperature rise values among all the restorative materials for the demineralized and non-demineralized dentin (p = 0.012) and the LCU (p < 0.001).
The QTH LCU resulted in a higher temperature rise during light-curing compared to the LED LCU (p < 0.001). The effects of dentin, LCU and material variables were evaluated through a paired sample t-test. The results showed a significant difference in the mean thermal rise during irradiation with QTH (21.21 ± 0.15℃) and LED (18.19 ± 0.13℃) LCUs (p < 0.001). The post hoc Tukey test, which was used to determine the differences in the temperature rise among the groups within the materials, showed that the temperature rise values measured under demineralized dentin were higher than those measured under non-demineralized dentin during the light-curing of all the resin-based restorative materials (p = 0.012). The highest final temperature rise was recorded during the light polymerization of Filtek P90 under demineralized dentin with the QTH LCU (6.48 ± 0.86℃), followed by that recorded in the corresponding group with the LED LCU (6.25 ± 1.20℃), as listed in Table 3.
Table 4 lists the DC of resin composites light-cured using two LCUs. The curing conditions were identical to those during the temperature rise measurements. The DC values of the RBMs cured by the QTH and LED LCUs were from 53 ± 0.11% to 60 ± 0.02% and from 52 ± 0.11% to 63 ± 0.08%, respectively, with no significantly higher DC for RBCs with either the LED or the QTH LCUs (p = 0.201).
It is well known that certain dental procedures increase the tooth temperature, including those of the hard tissues and the pulp.28 Moreover, nowadays, there are different pieces of equipment for RBM polymerization.2,7,29 The present study showed that the radiation-induced temperature rise in all the 12 study groups was higher than the overall temperature rise. This indicates that the most important etiologic agent for temperature rise during the light-curing of composite resins is the heat produced by the LCUs. Therefore, the exothermic polymerization of composite resins is a secondary factor. However, temperature variations due to light activation with a gross temperature rise due to the use of an LED LCU were not statistically significant for Filtek P90. In addition, the final temperature rise due to irradiation by QTH was not significantly greater than that produced by the LED LCU for this composite resin. This indicates that the temperature rise due to photopolymerization in this composite is higher than that in Ceram.X and Beautifil II. In a study by Miletic et al., Filtek LS exhibited a greater temperature rise than two methacrylate-based composite resins (Admira and Herculite XRV), which is consistent with the results of the present study.27
In the current study, the temperature rise due to irradiation and the total temperature rise induced by the QTH LCU were higher than those in the case of the LED LCU. In other words, the LCU effect was significant. In accordance with the results of the present study, some previous studies have shown that the temperature rise is significantly higher when composite resins are cured using QTH LCUs than that when using the PAC or LED LCUs.12,13,14,15 Dogan et al. reported that when manufacturer-recommended irradiation durations were used, the QTH LCU produced significantly higher temperature rises than the LED and PAC LCUs under all the test conditions.5 Tarle et al. reported that irrespective of the curing mode, lower temperatures were achieved when a blue LED light was used.21 In addition, Knezevic et al. reported that the LED LCU generates slightly lower conversion values and a significantly lower temperature increase than conventional lamps.2 Contrary to these reports, Atai and Motevasselian reported no significant differences in temperature rise and the DC between the standard mode of Optilux 501 as the QTH and the LED LCUs.4
The results of the current study showed that the temperature rise under the demineralized dentin disks was higher than that under the normal dentin disks. Tosun et al. reported recording a higher temperature rise during light-curing when caries-affected dentin was used as the substrate than that when normal dentin was used as the substrate.15 Fanibunda reported that carious dentin has a significantly higher thermal conductivity, indicating lower thermal insulation, than normal dentin.16 Electron probe microanalysis showed that the elemental contents of normal and caries-affected dentin substrates were different.30 These findings can explain the differences between the temperature rise values of normal and caries-affected dentin. To date, only a limited number of studies have measured temperature variations during the light activation of resin-based restorative materials under normal and demineralized dentin.15,16
Based on the results of this study, the temperature rises during the light-curing of Filtek P90 were 5.47 and 5.58℃ with the QTH and LED LCUs, respectively. In demineralized dentin, these values were 6.48 and 6.25℃ with the QTH and LED LCUs, respectively. The overall temperature rise with Filtek P90 was significantly higher than that with methacrylate-based composite resins. The temperature rise in the methacrylate-based composite (Ceram.X and Beautifil II) did not exceed the 5.5℃ rise obtained in two previous studies by Zach and Cohen, and Pohto and Scheinin, who speculated that any temperature rise exceeding 5.5℃ can induce irreversible pulpitis.3,31
In a study by Miletic et al. the silorane-based Filtek P90 exhibited a significantly different temperature curve with higher values in comparison with two dimethacrylate-based composite resins.27 It has been shown in similar studies that siloxane-based and oxirane-based composite resins lead to a greater temperature rise than methacrylate-based resins.32 In addition, studies employing optical pyrometry have shown that the cationic ring opening polymerization of oxiranes is a highly exothermic reaction, with temperatures exceeding 100℃ within seconds.27 Studies have shown that the temperature curves generated during the light activation of Filtek P90 and the heat generation pattern seen in Filtek P90 are affected by the slow heat diffusion through dentin.1,27
Temperature variations during the light-curing of RBMs were evaluated under 1.5 mm of dentin in the current study. Temperature variations reported in several studies have been on the order of 1 - 15℃ depending on the LCU, RDT, RBM, and measurement technique used. Al Qudah et al. suggested that an RDT of 1.9 mm or more is sufficient to protect the pulp from any thermal insults during the light activation of composite resins, which is close to the thickness of the dentin disks used in the present study.32 Their report was confirmed by Lonely, who suggested that an RDT of 2 mm would provide adequate insulation against restorative procedures.33 The speculation was based on a previous report by Stanely and Swerdlow, who found that cavity preparation 2 mm away from the pulp elicited minimal pulpal response.34 Hence, in the present study, a dentin thickness of 1.5 mm was used for evaluating temperature variations. According to Lonely and Price, a thicker dentin can significantly cope with temperature variations because of low thermal conductivity of dentin.33 Haning and Bott reported that for an LCU energy output of 670 mW/cm2, irradiation time of 40 seconds, and dentin thickness of 1 mm, the temperature rise did not exceed 5.5℃.35 The overall temperature rise in the present study was consistent with that in previous studies.32,33,34,35,36 However, it is important to note that clinically, the surfaces of most restorations are in contact with the dentin walls and the dentinal fluid within them. In addition, blood circulation in the pulp chamber diffuses the heat absorbed by the pulp. These factors and similar variables are the limitations of experimental studies, including the present study, and should not be ignored.
The DCs of three different RBMs were measured in this study. The results of material evaluations, including those of tests for mechanical strength, modulus, hardness, and leachable components, are related to polymer conversion. Therefore, DC evaluation is a critical component of interpreting the test results of dental materials.6 A high DC is important for appropriate clinical performance of dental materials. A low DC leads to poor wear resistance and low color stability.1,17
The DCs of the RBMs were evaluated using FTIR spectroscopy in the curent study. Of the several techniques used to determine the DC of RBCs, FTIR spectroscopy has been reported to be powerful and has been widely used.4 In addition, in this study, the degree of RBM polymerization was measured with a QTH LCU and an LED LCU with almost similar output powers (22 and 20 J/cm2, respectively). The DC values varied from 52 to 63%. According to the results, neither the LCU nor the restorative material is a significant factor in DC (p = 0.621 and p = 0.130, respectively). Previous studies have confirmed that DC depends on the material composition, light source properties, distance from the light source, and duration of irradiation. It ranges from 43.5 to 73.8% when standard curing is employed.23,36 Stupp and Weertman reported a DC range of 30 - 70% for nine proprietary self-cured composite resins, and Asmussen reported a range of 57 - 77% for nine proprietary Bis-GMA-based anterior composite resins.37,38 Ferracane and Greener studied unfilled Bis-GMA-based resins and reported that the DC value for these experimental resins varied between 55 and 72%.39 Recently, a study showed that the light polymerization of P90 low-shrinkage composite resin resulted in a temperature rise approaching the threshold value under artificially demineralized and undemineralized dentin.40
According to the results of this study, the DC values of Ceram.X with QTH and LED LCUs were 59 and 63%, respectively, with no statistically significant differences (p = 0.201). It appears that the lower filler content of Ceram. X and the larger filler sizes than those of Beautifil II result in an increased DC% in this material. A smaller filler size might be responsible for greater scattering of light in the superficial layers of the composite resin, thus decreasing the penetration of light into the deep layers.1,17
In terms of temperature rise, it might be appropriate to say that the temperature rise due to light irradiation is more significant and important than that due to photopolymerization when Ceram.X and Beautifil II are compared. However, temperature changes due to the polymerization of Filtek P90 were significantly higher than those in the case of the two other composite resins (p < 0.001). Moreover, Filtek P90 exhibited the highest temperature changes and DC values among the three materials studied, with no statistically significant differences in DC (p = 0.130). This outcome could be attributed to the number of DC evaluations, which was three. However, it seems there is a relationship between ΔTt and DC in silorane-based composite resins, and this needs to be evaluated in future studies.
Under the limitations of this in vitro study, it was concluded that the temperature rise resulting from irradiation with QTH was higher than that with the LED LCU. In addition, the temperature rise through all the studied RBMs under demineralized dentin was higher than that under non-demineralized dentin. The temperature rise through Filtek P90 was higher than that through Ceram.X and Beautifil II and the presence of 1.5-mm-thick dentin diminished the temperature rise during the light polymerization of the RBMs under study. Finally, the DC values of all the RBMs studied were not significantly different.
Figures and Tables
Figure 1
Schematic representation of the different steps for the preparation of dentinal discs. LCU, light-curing unit; QTH, quartz tungsten halogen; LED, light-emitting diode.
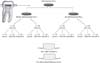
Figure 2
Schematic representation of the temperature rise measurement during polymerization of the resin material under the dentin disc.
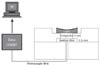
Acknowledgments
The authors gratefully acknowledge that this report is based on a part of thesis that was submitted to the School of Dentistry, Isfahan University of Medical Sciences, in partial fulfillment of the requirements for the MSc degree in Operative Dentistry (#390343). This study was financially supported and approved by Isfahan University of Medical Sciences, Isfahan, Iran.
References
2. Knezević A, Tarle Z, Meniga A, Sutalo J, Pichler G. Influence of light intensity from different curing units upon composite temperature rise. J Oral Rehabil. 2005; 32:362–367.


3. Zach L, Cohen G. Pulp response to externally applied heat. Oral Surg Oral Med Oral Pathol. 1965; 19:515–530.


4. Atai M, Motevasselian F. Temperature rise and degree of photopolymerization conversion of nanocomposites and conventional dental composites. Clin Oral Investig. 2009; 13:309–316.


5. Dogan A, Hubbezoglu I, Dogan OM, Bolayir G, Demir H. Temperature rise induced by various light curing units through human dentin. Dent Mater J. 2009; 28:253–260.


6. Yoon TH, Lee YK, Lim BS, Kim CW. Degree of polymerization of resin composites by different light sources. J Oral Rehabil. 2002; 29:1165–1173.


7. Bala O, Olmez A, Kalayci S. Effect of LED and halogen light curing on polymerization of resin-based composites. J Oral Rehabil. 2005; 32:134–140.


8. Santini A, Watterson C, Miletic V. Temperature rise within the pulp chamber during composite resin polymerisation using three different light sources. Open Dent J. 2008; 2:137–141.


9. Bouillaguet S, Caillot G, Forchelet J, Cattani-Lorente M, Wataha JC, Krejci I. Thermal risks from LED- and high-intensity QTH-curing units during polymerization of dental resins. J Biomed Mater Res B Appl Biomater. 2005; 72:260–267.


10. Mousavinasab SM, Meyers I. Comparison of depth of cure, hardness and heat generation of LED and high intensity QTH light sources. Eur J Dent. 2011; 5:299–304.


11. Hussey DL, Biagioni PA, Lamey PJ. Thermographic measurement of temperature change during resin composite polymerization in vivo. J Dent. 1995; 23:267–271.


12. Durey K, Santini A, Miletic V. Pulp chamber temperature rise during curing of resin-based composites with different light-curing units. Prim Dent Care. 2008; 15:33–38.


13. Ratih DN, Palamara JE, Messer HH. Temperature change, dentinal fluid flow and cuspal displacement during resin composite restoration. J Oral Rehabil. 2007; 34:693–701.


14. Yang B, Flaim G, Dickens SH. Remineralization of human natural caries and artificial caries-like lesions with an experimental whisker-reinforced ART composite. Acta Biomater. 2011; 7:2303–2309.


15. Tosun G, Usumez A, Yondem I, Sener Y. Temperature rise under normal and caries-affected primary tooth dentin disks during polymerization of adhesives and resin-containing dental materials. Dent Mater J. 2008; 27:466–470.


16. Fanibunda KB. Thermal conductivity of normal and abnormal human dentine. Arch Oral Biol. 1975; 20:457–459.


18. Shofu Dental Corporation SI: About Giomer. updated 2012. Available from: http://www.shofu.com/index.php/restoratives/187#name.
19. Lien W, Vandewalle KS. Physical properties of a new silorane-based restorative system. Dent Mater. 2010; 26:337–344.


20. Goracci C, Cadenaro M, Fontanive L, Giangrosso G, Juloski J, Vichi A, Ferrari M. Polymerization efficiency and flexural strength of low-stress restorative composites. Dent Mater. 2014; 04. 02. pii: S0109-5641(14)00082-7. doi: 10.1016/j.dental.2014.03.006.[Epub ahead of print].


21. Tarle Z, Knezevic A, Demoli N, Meniga A, Sutaloa J, Unterbrink G, Ristic M, Pichler G. Comparison of composite curing parameters: effects of light source and curing mode on conversion, temperature rise and polymerization shrinkage. Oper Dent. 2006; 31:219–226.


22. Bouillaguet S, Caillot G, Forchelet J, Cattani-Lorente M, Wataha JC, Krejci I. Thermal risks from LED- and high-intensity QTH-curing units during polymerization of dental resins. J Biomed Mater Res B Appl Biomater. 2005; 72:260–267.


23. Tarle Z, Meniga A, Ristic M, Sutalo J, Pichler G, Davidson CL. The effect of the photopolymerization method on the quality of composite resin samples. J Oral Rehabil. 1998; 25:436–442.


24. Yap AU, Soh MS. Thermal emission by different light-curing units. Oper Dent. 2003; 28:260–266.
25. Mousavinasab SM, Meyers I. Curing efficacy of light emitting diodes of dental curing units. J Dent Res Dent Clin Dent Prospects. 2009; 3:11–16.
26. da Silva EM, Penelas AG, Simão MS, Filho JD, Poskus LT, Guimarães JG. Influence of the degree of dentine mineralization on pulp chamber temperature increase during resin-based composite (RBC) light-activation. J Dent. 2010; 38:336–342.


27. Miletic V, Ivanovic V, Dzeletovic B, Lezaja M. Temperature changes in silorane-, ormocer-, and dimethacrylate-based composites and pulp chamber roof during light-curing. J Esthet Restor Dent. 2009; 21:122–131.


28. Kwon SJ, Park YJ, Jun SH, Ahn JS, Lee IB, Cho BH, Son HH, Seo DG. Thermal irritation of teeth during dental treatment procedures. Restor Dent Endod. 2013; 38:105–112.


29. Kwon Y, Kim SY, Chung SJ, Han YC, Lee IB, Son HH, Um CM, Cho BH. Shear bond strength of dentin bonding agents cured with a Plasma Arc curing light. J Korean Acad Conserv Dent. 2008; 33:213–223.


30. Nakajima M, Kitasako Y, Okuda M, Foxton RM, Tagami J. Elemental distributions and microtensile bond strength of the adhesive interface to normal and caries-affected dentin. J Biomed Mater Res B Appl Biomater. 2005; 72:268–275.


31. Pohto M, Scheinin A. Microscopic observations on living dental pulp. II. The effect of thermal irritants on the circulation of the pulp in the lower rat incisor. Acta Odontol Scand. 1958; 16:315–327.


32. Al-Qudah AA, Mitchell CA, Biagioni PA, Hussey DL. Thermographic investigation of contemporary resin-containing dental materials. J Dent. 2005; 33:593–602.


33. Loney RW, Price RB. Temperature transmission of high-output light-curing units through dentin. Oper Dent. 2001; 26:516–520.
34. Stanley HR Jr, Swerdlow H. Reaction of the human pulp to cavity preparation: results produced by eight different operative grinding technics. J Am Dent Assoc. 1959; 58:49–59.


35. Hannig M, Bott B. In vitro pulp chamber temperature rise during composite resin polymerization with various light-curing sources. Dent Mater. 1999; 15:275–281.


36. Chung K, Greener EH. Degree of conversion of seven visible light-cured posterior composites. J Oral Rehabil. 1988; 15:555–560.


37. Navarra CO, Cadenaro M, Armstrong SR, Jessop J, Antoniolli F, Sergo V, Di Lenarda R, Breschi L. Degree of conversion of Filtek Silorane Adhesive System and Clearfil SE Bond within the hybrid and adhesive layer: an in situ Raman analysis. Dent Mater. 2009; 25:1178–1185.


38. Asmussen E. Restorative resins: hardness and strength vs. quantity of remaining double bonds. Scand J Dent Res. 1982; 90:484–489.

