Abstract
Objective
Developmental venous anomalies (DVAs) are benign anatomic variations; therefore, they are usually discovered incidentally. The aim of this article was to describe radiological findings of DVAs.
Methods
A retrospective search for DVAs of the brain was performed in 1899 patients who had undergone magnetic resonance imaging (MRI) with contrast enhancement between January 1, 2005 and April 25, 2011. We also reviewed the results of computed tomography (CT), magnetic resonance angiography (MRA), CT angiography, and transfemoral cerebral angiography (TFCA) studies performed in patients with DVAs.
Results
Thirty-two DVAs were identified in 31 of the 1899 patients (1.63%). These 31 patients underwent five enhanced CTs, three MRAs, two CT angiographies, and two TFCAs. Thirty of the 32 DVAs were supratentorial (ST) and two were infratentorial (IT). All enhanced MRI studies exhibited excellent resolution of DVAs. All DVAs had only one draining vein. The venous drainage system was an IT vein in three DVAs and an ST vein in 29 DVAs. Two out of five enhanced CTs presented good visualization of the draining vein. None of the MRAs, including the source image, revealed the presence of DVAs. The two CT angiographies exhibited good resolution of DVAs. One of the two TFCAs yielded an excellent illustration of the DVA.
Developmental venous anomalies (DVAs), which have been called venous angiomas, are extreme variations of the normal transmedullary veins that are necessary for the drainage of white and gray matter.3) DVAs serve as normal drainage routes of the brain tissue. Though most DVAs are discovered fortuitously and bear no clinical significance, their diagnosis often generates concern among physicians who are less familiar with the field of intracranial vascular malformations. Moreover, their management requires thorough understanding of the nature of DVAs, including their frequent coexistence with other types of vascular malformations and the existence of more complex but rare forms of this clinical presentation, such as arterialized DVAs.9)
The aim of this article was to describe, using a retrospective series of cases, radiological findings of DVAs. In addition, we analyzed magnetic resonance imaging (MRI), computed tomography (CT) angiography, transfemoral cerebral angiography (TFCA), and magnetic resonance angiography (MRA) findings of DVAs and reviewed the clinical significance of DVAs.
A retrospective search for brain DVAs was performed in 1,899 patients who had undergone MRI studies with contrast enhancement between January 1, 2005 and April 25, 2011. A few patients underwent MRI as a routine checkup. However, in most cases, the MRI was acquired for a variety of clinical reasons, including symptoms of cerebral ischemia, Parkinson's disease, hemorrhagic contusion, intracranial hemorrhage, headache, psychiatric disorder, tinnitus, aneurysm, brain tumor and dizziness.
For the purposes of this study, typical DVAs were considered present on MR study if the following criteria were met: presence on contrast-enhanced MRI of star-like, tubular vessels that converged in a collector vein, which, in turn, drained into a dural sinus or ependymal vein. The cluster of veins in DVAs had a spoke-wheel appearance (the so-called caput medusae).
All MRIs (Intera Achieva, Philips Medical Systems, Eindhoven, Netherlands) were performed using 1.5 T magnets. T2-weighted images were acquired using a conventional spin echo technique with repetition time (TR) ranging from 4670 to 4240 ms and an echo time (TE) of 100 ms. For T1-weighted spin echo images, TR ranged from 653 to 450 ms and TE was 12 ms. TR and TE for fluid-attenuated inversion-recovery (FLAIR) images were 11,000 ms and 140 ms, respectively. The acquisition parameters used were as follows: field of view, 220 × 220 or 230 × 230 mm; matrix, 240 × 240 or 256 × 256; and slice thickness/gap, 5 mm/1 mm. T1-weighted contrast-enhanced images were obtained after administration of 15 mL of Magnevist (Bayer Schering Pharma AG, Berlin, Germany) via an antecubital vein over 10 s.
For the MRA studies, a three-dimensional time-of-flight (TOF) technique was used with a neurovascular phased array coil (SENSE-Head-8, Philips Medical System). A multiple-overlapping thin-slab acquisition technique was used. The following imaging parameters were selected : repetition time, 23 ms; echo time, 6.9 ms; field of view, 180 × 180 mm; number of slices, 120; slice thickness, 0.7 mm; slab thickness, 84 mm; imaging matrix, 384 × 512; number of excitations, 1. No intravenous paramagnetic contrast agent was administered to any of the patients. In each patient, a total of 20 maximum-intensity-projection images in the frontal view (both from left lateral to right lateral 180° and craniocaudally 180°) were routinely displayed stereoscopically.
Enhanced CTs were obtained using a Toshiba CT scanner (Toshiba Aquilion TSX-101A, 64 Channels; Toshiba Medical Systems, Tokyo, Japan) after administration of 100 mL of Iobrix 350 (Iohexol, Taejoon Pharmaceuticals Co. Ltd, Seoul, Korea) via automated antecubital venous infusion. The contrast infusion rate was 2.0 mL/s, and section thickness and section interval were 5 mm with no interval, and the field of view was 220 × 220 mm.
CT angiography was performed using a Toshiba CT scanner. The following imaging parameters were selected: CT configuration, 64 × 0.5 mm; contrast amount and delivery route, 100 mL of Iobrix 350 via automated antecubital venous infusion; contrast infusion rate, 4.0 mL/s; reconstructed section thickness and section interval, 5 mm with no interval; CT matrix, 512 × 512; 120 kVp; 100 mA; field of view, 64 × 64 mm.
TFCAs were performed using a Philips V3000 apparatus (Philips V3000, Philips Medical Systems, Eindhoven, Netherlands).
We evaluated the radiological findings and clinical symptoms of DVAs.
Thirty-two DVAs were identified in 31 out of 1899 patients (1.63%; 15 male and 16 female patients). All 32 DVAs were found incidentally. These 31 patients underwent five enhanced CTs, three MRAs, two CT angiographies and two TFCAs.
Thirty-one enhanced MRI studies exhibited good resolution of DVAs. T1- or T2-weighted images showed only the collector vein in some patients. Moreover, T1- or T2-weighted images did not provide a definitive diagnosis of DVA (Fig. 1). Four patients with DVAs underwent follow-up MRI at intervals ranging from 17 to 38 months. Follow-up MRI showed no changes in DVAs. None of the MRA studies, including the source image, revealed the presence of DVAs. Two out of five enhanced CTs presented good visualization of the draining vein. The two CT angiographies exhibited good resolution of DVAs (Fig. 2). One of the two TFCAs revealed the presence of a DVA with supratentorial (ST) superficial venous drainage (Fig. 3).
The DVAs were ST in 30 cases and infratentorial (IT) in two cases. The location of the DVAs was frontal in 18 cases, temporal in two cases, parietal in four cases, occipital in one case, temporoparietal in one case, in the basal ganglia in two cases, in the limbic lobe in two cases and cerebellar in two cases. All DVAs had only one draining vein. The venous drainage system was an IT vein in three DVAs and an ST vein in 29 DVAs. Among the three posterior fossa draining veins of DVAs, one was a lateral mesencephalic vein and two were posterior fossa superficial veins. Two posterior fossa superficial veins were drained to the superior petrosal sinus and tentorial sinus, respectively. Among the 29 ST venous draining veins of DVAs, 21 were superficial ST veins and eight were deep ST veins.
Associated pathologies included one cavernous malformation (CM) located adjacent to the draining vein of DVAs, two meningiomas and one arteriovenous malformation.
DVAs are rarely identified on CT without contrast enhancement, unless they are associated with a CM. On contrast-enhanced CT, the venous collector of the DVA is readily detectable as a linear or curvilinear focus of enhancement, typically coursing from the deep white matter to a cortical or a deep vein or to the dural sinus.6) However, the CT technique has limitations because of the presence of streak artifacts in cases of DVAs located in the posterior fossa. In our study, two out of five enhanced CTs presented good visualization of the draining vein but none of the enhanced CTs confirmed the diagnosis of DVA.
Currently used protocols for CT angiography allows the inclusion of venous structures in the data, which may lead to inaccurate interpretation of these studies. Therefore thorough knowledge of variant venous anatomy is necessary to avoid misinterpretation of normal veins as DVAs.5) However, this characteristic of CT angiography may identify DVAs. In our study, both CT angiographies revealed the presence of DVAs including the draining vein (Fig. 2).
In our study, none of the MRAs and source images identified DVAs.
On MRI, DVAs typically have a transhemispheric flow void, on both T1- and T2-weighted images. Noncontrast T1- and T2-weighted MRI may demonstrate flow voids and phase-shift artifacts produced by the collecting vein of a DVA.4) T1-weighted studies may yield normal results in the presence of small DVAs. The collector vein is detected as a linear or small, round, signal-void structure on all sequences and is shown most clearly on T2-weighted imaging. Similarly to CMs, low flow and low resistance almost always typify DVA hemodynamics. After the administration of gadolinium, significant enhancement of the medullary veins and venous collector is observed because of the slow flow.6) On contrast-enhanced MRI, the cluster of veins in DVAs has a spoke-wheel appearance; the veins are small at the periphery and gradually enlarge as they approach a central draining vein.1) This appearance has been referred to as caput medusae (or the head of Medusa). In our study, all 32 DVAs were diagnosed using enhanced MRI without the assistance of other imaging techniques. We identified the detailed characteristics of DVAs using enhanced MRI. Contrast-enhanced MRI was the sequence of choice for depiction of DVAs. A stellate configuration around an emanating transcortical vein is a typical image of DVA.10) However, in our study, T1- and T2-weighted images revealed only the collector vein in some cases. An angiographic appearance of a DVA architecture that is stable over long periods is the rule.2) In our study, MRI with enhancement on follow-up in four cases demonstrated no changes in DVAs.
The classical angiographic appearance of caput medusae for transmedullary veins is visualized during the early-to-middle venous phase, draining into a large venous collector, which can extend either to the superficial or deep venous system depending on the type of DVA.6) In most cases with DVA, angiographic studies illustrate the underdevelopment of the normal venous drainage pattern adjacent to a DVA.7) It is hypothesized that this results from a focal arrest of venous development and retention of primitive medullary veins that drain into a single, large draining vein. Surgical excision of DVA has led to disastrous postoperative complications resulting from venous infarction and cerebral edema, because the brain was deprived of functionally normal venous drainage conduit. In our study, we found a good illustration of a DVA in one out of two TFCAs. However, we did not identify underdevelopment of the normal venous drainage pattern adjacent to DVA. The other TFCA did not provide an exact diagnosis of the DVA because of coexistent arteriovenous malformation and concomitant large number of venous structures.
Most patients who harbor DVA have no symptoms; the DVA is found incidentally during a neuroimaging investigation. DVAs are usually diagnosed in young adults who present with various symptoms that often are not related to the DVA per se.
The reported high incidence of intracranial hemorrhage associated with DVAs in previous studies is currently attributed to the coexistence of a CM.7) Any DVA that presents with symptoms and signs that can be directly attributed to the DVA merits vigorous investigation using high-field MRI and TFCA, to exclude the possibility of a coexisting CM or of an arteriovenous malformation. On MRI, a typical CM appears as a well-circumscribed lesion of mixed signal intensity on T1- and T2-weighted sequences, with variable enhancement after contrast administration, a hypointense rim corresponding to hemosiderin deposition, and a characteristic "blooming effect" resulting from the susceptibility dephasing caused by hemosiderin.8) In our study, we identified one CM adjacent to the draining vein of a DVA using T1- and T2-weighted images and FLAIR images (Fig. 1).
Conservative management of isolated DVAs is warranted. Neither neurosurgical intervention nor stereotactic radiosurgery is adequate for the treatment of isolated DVAs, because these practices can lead to unacceptable morbidity.7)
Although DVAs should be considered benign, under rare circumstances they can be symptomatic. Pereira et al.6) reported a review of all possible pathomechanisms of symptomatic DVAs, which were divided by these authors into mechanical and flow-related causes. Mechanical (obstructive or compressive) pathomechanisms accounted for 14 out of 69 symptomatic patients, resulting in hydrocephalus or nerve-compression syndromes. Flow-related pathomechanisms (49 out of 69 patients) were subdivided into complications resulting from an increase of flow into the DVA (due to an arteriovenous shunt using the DVA as the drainage route; n = 19), a decrease of outflow (n = 26), or a remote shunt with increased venous pressure (n = 4) leading to symptoms of venous congestion.6)
Accurate surgical planning during dissection of coexisting lesions should focus on sparing the DVA. In the rare cases, in which isolated symptomatic DVAs cause hemorrhage or thrombosis, surgical treatment should target not DVA but resultant complication (e.g., surgical excision of intracerebral hemorrhage with mass effect, decompression with extensive infarction or edema, and cerebrospinal fluid diversion with ventricular outflow obstruction). Anticoagulation therapy may be an option in the uncommon event of DVA thrombosis and ischemic complications.7)
CT angiography and MRI with contrast enhancement provided detailed findings of DVAs. However, MRA did not show DVAs. Enhanced CT identified only the collecting vein of DVAs in some cases. DVAs were located mainly in the ST space and the main venous drainage system of ST DVAs was a superficial vein.
Figures and Tables
Fig. 1
A 26-year-old man presented with cerebral concussion. T1-weighted axial image (A) and T2-weighted axial image (B) show a draining vein (A, arrow) and a cavernous malformation (B, arrow) located in the left frontal lobe, respectively. T1-weighted images after contrast enhancement (C: axial, D: sagittal, E: coronal) indicate a cavernous malformation (C, arrow), a draining vein (D, arrow) into the ependymal vein and a developmental venous anomaly (E, arrow).
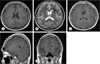
Fig. 2
A 29-year-old woman presented with seizure. T1-enhanced images after contrast enhancement (A: axial; B: coronal) show a draining vein (A and B, arrow) located in the right frontal lobe. T2-weighted image (C) reveals a draining vein (arrow) in the right frontal lobe. Enhanced computed tomography (D) shows a draining vein (arrow) located in the right frontal lobe. Computed tomography angiography (E) reveals a developmental venous anomaly (arrow) located in the right frontal lobe.
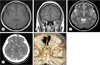
Fig. 3
A 54-year-old male underwent magnetic resonance imaging (MRI) as a routine check-up. T1-weighted images after contrast enhancement (A: axial image; B: sagittal image) show a developmental venous anomaly (DVA) (A and B, arrow) located in the right parietal lobe. Transfemoral cerebral angiography (C) reveals a DVA (arrow) with superficial draining vein into the superior sagittal sinus.
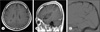
Acknowledgements
This work was supported by research grant from an Inje University College of Medicine. This study was presented in 25th annual meeting of The Korean Society of Cerebrovascular Surgeons at Jeju, Korea.
References
1. Augustyn GT, Scott JA, Olson E, Gilmor RL, Edwards MK. Cerebral venous angiomas: MR imaging. Radiology. 1985. 08. 156(2):391–395.


2. Hashimoto M, Yokota A, Kajiwara H, Matsuoka S, Tsukamoto Y. Venous angioma: follow-up study and therapeutic considerations. Neurol Med Chir (Tokyo). 1990. 08. 30(8):599–603.


3. Lasjaunias P, Burrows P, Planet C. Developmental venous anomalies (DVA): the so-called venous angioma. Neurosurg Rev. 1986. 9(3):233–242.


4. Lee C, Pennington MA, Kenney CM 3rd. MR evaluation of developmental venous anomalies: medullary venous anatomy of venous angiomas. AJNR Am J Neuroradiol. 1996. 01. 17(1):61–70.
5. Peebles TR, Vieco PT. Intracranial developmental venous anomalies: diagnosis using CT angiography. J Comput Assist Tomogr. 1997. Jul-Aug. 21(4):582–586.
6. Pereira VM, Geibprasert S, Krings T, Aurboonyawat T, Ozanne A, Toulgoat F, et al. Pathomechanisms of symptomatic developmental venous anomalies. Stroke. 2008. 12. 39(12):3201–3215.


7. Rammos SK, Maina R, Lanzino G. Developmental venous anomalies: current concepts and implications for management. Neurosurgery. 2009. 07. 65(1):20–29. discussion 29-30.
8. Ruiz DS, Yilmaz H, Gailloud P. Cerebral developmental venous anomalies: current concepts. Ann Neurol. 2009. 09. 66(3):271–283.
9. San Millán Ruíz D, Gailloud P. Cerebral developmental venous anomalies. Childs Nerv Syst. 2010. 10. 26(10):1395–1406.


10. Toro VE, Geyer CA, Sherman JL, Parisi JE, Brantley MJ. Cerebral venous angiomas: MR findings. J Comput Assist Tomogr. 1988. 12(6):935–940.