Abstract
Purpose
Erosions and osteophytes are radiographic characteristics that are found in different stages of temporomandibular joint (TMJ) osteoarthritis. This study assessed the effectiveness of digital subtraction radiography (DSR) in diagnosing simulated osteophytes and erosions in the TMJ.
Materials and Methods
Five intact, dry human skulls were used to assess the effectiveness of DSR in detecting osteophytes. Four cortical bone chips of varying thicknesses (0.5 mm, 1.0 mm, 1.5 mm, and 2.0 mm) were placed at the medial, central, and lateral aspects of the condyle anterior surface. Two defects of varying depth (1.0 mm and 1.5 mm) were created on the lateral, central, and medial poles of the condyles of 2 skulls to simulate erosions. Panoramic images of the condyles were acquired before and after artificially creating the changes. Digital subtraction was performed with Emago dental image archiving software. Five observers familiar with the interpretation of TMJ radiographs evaluated the images. Receiver operating characteristic (ROC) analysis was used to evaluate the diagnostic accuracy of the imaging methods.
Results
The area under the ROC curve (Az) value for the overall diagnostic accuracy of DSR in detecting osteophytic changes was 0.931. The Az value for the overall diagnostic accuracy of panoramic imaging was 0.695. The accuracy of DSR in detecting erosive changes was 0.854 and 0.696 for panoramic imaging. DSR was remarkably more accurate than panoramic imaging in detecting simulated osteophytic and erosive changes.
Radiographic evaluations play an important role in diagnosing temporomandibular joint (TMJ) conditions.1 It has been confirmed that a clinical examination alone is not reliable for diagnosing TMJ disorders (TMD).2 Radiographic evaluations are used to detect osseous abnormalities, to estimate the severity of abnormalities, and to assess the condyle-fossa relationship.1 Loss of articular cortication, erosion, sclerosis, flattening of the articular surface, and osteophyte formation are the most common osseous features of degenerative arthritis of the TMJ.34 Furthermore, articular surface erosion and osteophytes are radiographic characteristics that are found in different stages of TMJ osteoarthritis.5
Cortical erosion is seen in the early stages of degeneration. This suggests that the TMJ is unstable and that the modification of osseous joint surfaces may be taking place, which may lead to occlusal changes. Erosions are seen as areas of decreased density on the osseous surface with interruption and non-uniformity of the cortical outline. In contrast, osteophytes present in later stages of degeneration to stabilize and broaden the surface of the joint in order to better resist loading forces. This indicates that the body is adapting to repair the TMJ. Osteophytes are seen as areas of new cartilage and bone formation, and, radiographically, they present as marginal bony outgrowths.5
A superimposition-free image of the TMJ and the surrounding structures is necessary for accurate diagnosis and proper treatment planning in TMD patients.6 Revesz et al.7 reported that the detectability of a radiographic feature depends on its own characteristics and the structures surrounding it. The authors also stated that the visibility of a lesion is directly proportional to its own contrast and is inversely proportional to the background noise. Therefore, lesion detection can be improved by reducing the background noise.
Currently, the techniques used to evaluate bony changes in the TMJ include panoramic imaging, panoramic TMJ radiography, conventional tomography, computed tomography (CT), and cone-beam computed tomography (CBCT). Panoramic images show the right and left mandibular condyles in open and closed positions in a single image. These images contain superimposition of the skull base and zygomatic arches over the TMJ, making it difficult to detect the extent of the disease. Moreover, the anatomic relations of the TMJ bony structures are not seen properly on panoramic images. However, these images are considered suitable for an initial diagnosis.68
In order for an alteration to become visible on a conventional radiograph, approximately 30%-50% of bone mineral content must be lost.9 New techniques that may increase the sensitivity of detecting small bone mineral changes without reducing the specificity have been investigated. Digital subtraction radiography (DSR) is a technique that decreases the structural noise by eliminating identical image features in serially acquired radiographs.10 DSR hides the background features, reduces the background complexity, compresses the dynamic range, and enhances small differences by superimposing images obtained serially at different times.1011
Existing guidelines proposed by the Research Diagnostic Criteria for Temporomandibular Disorders have conventionally suggested CT as the modality of choice for the assessment of osseous changes of the TMJ.3 Since it involves lower doses of radiation, however, CBCT is preferred over CT, and it is now the modality of choice for evaluating osseous structures of the TMJ.112 Yet, these techniques expose patients to more radiation than panoramic radiography.8 On the contrary, DSR acquisition requires only 2 panoramic radiography exposures, which leads to considerably lower doses of radiation. This advantage plays an important role in reducing exposure doses, particularly in young patients.
Multiple imaging systems have been used to detect osteophytes and erosions, but with marginal results.5 DSR may provide the clinician with a more effective technique to detect such changes with a considerably lower dose of radiation.
The aim of this study was to assess the effectiveness of DSR in diagnosing simulated osteophytes and erosions in the TMJ.
Five intact, dry human skulls with no obvious evidence of degenerative joint pathology were selected and used to assess the effectiveness of DSR in detecting osteophytes. Four different cortical bone chips of varying thicknesses (0.5 mm, 1.0 mm, 1.5 mm, and 2.0 mm) were created from the cortical bone of a dry human mandible. All 4 bone chips were pyramidal in shape and had a base of roughly 3 mm×3 mm with specific heights of 0.5 mm, 1.0 mm, 1.5 mm, and 2.0 mm. Each of the chips was placed at the medial, central, and lateral aspects of the condyle anterior surface to mimic osteophytes (Fig. 1A).
Two skulls with no obvious evidence of degenerative joint pathology were used for the evaluation of erosive change. Two round defects measuring 1.0 mm and 1.5 mm in diameter were created on the lateral, central, and medial poles of the superior aspect of the condyle to mimic erosions (Fig. 1B).
Mandibular condyles were stabilized in a fixed position relative to the glenoid fossa and the articular eminence with a removable silicone-based impression material. The skulls were fixed onto an adjustable tripod apparatus for positioning in the panoramic radiography machine (Fig. 1C).
Panoramic images of every condyle were acquired before creating each of the artificial changes and were used as reference images to eliminate the effect of previous changes in the resulting image. After creating artificial bone changes, second images were obtained. Bone changes were not created simultaneously in the same condyle. Only one change was made and imaged at a time. The image subsequent to the first defect served as a base image for the second defect, since the image of the first defect would be eliminated by DSR, and so on. Thus, an even number of images with and without bone changes were used in the study. Panoramic images were acquired using a digital panoramic machine (Morita Veraviewepocs, J. Morita Mfg. Corp., Kyoto, Japan). The exposure parameters were 60 kVp, 2 mA, and 14 seconds, and these settings were maintained throughout the study. Forty-eight images per skull for the osteophytes were acquired, including 24 images without bone chips and 24 images with bone chips, for a total of 240 images from the 5 skulls. Twenty-four images per skull were obtained for the erosions: 12 images without erosions and 12 images with erosions, for a total of 48 images from 2 skulls. The contrast of the images was adjusted and standardized using histogram features within the proprietary software of the panoramic machine. Panoramic images were then exported and saved in the bitmap format.
DSR images were created from the panoramic images using the Emago dental image archiving software (Oral Diagnostic Systems, Amsterdam, The Netherlands). All image manipulation procedures, including image acquisition, storage, alignment, and gamma-corrected digital subtraction, were made with this software. The program allowed the operator to choose various reference points on the radiographs in order to achieve an optimal match between 2 panoramic images for the DSR image. DSR was obtained by subtracting the reference image from the subsequent image. Changes associated with bone gain appeared brighter than the background (Fig. 2), and changes associated with bone loss appeared darker than the background (Fig. 3).
Five observers (2 oral and maxillofacial radiologists, 2 medical radiologists, and 1 oral and maxillofacial surgeon) who were familiar with the interpretation of TMJ radiographs evaluated the images independently. Before each evaluation session, observers were given verbal and written instructions. Sample images of each technique were also shown to observers before each evaluation session as a calibration session.
DSR images were randomized and displayed under dim lighting using a 20.1-inch Digital Imaging in Communications and Medicine monitor (Dome GX2MP, NDS Surgical Imaging, LLC, San Jose, USA), a 1600×1200 resolution, a 10-bit gray-scale, and a 24-bit color capability. In order to standardize the image quality, observers were not allowed to modify the contrast or brightness of images but were able to use the default magnification option in the Windows 7 Home Basic operating system.
All 5 observers evaluated each of the 2 techniques blindly in separate sessions and rated their level of confidence with respect to the presence or absence of the simulated bony pathosis. In a third viewing session, each observer reevaluated images from 1 of the 2 techniques, chosen randomly, to assess intraobserver reliability. No time limit was set for image evaluation. Observers assessed the images on a 5-point scale, with 1 representing lesions definitely not being present and 5 representing lesions definitely being present.
All statistical analyses were performed using NCSS 2004 (NCSS LLC, Kaysville UT, USA). Receiver operating characteristic (ROC) analysis was used to evaluate the diagnostic accuracy of both imaging techniques. The relationship between techniques and bony changes was assessed using the chi-square test. Intraobserver and interobserver agreement were determined by kappa (κ) tests. P<.05 was considered to indicate statistically significance in all analyses.
In the assessment of osteophytes, near-perfect intraobserver agreement was found (κ=0.89, P<.05). Perfect interobserver reliability was also found for panoramic imaging and DSR, with κ values of 0.96 and 0.95, respectively (P<.05). The area under the ROC curve (Az) for the overall diagnostic accuracy of detecting osteophytes was 0.931 for DSR and 0.695 for panoramic imaging. DSR was remarkably more accurate than panoramic imaging in detecting simulated osteophytic changes (P<.05). Table 1 shows the pooled Az values for all observers across all locations and lesion sizes for panoramic imaging and DSR. Figure 4A shows the ROC curves for the 2 modalities.
Table 2 shows chi-square test results by technique and lesion size. For data analysis, the 0.5-mm and 1.0-mm bone chips were classified as small osteophytes, and the 1.5-mm and 2.0-mm bone chips were classified as large osteophytes. There was a significant difference between panoramic imaging and DSR (P<.05). In the large osteophyte group, 63.3% of osteophytes were detected by panoramic imaging, and in the small osteophyte group, 11.6% of osteophytes were detected by panoramic imaging. Furthermore, 100% of osteophytes were detected by DSR in the large osteophyte group, while 71% of osteophytes were detected by DSR in the small osteophyte group. Osteophytes greater than 1 mm could be easily seen via DSR.
Table 3 shows chi-square test results by technique and osteophyte location. In panoramic imaging, osteophytes in the lateral location were detected with significantly higher (P<.05) diagnostic accuracy than at the medial and central locations. There was no significant difference among locations in DSR (P>.05).
Near-perfect interobserver agreement was seen in the evaluation of erosions (κ=0.85, P<.05). Perfect interobserver reliability was also found for panoramic imaging and DSR, with κ values of 0.95 and 0.93, respectively (P<.05). The Az for the overall diagnostic accuracy of detecting erosions was 0.854 for DSR and 0.696 for panoramic imaging (Table 4). A significant difference was seen between panoramic imaging and DSR in detecting erosive changes (P<.05). Figure 4B shows the ROC curves for the 2 modalities.
Table 5 shows chi-square test results by technique and erosion size (1.0 mm and 1.5 mm). There was a significant difference between panoramic imaging and DSR (P<.05). DSR detected both 1.0-mm and 1.5-mm erosions with greater accuracy (66.7% and 66.7%, respectively) than panoramic imaging (41.7% and 33.3%, respectively) (P<.05).
Table 6 shows chi-square test results by technique and erosion location. There was no significant difference among the locations of erosions with panoramic imaging (P>.05). Erosions at the lateral surface were detected with a significantly higher diagnostic accuracy (P<.05) than at the medial and central locations.
Many studies have been conducted to evaluate the imaging techniques used to diagnose TMD. However, few studies have focused on TMJ erosion and osteophytes.5 DSR is a method used to detect subtle differences among serially taken radiographs by the superimposition of radiographs, which involves considerably lower doses of radiation than other techniques.610
In this study, the diagnostic accuracy of DSR was significantly higher than the diagnostic accuracy of panoramic imaging in detecting both small and large osteophytes (P<.05). This might be explained by the superimposition of surrounding structures on the TMJ and the oblique projection of the joint that prevented the condylar head and articular space from being seen clearly. In the small osteophyte group, 11.6% of osteophytes were detected by panoramic imaging, and in the large osteophyte group, 63.3% of osteophytes were detected by panoramic imaging. The reason for the low detection rate in the small osteophyte group may be due to the poor level of detail in panoramic imaging. DSR eliminated the superimposed structures and, thereby, the area of change was more clearly observed. With DSR, 73.3% of osteophytes in the small osteophyte group and all osteophytes (100%) in the large osteophyte group were detected.
Masood et al.6 evaluated the diagnostic efficacy of panoramic TMJ radiography, DSR, and color-enhanced DSR in the detection of osteophytes in dry human skulls and reported that both of the DSR techniques were significantly better at detecting small and large osteophytes (Az=0.79 for both) than the panoramic technique (Az=0.54). The authors stated that large osteophytes were detected at a higher rate than small osteophytes for all 3 imaging techniques, with Az values for large osteophytes of 0.55, 0.86, and 0.86 for panoramic imaging, DSR, and color-enhanced DSR, respectively, and Az values for small osteophytes of 0.55, 0.74, and 0.72 for panoramic imaging, DSR, and color-enhanced DSR, respectively.
In this study, osteophytes at the lateral location were detected with greater diagnostic accuracy through panoramic imaging than osteophytes at the central and medial locations (P<.05). This might be explained by the limited overlap of anatomic structures at the lateral location compared to the overlap at the medial and central locations. On the contrary, a significant difference was not observed among the locations with the DSR technique (P>.05). This finding might be a result of the elimination of background noise in DSR by removing superimpositions through complete subtraction of anatomic structures, such as the glenoid fossa and temporal component.
Masood et al.6 reported that osteophytes located at the central and medial locations were detected with higher diagnostic accuracy for all 3 imaging techniques (panoramic TMJ imaging, DSR, and color-enhanced DSR) than osteophytes at the lateral location. The authors stated that the superimposition of the glenoid fossa and background noise stemmed from the incomplete subtraction of the temporal component and might be the reason for their findings. However, Ludlow et al.13 found that lesion location did not play a role in the diagnostic accuracy of panoramic imaging. The results of our study differed from the results of Masood et al.,6 as no significant difference was observed among locations in detecting osteophytes with DSR. This could be due to the better standardized projection geometry and the different DSR program that was used in this study.
Currently, CBCT is the modality of choice and is considered the gold standard for evaluating osseous structures of the TMJ. It provides crucial information for diagnosing osteoarthritis.14 CBCT examinations of the TMJ were first evaluated only a few years after CBCT had been introduced to dentomaxillofacial radiology.1516 The diagnostic capability of CBCT in comparison with conventional radiographic techniques has been investigated for different conditions, such as intra-articular fractures, fibro-osseous ankylosis, hypoplasia, and osteoarthritis of the TMJ.1718 CBCT was found to have a sensitivity of 0.80 for detecting erosions/osteophytes in autopsy material when macroscopic examination was used as the gold standard, and it was found to be comparable to CT.19 In a larger series of dry human skulls where the detection of osteophytes was evaluated by CBCT and spiral tomography, no significant difference between the techniques was found.20 The diagnostic efficacy of CBCT for detecting osseous changes has been stated to be superior to panoramic radiography, linear tomography, and magnetic resonance imaging (MRI).1221
In the current study, the diagnostic accuracy of DSR was found to be higher than the accuracy of panoramic imaging for the detection of erosions. This finding may possibly be attributed to the elimination of superimposed structures and background noise during DSR that contributed to clearer visibility of different areas. However, no significant difference was found between panoramic imaging and DSR according to erosion size (1.0 mm vs. 1.5 mm) (P>.05). Erosion causes changes in bone density. In order to observe these changes in conventional radiography, roughly 30%-50% of the bone mineral content must be lost.9 The erosions created in our study were 1.0 mm and 1.5 mm in size, and the change in density generated by these defects was not distinguishable by the human eye. Therefore, no significant difference was found between DSR and panoramic imaging in the detection of 1.0-mm and 1.5-mm erosions.
In our study, while there was no significant difference (P>.05) among locations in panoramic imaging, erosions at the lateral location were detected with greater diagnostic accuracy in DSR than erosions at the central and medial locations (P<.05). In panoramic imaging, the head of the condyle and the inter-articular space could not be displayed clearly due to superimposition of the surrounding structures and the oblique orientation of the beam with respect to the long axis of the condyle. Thus, the location of the erosion did not affect the diagnostic accuracy in panoramic imaging. With DSR, it was predicted that the detection of erosion would be roughly equivalent for all 3 locations because superimposed structures would be removed. However, this was not the case. Erosions at the medial and central location were not easily distinguished from background noise due to the incomplete subtraction of the temporal component.
The high accuracy of CBCT in detecting bone morphology changes in the TMJ has been confirmed.22 CBCT has been shown to have a superior reliability and higher accuracy than linear tomography and panoramic radiography in detecting erosions.12 However, Marques et al.23 showed that the sensitivity of CBCT for detecting bone defects depended on the size of the defects. This information was also confirmed by Patel et al.24 in a study of simulated condylar lesions. Very small defects (i.e., <2 mm) have been shown to be difficult to detect, despite a generally high sensitivity (72.9%-87.5%). In the same study, the authors also investigated the effect of different voxel sizes (0.4 mm and 0.2 mm) in CBCT scans for evaluating simulated defects in pig condyles. They concluded that the sensitivity of the scans increased significantly for small defects (2 mm) as scanning resolution increased, but no difference in sensitivity was found for large defects. When a 0.2-mm voxel size was used, defects were detected with 80% sensitivity regardless of size. In a study comparing conventional tomography, CT, and CBCT with micro-CT and microscopic examinations, CBCT was found to be the most accurate technique for detecting erosive changes in the condyle.22
Our findings showed that DSR might also have the potential for clinical application in diagnosing TMD, particularly with low doses of radiation. However, it requires exact reproducibility of the projection geometry, which is difficult to provide, particularly in busy clinics. Further experimental and clinical studies should be conducted to confirm the diagnostic capacity of DSR in a clinical setting. To our knowledge, there have been no studies investigating the clinical application of DSR for TMD. Going forward, studies comparing DSR and CT/CBCT may also show that DSR can provide an alternative approach to diagnosing TMD that is less invasive and less expensive than arthrography, more readily available than MRI, and requires less radiation exposure than CT/CBCT.
In conclusion, panoramic imaging was found to be significantly less accurate in detecting osteophytes and erosions on the TMJ than DSR. DSR improved the accuracy of detection using panoramic images.
Figures and Tables
Fig. 1
A. Bone chips were used to simulate osteophyte formation on the condylar heads. B. An artificial erosion was created on the medial pole of the right condyle. C. The skull was positioned for panoramic image acquisition.
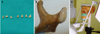
Fig. 2
A. A panoramic image shows the 2.0-mm bone chip placed on the anterolateral aspect of the right condylar head. B. A subtracted image shows the same condyle with the same bone chip.
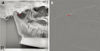
Fig. 3
A. A panoramic image shows a 1.5-mm erosion created on the medial pole of the right mandibular condyle. B. A subtracted image shows the same condyle with the erosion.
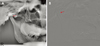
Fig. 4
A. Receiver operating characteristic (ROC) analysis of the detection of overall condylar osteophytes. Curves were plotted from the data obtained for each modality when all osteophyte sizes and locations were considered. B. ROC analysis of the detection of overall condylar erosions. Curves were plotted from the data obtained for each modality when all erosion sizes and locations were considered.
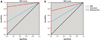
Table 1
Mean areas under the receiver operating characteristic curve for the 2 imaging techniques and all osteophyte sizes and locations

Table 4
Mean areas under the receiver operating characteristic curve for the 2 imaging techniques and all erosion sizes and locations

Notes
References
1. Librizzi ZT, Tadinada AS, Valiyaparambil JV, Lurie AG, Mallya SM. Cone-beam computed tomography to detect erosions of the temporomandibular joint: effect of field of view and voxel size on diagnostic efficacy and effective dose. Am J Orthod Dentofacial Orthop. 2011; 140:e25–e30.


2. Paesani D, Westesson PL, Hatala MP, Tallents RH, Brooks SL. Accuracy of clinical diagnosis for TMJ internal derangement and arthrosis. Oral Surg Oral Med Oral Pathol. 1992; 73:360–363.


3. Ahmad M, Hollender L, Anderson Q, Kartha K, Ohrbach R, Truelove EL, et al. Research diagnostic criteria for temporomandibular disorders (RDC/TMD): development of image analysis criteria and examiner reliability for image analysis. Oral Surg Oral Med Oral Pathol Oral Radiol Endod. 2009; 107:844–860.


4. Larheim TA, Kolbenstvedt A. High-resolution computed tomography of the osseous temporomandibular joint. Some normal and abnormal appearances. Acta Radiol Diagn (Stockh). 1984; 25:465–469.
5. Hussain AM, Packota G, Major PW, Flores-Mir C. Role of different imaging modalities in assessment of temporomandibular joint erosions and osteophytes: a systematic review. Dentomaxillofac Radiol. 2008; 37:63–71.


6. Masood F, Katz JO, Hardman PK, Glaros AG, Spencer P. Comparison of panoramic radiography and panoramic digital subtraction radiography in the detection of simulated osteophytic lesions of the mandibular condyle. Oral Surg Oral Med Oral Pathol Oral Radiol Endod. 2002; 93:626–631.


7. Revesz G, Kundel HL, Graber MA. The influence of structured noise on the detection of radiologic abnormalities. Invest Radiol. 1974; 9:479–486.


8. Brooks SL, Brand JW, Gibbs SJ, Hollender L, Lurie AG, Omnell KA, et al. Imaging of the temporomandibular joint: a position paper of the American Academy of Oral and Maxillofacial Radiology. Oral Surg Oral Med Oral Pathol Oral Radiol Endod. 1997; 83:609–618.
9. Dreyer WP. Technological advances in the clinical diagnosis of periodontal diseases. Int Dent J. 1993; 43:557–566.
10. Woo BM, Zee KY, Chan FH, Corbet EF. In vitro calibration and validation of a digital subtraction radiography system using scanned images. J Clin Periodontol. 2003; 30:114–118.


11. Hekmatian E, Sharif S, Khodaian N. Literature review digital subtraction radiography in dentistry. Dent Res J (Isfahan). 2005; 2:1–8.
12. Honey OB, Scarfe WC, Hilgers MJ, Klueber K, Silveira AM, Haskell BS, et al. Accuracy of cone-beam computed tomography imaging of the temporomandibular joint: comparisons with panoramic radiology and linear tomography. Am J Orthod Dentofacial Orthop. 2007; 132:429–438.


13. Ludlow J, Gilbert DB, Tyndall DA, Bailey L. Analysis of condylar position change on digitally subtracted Orthophos P-4 and Sectograph zonogram images. Int J Adult Orthodon Orthognath Surg. 1995; 10:201–209.
14. Larheim TA, Abrahamsson AK, Kristensen M, Arvidsson LZ. Temporomandibular joint diagnostics using CBCT. Dentomaxillofac Radiol. 2015; 44:20140235.


15. Mozzo P, Procacci C, Tacconi A, Martini PT, Andreis IA. A new volumetric CT machine for dental imaging based on the cone-beam technique: preliminary results. Eur Radiol. 1998; 8:1558–1564.


16. Arai Y, Tammisalo E, Iwai K, Hashimoto K, Shinoda K. Development of a compact computed tomographic apparatus for dental use. Dentomaxillofac Radiol. 1999; 28:245–248.


17. Honda K, Larheim TA, Johannessen S, Arai Y, Shinoda K, Westesson PL. Ortho cubic super-high resolution computed tomography: a new radiographic technique with application to the temporomandibular joint. Oral Surg Oral Med Oral Pathol Oral Radiol Endod. 2001; 91:239–243.


18. Tsiklakis K, Syriopoulos K, Stamatakis HC. Radiographic examination of the temporomandibular joint using cone beam computed tomography. Dentomaxillofac Radiol. 2004; 33:196–201.


19. Honda K, Larheim TA, Maruhashi K, Matsumoto K, Iwai K. Osseous abnormalities of the mandibular condyle: diagnostic reliability of cone beam computed tomography compared with helical computed tomography based on an autopsy material. Dentomaxillofac Radiol. 2006; 35:152–157.


20. Hintze H, Wiese M, Wenzel A. Cone beam CT and conventional tomography for the detection of morphological temporomandibular joint changes. Dentomaxillofac Radiol. 2007; 36:192–197.


21. Alkhader M, Ohbayashi N, Tetsumura A, Nakamura S, Okochi K, Momin MA, et al. Diagnostic performance of magnetic resonance imaging for detecting osseous abnormalities of the temporomandibular joint and its correlation with cone beam computed tomography. Dentomaxillofac Radiol. 2010; 39:270–276.


22. Katakami K, Shimoda S, Kobayashi K, Kawasaki K. Histological investigation of osseous changes of mandibular condyles with backscattered electron images. Dentomaxillofac Radiol. 2008; 37:330–339.

