Abstract
Purpose
To evaluate the effect of different numbers of basis images and the use of metal artifact reduction (MAR) on the production and reduction of artifacts in cone-beam computed tomography images.
Materials and Methods
An acrylic resin phantom with a metal alloy sample was scanned, with 450 or 720 basis images and with or without MAR. Standard deviation values for the test areas (around the metal object) were obtained as a way of measuring artifact production. Two-way analysis of variance was used with a 5% significance level.
Results
There was no significant difference in artifact production among the images obtained with different numbers of basis images without MAR (P=.985). MAR significantly reduced artifact production in the test areas only in the protocol using 720 basis images (P=.017). The protocol using 450 basis images with MAR showed no significant difference in artifact production when compared to the protocol using 720 basis images with MAR (P=.579).
Cone-beam computed tomography (CBCT) images have been increasingly used for diagnosis in dentistry.1 However, the presence of high-density and high-atomicnumber materials results in the generation of artifacts, which may cause more time to be needed to evaluate the images and even compromise the diagnosis in areas where artifacts are observed.234
In order to minimize artifacts and achieve better quality in CBCT images, the metal artifact reduction (MAR) tool was developed. This tool is an algorithm applied in tomographic image reconstruction that minimizes or, if possible, eliminates image artifacts. Some companies, such as Vatech (Hwaseong, Korea), Planmeca (Helsinki, Finland), and Soredex (Tuusula, Finland), make these tools commercially available in some CBCT equipment. However, some studies have shown that the MAR tool was not effective for periodontal and peri-implant defects5 or even that the MAR tool led to decreased diagnostic accuracy for root fractures.67 Thus, it remains necessary to study the factors that could result in improved image quality in terms of image artifacts.
CBCT image acquisition is done by acquiring multiple basis images. The number of basis images can be determined in commercially available devices through the rotation arc and/or by the scanning time. The more basis images are obtained, the more information is available for image reconstruction, which could result in fewer artifacts. However, the more basis images are obtained, the more time is required for scanning and reconstruction, and more importantly, the higher the radiation dose is for the patient.8
Considering the possibilities of using the MAR tool and acquiring volumes with a different number of basis images, features that have already been studied separately with the goal of achieving better CBCT image quality, it is important to study whether the combination of these factors can significantly increase tomographic image quality. Therefore, this study was designed to evaluate the effects of different numbers of basis images and the use of the MAR tool on the production and reduction of artifacts in CBCT images.
A chemically activated acrylic resin phantom (VIPI, São Paulo, Brazil) was constructed (98 mm diameter×40 mm height), containing a cylindrical sample (5.4 mm diameter× 5.4 mm height) made of an aluminum-bronze (Cu-Al) alloy (Dental Gaúcho - Marquart & Cia. Ltda., Barueri, Brazil) (Fig. 1).
A Picasso Trio CBCT scanner (Vatech, Hwaseong, Republic of Korea) was used to acquire the images. For scanning, the metal alloy cylinder was centered in a field of view measuring 50 mm×50 mm, and the parameters used were a voxel size of 0.2 mm, 80 kVp, and 3.7 mA.
The apparatus used has exposure times of 15 seconds, which results in 450 basis images, and of 24 seconds, resulting in 720 basis images; additionally, the MAR tool can be activated for any protocol. The phantom was scanned using 4 different acquisition protocols (Table 1), considering these parameters.
One observer evaluated all acquired images (Fig. 2) using OnDemand3D software (CyberMed, Seoul, Korea). The midpoint of the sample was determined in the coronal view and, from that, the corresponding axial slice of the central area of the metal alloy sample was obtained. Using the histogram tool, 6 circular regions of interest (5.4 mm in diameter) were placed around the metal alloy image (test areas) (Fig. 3). Standard deviations (SDs) of the gray values were obtained as a method to measure artifact production.9
Data homogeneity and variance were analyzed by the Kolmogorov-Smirnov and Bartlett tests, respectively. Two-way analysis of variance was used to evaluate the influence of the number of basis images and MAR on artifact production. The significance level was set at 5%. All data analysis was done using GraphPad Prism 7 software (GraphPad Software, La Jolla, CA, United States).
Table 2 shows the mean value of the SDs of the gray values (artifact production) for the metal area. Regarding the number of basis images, no significant difference (P=.985) was observed in artifact production for images obtained with 450 or 720 basis images.
In the images obtained with 720 basis images, significantly less (P=.017) artifact production was seen when the MAR tool was used. For protocols with 450 basis images, no significant difference was observed depending on whether the MAR tool was used (P=.365). However, when the MAR tool was active, there was no significant difference (P=.579) in artifact production in images obtained with different numbers of basis images.
It is important to note that no statistically significant differences were observed when the protocol using 450 basis images with MAR was compared with the protocol using 720 basis images, with (P=.5795) or without (P=.2174) MAR.
The protocol using 720 basis images with the MAR tool showed better results due to the lower artifact production, but no statistically significant difference was observed (P=.2174) in comparison with the protocol using 450 basis images with the MAR tool.
The more basis images are obtained, the more information is available for image reconstruction, which, according to Scarfe and Farman,8 results in fewer image artifacts. In the present study, this association between a greater number of basis images and fewer image artifacts was not observed. Instead, it was found that a greater number of basis images did not significantly reduce the amount of image artifacts, which agrees with the results found by Bechara et al.,6 who reported that no diagnostic improvement was observed when high-density materials were scanned with a greater number of basis images. Thus, it is possible to infer that when a higher number of basis images is selected in the presence of a metallic material, although there is an increase in the amount of information for image reconstruction, there is also an increased amount of image noise.
Increasing the number of basis images leads to a longer scanning time, which results in a higher radiation dose compared to an acquisition with the same exposure parameters but a lower number of basis images, and consequently, a shorter scanning time. Additionally, a longer scanning time increases the probability of generating another type of artifact that is especially relevant in clinical practice: the movement artifact.10 The presence of a movement artifact may render the image unusable for diagnosis, meaning that the patient must be exposed again to acquire a new volume.
In addition, obtaining more basis images increases the working time, because in addition to the greater scanning time, more time is needed for reconstructing the images. However, in this study, no improvement in image quality occurred; we found no benefits that would justify the longer working time. Thus, it does not seem reasonable to increase the number of basis images alone.
Therefore, an alternative for artifact reduction without increasing the radiation dose should be pursued. MAR was evaluated in this study as a possible way to accomplish this goal.
Considering only the number of basis images, in this in vitro study, the MAR tool was effective when CBCT images were acquired with 720 images. This may have been due to the greater amount of information available in this protocol, which could have resulted in a better thresholding accuracy, improving the effectiveness of this tool. Nonetheless, it is important to keep in mind that, in the present study, the acquisition protocol using 450 basis images with the MAR tool resulted in images with a similar quality, as measured by the amount of noise, to that of the images obtained with a greater number of basis images, with or without the MAR tool.
The acquisition time required for volumes with fewer basis images is shorter than is needed for volumes with more basis images, meaning that in clinical situations, the patient would be exposed to a lower radiation dose. The MAR tool increases the reconstruction time. As such, if the volume is acquired with 720 basis images and the tool is activated, there will be an increase in both the required clinical time and the radiation dose to which the patient is exposed, without no corresponding improvement in the amount of image noise.
However, the MAR tool itself does not increase the radiation dose to which the patient is exposed. Thus, by using fewer basis images, the acquisition time will be shorter, compensating for the longer reconstruction time when the tool is activated. In such a clinical situation, the patient would be exposed to a lower dose of radiation than if the volume is acquired with 720 basis images.
It is important to consider that in vitro studies, such as this study, allow variables to be precisely controlled and enable specific factors to be isolated. Although they do not precisely reproduce the variation inherent in clinical practice, in vitro studies are important as a reference point for future clinical studies.
In conclusion, in this in vitro setting, in the presence of an artifact-generating metal object, it was preferable for CBCT scans to be acquired with fewer basis images and with MAR activated to minimize artifact production. This would be clinically beneficial because such a protocol would avoid exposing patients to unnecessary radiation.
Figures and Tables
Fig. 2
Examples of axial images obtained with different numbers of basis images, with and without the metal artifact reduction (MAR) tool, of the metal test areas. A. 450 basis images without MAR. B. 450 basis images with MAR. C. 720 basis images without MAR. D. 720 basis images with MAR. MAR, metal artifact reduction.
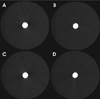
Fig. 3
Six circular regions of interest around the metal sample were used to evaluate artifact production.
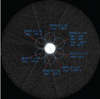
References
1. Scarfe WC, Farman AG, Sukovic P. Clinical applications of cone-beam computed tomography in dental practice. J Can Dent Assoc. 2006; 72:75–80.
2. Vande Berg B, Malghem J, Maldague B, Lecouvet F. Multi-detector CT imaging in the postoperative orthopedic patient with metal hardware. Eur J Radiol. 2006; 60:470–479.


3. Draenert FG, Coppenrath E, Herzog P, Müller S, Mueller-Lisse UG. Beam hardening artefacts occur in dental implant scans with the NewTom cone beam CT but not with the dental 4-row multidetector CT. Dentomaxillofac Radiol. 2007; 36:198–203.
4. Schulze R, Heil U, Gross D, Bruellmann DD, Dranischnikow E, Schwanecke U, et al. Artefacts in CBCT: a review. Dentomaxillofac Radiol. 2011; 40:265–273.


5. Kamburoğlu K, Kolsuz E, Murat S, Eren H, Yüksel S, Paksoy CS. Assessment of buccal marginal alveolar peri-implant and periodontal defects using a cone beam CT system with and without the application of metal artefact reduction mode. Dentomaxillofac Radiol. 2013; 42:20130176.


6. Bechara B, McMahan CA, Nasseh I, Geha H, Hayek E, Khawam G, et al. Number of basis images effect on detection of root fractures in endodontically treated teeth using a cone beam computed tomography machine: an in vitro study. Oral Surg Oral Med Oral Pathol Oral Radiol. 2013; 115:676–681.


7. Bezerra IS, Neves FS, Vasconcelos TV, Ambrosano GM, Freitas DQ. Influence of the artefact reduction algorithm of Picasso Trio CBCT system on the diagnosis of vertical root fractures in teeth with metal posts. Dentomaxillofac Radiol. 2015; 44:20140428.


8. Scarfe WC, Farman AG. What is cone-beam CT and how does it work? Dent Clin North Am. 2008; 52:707–730.

