Abstract
A role of nitric oxide (NO) in the regulation of sodium transporters and water channels in the salivary gland was investigated. Male Sprague-Dawley rats were treated with NG-nitro-L-arginine methyl ester (L-NAME, 100 mg/L drinking water) for 1 week. The control group was supplied with normal tap water. The expression of Na+,K+-ATPase, type 2 Na+/K+/2Cl- cotransporter (NKCC2), type 1 Na+/H+ exchanger (NHE1), α-subunit of epithelial sodium transporter (ENaC), and aquaporin-5 (AQP5) and aquaporin-1 (AQP1) proteins were determined in the submandibular gland by Western blot analysis. Following the treatment with L-NAME, the expression of Na+,K+-ATPase α1-subunit, NKCC2, NHE1, and ENaC α-subunit increased significantly. On the contrary, the expression of AQP5 was significantly decreased, while that of AQP1 was not significantly altered. These findings indicate that the sodium transporters and water channels may be under a tonic regulatory influence of NO in the salivary gland.
Salivary glands are composed of acinar cells, either serous or mucous, and ductal cells of several varieties. The primary saliva is formed in the lumen of the acinar cells, where the accumulation of ions generates a transepithelial osmotic gradient driving water movement. In the second stage of secretion, sodium is actively reabsorbed while the primary saliva passes through the duct. Therefore, alterations of sodium transporters and water channels may affect the formation and the ductal modification of the saliva.
Immunofluorescence labelling and confocal microscopy revealed a polarized distribution of Na+,K+-ATPase, the secretory isoform of Na+/K+/2Cl- cotransporter (NKCC2), and type 1 Na+/H+ exchanger (NHE1) in the basolateral membranes of acinar and intralobular duct cells1). Na+,K+-ATPase produces an inward-directed sodium chemical gradient in the acinar cell2). Although sodium influx is negligible in resting cells, it is rapidly increased by activation of NKCC2, NHE1, and nonselective cation channels in the acinar cell3). Blockade of NKCC2 causes significant reduction of acetylcholine-stimulated secretion4), in which the residual secretion is attributed to the operation of Na+/H+ and Cl-/HCO3- antiport5, 6). The amiloride-sensitive epithelial sodium channels (ENaC) are localized to the surface epithelial cells of the secretory duct. Among their three homologous subunits, only α-subunit can produce an amiloride-sensitive current7), while β- and γ-subunits are not functional on their own8).
On the other hand, there have been known multiple isoforms of aquaporin (AQP) water channels in the salivary gland. Among them, AQP5 may be the only isoform of AQP playing a major role in the salivary secretion process. Strong AQP5 labelling is located in the apical membrane of serous-type acinar cells from the rat submandibular gland9). The transepithelial water movement occurs through the apical AQP5 channels and paracellular pathways10), leading to the secretion of isotonic primary saliva. Although the presence of AQP1 and AQP8 has been also generally accepted, their role in the salivary secretion remains controversial11).
Over the last two decades, nitric oxide (NO) has been found to play a role in the regulation of various physiological functions. It has been also implicated in mediating the nerve-evoked vasodilator and secretory responses in the salivary gland. Parasympathetic nerve activity increases the submandibular blood flow, and elicits the flow of saliva and output of protein by mechanisms that involve in situ generation of NO in the ferret12). A previous study revealed that NO synthase (NOS) is not present in the acinar cells, but in neural terminals within the gland and in the apical membrane of the excretory and striated ducts, the cytoplasm of granular convoluted tubules, and, to a lesser extent, in the cytoplasm of excretory and striated ducts13). However, later investigators demonstrated that acinar cells also posses endogenous NOS activity14). Furthermore, the salivation induced by cholinergic agonists or that produced by the chorda stimulation was decreased by inhibition of NO synthesis by NG-nitro-L-arginine methyl ester (L-NAME) in the rat submandibular gland11, 15).
Nevertheless, the role of NO in the regulation of sodium transporters and AQP channels in the salivary gland has not been established. Therefore, the present study was aimed to explore the role of NO in the regulation of sodium transporters and AQP channels in the salivary gland. Rats were inhibited of endogenous generation of NO by treatment with L-NAME, and the expression of sodium transporters and water channels was determined in the submandibular gland.
Male Sprague-Dawley rats (200-250 g) were used. The experimental group was treated with L-NAME (100 mg/L drinking water) for 1 week. The control group was supplied with normal tap water. The experimental procedure conformed to the Institutional Guidelines for Experimental Animal Care and Use.
The submandibular glands were rapidly isolated under ketamine anesthesia. They were rapidly frozen and kept at -70℃ until analyzed. For protein preparation, they were thawed and homogenized with Polytron homogenizer at 3,000 rpm in a solution containing sucrose (250 mmol/L), EDTA (1 mmol/L), phenylmethylsulfonyl fluoride (0.1 mmol/L), and potassium phosphate buffer (20 mmol/L), at pH 7.6. The homogenate was centrifuged at 1,000 g for 15 min to remove the whole cells, nuclei, and mitochondria. The supernatant was then ultracentrifuged at 100,000×g for 1 h to produce a pellet containing membrane fractions enriched for both plasma membranes and intracellular vesicles. The pellet was resuspended in homogenizing solution for protein blotting.
Protein samples were loaded and electrophoretically size-separated with a discontinuous system consisting of 8-12.5% polyacrylamide resolving gel and 5% polyacrylamide stacking gel. The proteins were then transferred to a nitrocellulose membrane at 40 V for 3 h. The membrane was washed in Tris-based saline buffer (pH 7.4) containing 0.1% Tween-20 (TBST), blocked with 5% nonfat milk in TBST for 1 h, and incubated with antibodies in 2% nonfat milk/TBST for 1 h at room temperature. The antibodies used were polyclonal anti-rabbit α-1 and β-1 subunits of Na+,K+-ATPase (1:2,500, Upstate Biotechnology, Lake Placid, NY, USA), NKCC2 (1:1,000, Chemicon, Temecula, CA, USA), NHE1 (1:500, Alpha Diagnostic, San Antonio, TX, USA), α-subunit of ENaC (1:500, Alpha Diagnostic, San Antonio, TX, USA), AQP1 (1:1,000, Alomone, Jerusalem, Israel), AQP5 (1:1000, Alpha Diagnostic, San Antonio, TX, USA), endothelial NOS (eNOS) and neuronal NOS (nNOS) (1:750, Transduction; Lexington, KY, USA). β-Actin was used as internal control (1:1,000, Sigma, St. Louis, MO, USA). The membrane was then incubated with horseradish peroxidase-labeled goat anti-rabbit IgG (1:1,200) in 2% nonfat milk in TBST for 2 h. The bound secondary antibody was detected by enhanced chemiluminescence (Amersham, Buckinghamshire, England). The protein levels were determined, using the transmitter scanning videodensitometer (Biomed Instruments, Fullerton, CA, USA).
In the L-NAME-treated group, the systolic blood pressure measured indirectly by the tail cuff method in a conscious state was significantly higher in the experimental group than in the control (146±9 vs 121±6 mmHg, p<0.05, n=6 each). Accordingly, the expression of eNOS and nNOS in the submandibular gland was decreased significantly (Fig. 1). The amount of β-actin was not significantly altered (Fig. 2). On the contrary, the expression of α1-subunit of Na+,K+-ATPase was significantly increased, although that of β1-subunit remained unchanged (Fig. 3). The expression of NKCC2, NHE1, and α-subunit of ENaC was increased significantly (Fig. 4). The expression of AQP5 was significantly decreased, while that of AQP1 was not significantly altered (Fig. 5).
NO has been implicated in mechanisms mediating nerve-evoked vasodilator and secretory responses in salivary glands. The salivation induced by cholinergic agonists or chorda stimulation was decreased by NO synthesis inhibition in the submandibular gland12, 13, 15). The formation of primary and secondary saliva involves transepithelial electrolyte and water movement. Therefore, it is plausible to hypothesize that NO plays a role in regulating the sodium transporters and AQP channels in the salivary gland.
Previous studies have shown that L-NAME treatment decreases the gene and protein expression of eNOS16, 17). The present study also demonstrated a decreased expression of both eNOS and nNOS in the submandibular gland following the treatment with L-NAME, which may have resulted in a decreased formation of NO. The down-regulation of NOS was associated with an increased expression of a1-subunit of Na+,K+-ATPase, NKCC2, NHE1, and a-subunit of ENaC, suggesting that these sodium transporters should be under tonic inhibitory influence of NO in the submandibular gland. The up-regulation of these transporters may then, at least in part, account for the decreased salivation following inhibition of NO synthesis in rat submandibular gland13, 15), possibly through an enhancement of the ductal reabsorption of sodium.
On the other hand, the expression of AQP5 was significantly decreased by the treatment with L-NAME. This finding suggests that AQP5 is under tonic excitatory influence of NO in the submandibular gland. A key role of AQP5 in saliva fluid secretion has been well known. Transgenic mice lacking AQP5 display reduced pilocarpine-stimulated saliva secretion (60%), in which the secreted saliva is more hypertonic and viscous18). In Sjøgren's syndrome, an abnormal distribution of AQP5 has been demonstrated in the acini, which is likely to contribute to the deficiency of fluid secretion19). A down-regulation of AQP5 may result in a decrease of water flux in the acinar cell and hence the formation of primary saliva. In this context, the L-NAME-induced reduction of secretory responses12, 13, 15) may be attributed in part to the down-regulation of AQP5. The expression of AQP1 has been demonstrated to be continuously distributed to microvasculature during embryonic and postnatal development in the rat submandibular gland20, 21). However, the expression of AQP1 proteins was not significantly altered by the L-NAME-treatment. In transgenic mice lacking AQP1, pilocarpine-induced salivary secretion showed no defects in volume or composition of saliva18, 22). It is unlikely that AQP1 should play a major role in salivary secretion.
Finally, it is worth to note that the systolic blood pressure was significantly increased, indicating that the animals were in a state of systemic withdrawal of tonic influences of endogenous NO. Furthermore, the alteration of sodium transporters and water channels cannot be attributed to the increased blood pressure, perse, since an attenuated salivation has not been documented in other models of hypertension.
In summary, these results indicate that sodium transporters and water channels are under tonic regulatory influence of NO in the submandibular gland. An altered NO synthesis may affect the expression of these transporters and channels, and hence the formation and secretion of saliva. Further studies will be needed to directly measure the sodium and water flux in the ductal epithelia to determine the actual contribution of each transporter.
References
1. Robertson MA, Woodside M, Foskett JK, Orlowski J, Grinstein S. Muscarinic agonists induce phosphorylation-independent activation of the NHE-1 isoform of the Na+/H+ antiporter in salivary acinar cells. J Biol Chem. 1997; 272:287–294. PMID: 8995260.


2. Peagler FD, Redman RS. Enzyme histochemical localization of Na(+),K(+)-ATPase and NADH-DE in the developing rat parotid gland. Anat Rec. 1999; 256:72–77. PMID: 10456987.


3. Robertson MA, Foskett JK. Na+ transport pathways in secretory acinar cells: membrane cross talk mediated by [Cl-]i. Am J Physiol. 1994; 267:C146–C156. PMID: 8048476.


4. Pirani D, Evans LA, Cook DI, Young JA. Intracellular pH in the rat mandibular salivary gland: the role of Na-H and Cl-HCO3 antiports in secretion. Pflugers Arch. 1987; 408:178–184. PMID: 2436139.


5. Case RM, Hunter M, Novak I, Young JA. The anionic basis of fluid secretion by the rabbit mandibular salivary gland. J Physiol. 1984; 349:619–630. PMID: 6737303.


6. Young JA, Cook DI, Evans LA, Pirani D. Effects of ion transport inhibition on rat mandibular gland secretion. J Dent Res. 1987; 66:531–536. PMID: 2442220.


7. Awayda MS, Tousson A, Benos DJ. Regulation of a cloned epithelial Na+ channel by its beta- and gamma-subunits. Am J Physiol. 1997; 273:C1889–C1899. PMID: 9435494.
8. McNicholas CM, Canessa CM. Diversity of channels generated by different combinations of epithelial sodium channel subunits. J Gen Physiol. 1997; 109:681–692. PMID: 9222895.


9. Nielsen S, King LS, Christensen BM, Agre P. Aquaporins in complex tissues. II. Subcellular distribution in respiratory and glandular tissues of rat. Am J Physiol. 1997; 273:C1549–C1561. PMID: 9374640.
10. Koyama Y, Yamamoto T, Tani T, Nihei K, Kondo D, Funaki H, et al. Expression and localization of aquaporins in rat gastrointestinal tract. Am J Physiol. 1999; 276:C621–C627. PMID: 10069989.
11. Delporte C, Steinfeld S. Distribution and roles of aquaporins in salivary glands. Biochim Biophys Acta. 2006; 1758:1061–1070. PMID: 16537077.


12. Tobin G, Edwards AV, Bloom SR, Ekstrom J. Nitric oxide in the control of submandibular gland function in the anaesthetized ferret. Exp Physiol. 1997; 82:825–836. PMID: 9331552.


13. Lomniczi A, Suburo AM, Elverdin JC, Mastronardi CA, Diaz S, Rettori V, et al. Role of nitric oxide in salivary secretion. Neuroimmunomodulation. 1998; 5:226–233. PMID: 9730690.


14. Looms DK, Dissing S, Tritsaris K, Pedersen AM, Nauntofte B. Adrenoceptor-activated nitric oxide synthesis in salivary acinar cells. Adv Dent Res. 2000; 14:62–68. PMID: 11842926.


15. Takai N, Uchihashi K, Higuchi K, Yoshida Y, Yamaguchi M. Localization of neuronal-constitutive nitric oxide synthase and secretory regulation by nitric oxide in the rat submandibular and sublingual glands. Arch Oral Biol. 1999; 44:745–750. PMID: 10471158.


16. Toba H, Nakagawa Y, Miki S, Shimizu T, Yoshimura A, Inoue R, et al. Calcium channel blockades exhibit anti-inflammatory and antioxidative effects by augmentation of endothelial nitric oxide synthase and the inhibition of angiotensin converting enzyme in the N(G)-nitro-L-arginine methyl ester-induced hypertensive rat aorta: vasoprotective effects beyond the blood pressure-lowering effects of amlodipine and manidipine. Hypertens Res. 2005; 28:689–700. PMID: 16392774.


17. De Gennaro Colonna V, Rigamonti A, Fioretti S, Bonomo S, Manfredi B, Ferrario P, et al. Angiotensin-converting enzyme inhibition and angiotensin AT1-receptor antagonism equally improve endothelial vasodilator function in L-NAME-induced hypertensive rats. Eur J Pharmacol. 2005; 516:253–259. PMID: 15963975.


18. Ma T, Song Y, Gillespie A, Carlson EJ, Epstein CJ, Verkman AS. Defective secretion of saliva in transgenic mice lacking aquaporin-5 water channels. J Biol Chem. 1999; 274:20071–20074. PMID: 10400615.


19. Steinfeld S, Cogan E, King LS, Agre P, Kiss R, Delporte C. Abnormal distribution of aquaporin-5 water channel protein in salivary glands from Sjogren's syndrome patients. Lab Invest. 2001; 81:143–148. PMID: 11232635.
20. King LS, Nielsen S, Agre P. Aquaporins in complex tissues. I. Developmental patterns in respiratory and glandular tissues of rat. Am J Physiol. 1997; 273:C1541–C1548. PMID: 9374639.
21. Akamatsu T, Parvin MN, Murdiastuti K, Kosugi-Tanaka C, Yao C, Miki O, et al. Expression and localization of aquaporins, members of the water channel family, during development of the rat submandibular gland. Pflugers Arch. 2003; 446:641–651. PMID: 12838423.


Fig. 1
Expression of endothelial NOS (eNOS) and neuronal NOS (nNOS) in the submandibular gland. Representative immunoblots and densitometric data are shown. The open column denotes control, and the hatched column depicts NG-nitro-L-arginine methyl ester (L-NAME)-treated group. Each column represents mean±SEM of 6 rats.
*p<0.05, ***p<0.001, compared with control.
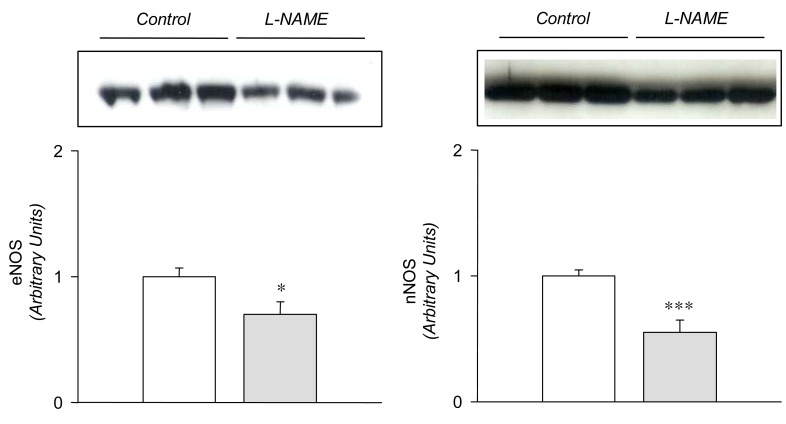
Fig. 2
Expression of β-actin in the submandibular gland. Legends as in Fig. 1. Mean±SEM of 6 rats each.
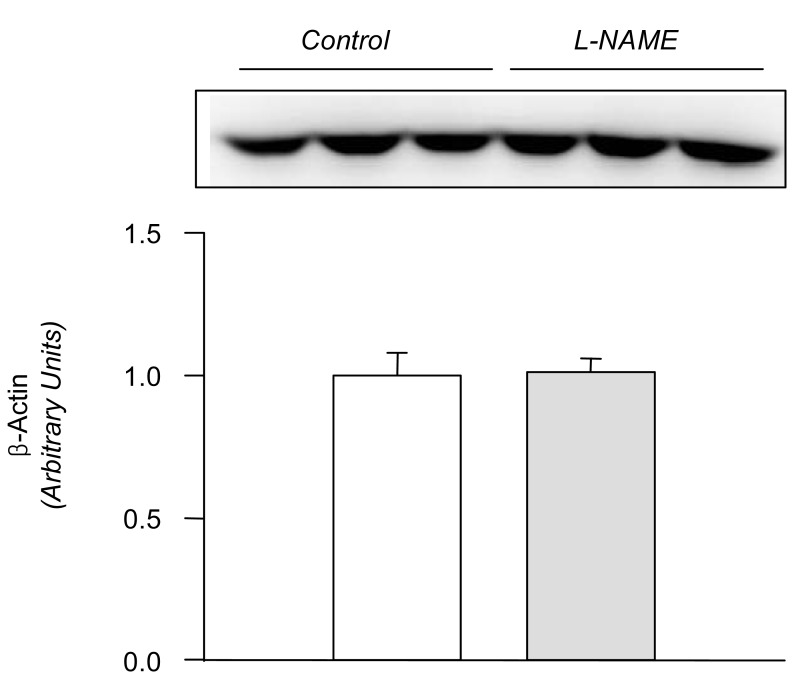
Fig. 3
Expression of α1- and β1-subunits of Na+,K+-ATPase in the submandibular gland. Legends as in Fig. 1. Mean±SEM of 6 rats each.
**p<0.01, compared with control.
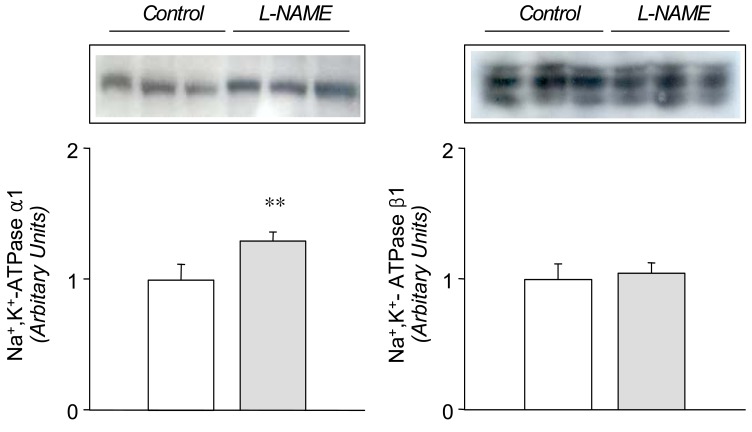
Fig. 4
Expression of Na+/K+/2Cl- cotransporter (NKCC2), Type 1 Na+/H+ exchange (NHE1), and α-subunit of epithelial sodium channel (ENaCα) in the submandibular gland. Legends as in Fig. 1. Mean±SEM of 6 rats each.
*p<0.05, **p<0.01, ***p<0.001, compared with control.
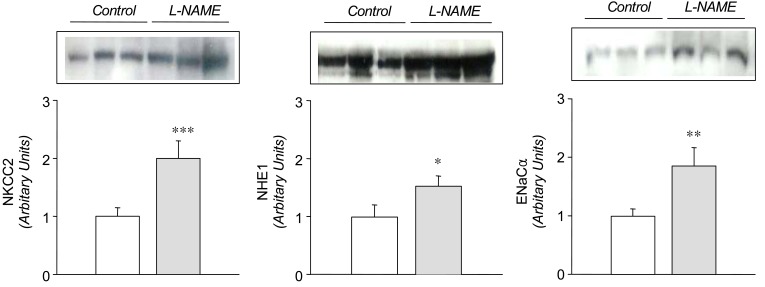
Fig. 5
Expression of aquaporin-1 (AQP1) and aquaporin-5 (AQP5) proteins in the submandibular gland. Legends as in Fig. 1. Mean±SEM of 6 rats each.
*p<0.05, compared with control.
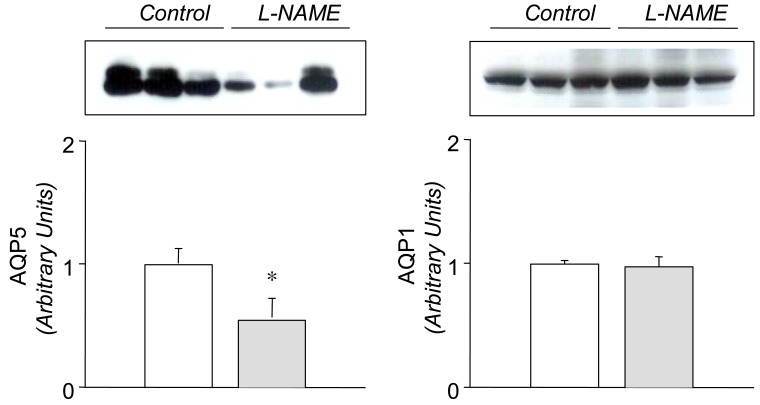