Abstract
Purpose
This study evaluated the effect of various head orientations during cone-beam computed tomography (CBCT) image acquisition on linear measurements of potential implant sites.
Materials and Methods
Six dry human skulls with a total of 28 implant sites were evaluated for seven different head orientations. The scans were acquired using a Hitachi CB-MercuRay CBCT machine. The scanned volumes were reconstructed. Horizontal and vertical measurements were made and were compared to measurements made after simulating the head position to corrected head angulations. Data was analyzed using a two-way ANOVA test.
Results
Statistical analysis revealed a significant interaction between the mean errors in vertical measurements with a marked difference observed at the extension head position (P<0.05). Statistical analysis failed to yield any significant interaction between the mean errors in horizontal measurements at various head positions.
Treatment of edentulous areas with dental implants is becoming a popular choice for patients and a reliable method for dentists. Diagnostic evaluation and pre-operative treatment planning of implant sites has undergone significant changes since the advent of cone-beam computerized tomography (CBCT).1 Accurate dimensional evaluation of potential implant sites is the key to successful placement of implants during surgery. CBCT provides accurate linear measurements and is comparable to physical measurements with a relative error of less than 1%.2 CBCT allows depiction of the area of interest in three dimensions and in all the three orthogonal planes devoid of superimposition of anatomical structures and provides high-resolution images to make dimensional measurements.345 Pertl et al.6 compared the diagnostic accuracy of panoramic radiography with multi-detector computed tomography (MDCT) and CBCT imaging for determining the alveolar dimensions. They measured the vertical height of the alveolar bone in relation to the mandibular canal on both imaging modalities and concluded that panoramic radiographs have a high range of distortion error that ranges between -0.2 mm and 5.7 mm and CBCT scan errors ranging from -1.5 mm to 0.8 mm.
Although CBCT offers high resolution and dimensionally accurate images, obtaining accurate measurements of potential implant sites as measured by different observers and at different time points remains challenging. Studies have reported conflicting results regarding measurement accuracy in CBCT scans.678910 Ganguly et al. studied radiopaque fiduciary markers made of gutta percha placed over the buccal and lingual cortical plates of the mandibles in cadaver heads and compared them with physical measurements using calipers. They concluded that CBCT is reliable for linear measurement of pre-operative implant sites with an error of less than 1 mm.7 On the other hand, when Leung et al. studied the skull's alveolar bone margins for evaluation of dehiscence and fenestration, they found that CBCT underestimated the bone margin by up to 6 mm, and they attributed this to the decreased spatial resolution of CBCT in comparison to conventional periapical radiography.10 If CBCT was to be routinely used for implant treatment planning, a dimensional inaccuracy of 1-2 mm would be critical in the final selection of the implant size and type and surgical management of the implant site and placement.
In a significant number of large volume CBCT scanners, there are no head positioning restraints or guides that help in standardization of the head position to compare or overlay it with subsequent, comparable CBCT scans. Several studies of the measurement accuracy of CBCT scans have shown that it is critically important to perform quantitative analysis of the scans based on a corrected orientation of the area of interest in all the three orthogonal planes.111213 Inability to account for this discrepancy will result in under- or overestimated measurements at the potential implant site leading to an erroneous estimation of the available bone and can affect the final implant size and type selection. To the best of our knowledge, there has been limited information on measurement discrepancy associated with changes in head orientation during scan acquisition. Thus, this study aimed to evaluate the effect of various head positions and angulations on the accuracy of implant site measurements using CBCT scans. We also sought to evaluate the role of reconstruction software and its ability to adjust and correct the basic orthogonal imaging planes in all three axes (x, y, and z) to the standard position in correction of the existing measurement error.
Six dry human skulls were randomly selected from the University of Connecticut's anatomy lab with no information about gender, age, or ethnicity of the skulls. The selection of skulls was done on the basis of having missing anterior and posterior teeth with continuous and intact buccal, lingual, and palatal bone cortices. Due to the difficulty involved in selecting skulls with specific areas of missing teeth, clinical crowns were modified and or reduced to the level of the alveolar crest using a high-speed fissure bur with a high cooling system to avoid damaging the surrounding alveolar bone. Our goal was to create two edentulous spaces (one anterior and one posterior) that would act as potential implant sites in each arch.
To be able to reproduce centric and eccentric skull/neck angulations resembling clinical situations, we designed a wooden platform with a screw in the center of the platform to seat the tripod stand's base (Manfrotto 804RC2; Cassola VI, Italy), upon which the skulls were fixed. The tripod stand had calibrated angles in the x, y, and z directions that help in tilting and adjusting the wooden platform, in order to simulate the desired angle akin to a clinical situation. This arrangement enabled creation of various skull positions by dialing the desired angle on the tripod's base while imaging the skulls in various simulated head positions with defined/standardized angles.
To reproduce the location of the skull position on the wooden platform, three radiographic points were used. These points helped in localizing the skulls over the wooden platform. Three gutta- percha points (size 15) were placed on the mandible; one in the center, one on the right angle, and one on the left angle of the mandible. Parallel lines were marked on the wooden platform to align with the marked point. A soft pad was placed under the base of the skull in the occipital region to establish parallelism of the skull to simulate a normal occlusal plane, and the skull was fixed in place using clear tape. Each skull was given a random number from 1 to 6 prior to imaging.
After the skull was seated on the wooden platform within the desired position, the platform was fixed to the tripod.
Seven CBCT scans were acquired for each skull in seven different positions using a Hitachi CB MercuRay CBCT machine (Hitachi Medical Systems, Kyoto, Japan) with a 6-inch FOV (Fig. 1). The scans were done with exposure parameters of 120 kVp, 15 mA, and an acquisition time of 10 seconds.
In this pilot study, measurements were done by an Oral and Maxillofacial Radiology resident (HS) under the supervision of an experienced Oral and Maxillofacial radiologist (AT). All the acquired CBCT scan data were stored in the DICOM-3 (Digital Imaging and Communication in Medicine) format and were viewed using InVivo Dental CBCT reconstruction software (Anatomage Inc., San Jose, CA, USA). A split screen dual monitor CBCT reconstruction workstation was used for analysis (HP Compaq DC7800, HP Corp., Palo Alto, CA, USA).
Each scan had six implant sites, which were marked by the radiographic stents placed on the teeth and the edentulous spaces prior to imaging. A simulated panoramic reconstruction was done for all the scans by drawing a curve along the center of the ridge in the axial plane, and cross sectional images of the sites of interest were generated with a slice thickness of 0.5 mm and pitch distance of 1 mm. To minimize error in measurements, the reconstructions were done in an identical manner for all the scans. Based on the location of the radiographic guides, the potential implant sites were identified and displayed as single cross-sectional images on the screen. Evaluation of the site was subsequently performed based on the measurements done at the center of the edentulous site with the radiographic marker located in the center of the cross sectional image (Fig. 2).
A vertical and a horizontal measurement of the alveolar bone were done for each site. The vertical measurement was defined as the height of the alveolar ridge from the crest to the floor of the maxillary sinus or nasal fossa in the maxilla and the inferior border of the mandible or the superior cortex of the mandibular canal in the mandibular arch. Horizontal measurement was defined as the buccolingual width of the alveolar ridge measured halfway along the vertical axis. The measurements of the seven different head orientations of the same implant site were compared.
For the second part of the study, correction of the head position was done by rectifying the acquired scan volume in the X, Y, and Z axes. The Z-axis is most valuable in the correction of the accentuated neck position most commonly encountered in clinical situations. We took Frankfort's horizontal plane to be the desired head position that yields accurate implant site measurements. The aim of this part of the study was to compare the corrected head position with the accentuated head position at acquisition and see the percentage of error that was mitigated.
We performed software correction of three head positions to examine the percentage of software correction of the error factor. This was evaluated after measuring the same six proposed implant sites of all the six cases. These three positions were flexion, extension, and right flexion, which were selected randomly.
Statistical analyses were performed using SAS software. Comparison of each head position with the gold standard (centric) was done using two-way ANOVA. The mean of error interaction for each vertical and horizontal measurement was analyzed. P values less than 0.05 were deemed significant.
The data analyzed for this study included 6 dry skulls with 28 sites (12 anterior teeth and 16 molars). Errors in measurements of the potential implant sites were compared among the 6 different head positions. The measurements were performed with the centric head position as the gold standard. ANOVA was used to test the effects of the various head positions and their interactions.
Tables 1 and 2 show the mean and standard deviations of errors for vertical and horizontal measurements, respectively, for each head position and each area. The ANOVA test results revealed a statistically significant interaction between the mean errors in vertical measurements (P<0.05). According to Tukey's test, the marked difference between the mean errors was observed at the extension head position (P<0.05). The statistical analysis failed to yield any significant interaction between the mean errors in the horizontal measurements at various head positions.
Figure 3 shows the interactions between the mean errors of horizontal and vertical measurements at various head positions in different implant sites. The dots in the plot represent the means of errors for each head position and each area. A significant interaction is observed between the mean errors in the vertical measurements.
The results of this part of the study showed that all the six cases had a significantly different measurement when the sites were measured at eccentric angles and compared to the centric head position acquired with the Frankfort's horizontal plane parallel to the floor of the acquisition room, which we marked as the zero angle. When we did software correction of the eccentric head position and measured the implant sites again, the software correction showed that the implant sites were measured as having very similar dimensions to those measured with the centric head position (Table 3).
In this study we evaluated the linear measurement error at the upper and lower anterior and posterior implant sites in various eccentric head positions during CBCT examination. The majority of cone-beam machines' manuals suggest seating the patient based on reference lines in a way that the occlusal plane is parallel to the floor and the patient's head is in a relaxed position to minimize movement.
Patients with skeletal malformation or malocclusion, who are mostly referred for orthodontic treatment planning or surgical procedures, are at a higher risk of possible measurement discrepancies due to the maxilla-mandible relationship.
Potential dental implant sites are often assessed using CBCT scans to determine the size and type of implant and also to decide on the need for bone augmentation or sinus/site augmentation based on the measurements yielded by CBCT. In this regard, having accurate and reliable measurements becomes very important for final implant selection.
Araki et al.14 found that the linear accuracy of measurements done on CBCT machines with image intensifiers was adequate to translate into the clinical setting. This concept was further shown to be true with a study done by Statemann et al.,15 who compared the CB MercuRay and NewTom CBCT machines with physical measurements and found them to be reliably similar. Based on the results of these studies, we decided to compare only CBCT-derived measurements. This is one of the limitations of the study, in that our institutional rules did not permit cutting and analyzing dry skulls to derive physical measurements. However, we felt that the existing literature adequately supports that CBCT measurements are reliably similar to physical measurements. To address some of the clinical challenges that have been under discussion, this study evaluated the effect of various head positions and orientations during CBCT scans at anterior and posterior implant sites for both upper and lower jaws.
Moreira et al.16 and Frongia et al.17 failed to show a significant measurement discrepancy in CBCT volumes among patients referred for orthodontic treatment planning. This was possibly because they ignored discrepancies of a small number of millimeters, whereas for implant treatment planning, every tenth of a millimeter is significant and any measurement discrepancy could affect the success rate of the implant. Wrong estimation of an implant site may cause irreversible damage to vital anatomical structures such as the inferior alveolar nerve, vascular structures, maxillary sinus, or nasal cavity.
In another study by Sheikhi et al., a measurement error of only 0.5 mm was observed because they used small angles (10-15 mm) and only four selected head orientations. 18 In a recent study by Visconti et al.,19 they studied the effect of the position of the gnathic bones on bone heights and widths for potential implant sites. They scanned ten dry edentulous human skulls using an I-CAT cone-beam machine and standardized the field of view to 6 cm for skulls in standard positions and 10 cm for skulls with other inclinations. The scan was done in four different orientations, each orientation with two angles, 10 and 20. They demonstrated a significant influence of the head position on measurement reliability, and the greatest discrepancy was observed in the upper and lower premolar areas.
In the present study, we used the 6-inch field of view for all our scans and attempted to simulate clinical conditions by creating implant sites in dentate jaws. We did the scans in six different orientations in addition to the standard position and studied more than one lateral movement. Simultaneously, we attempted to evaluate the effect of combined movement in the form of lateral flexion position on the measurement accuracy.
The mean error and standard deviation for vertical and horizontal measurements was calculated for all implant sites. The premolar sites were excluded from analysis due to close proximity with the mental foramen and the nerve loop, which resulted in a wide range of vertical measurements. The statistical analysis of vertical measurements showed mean errors ranging between -2 mm and 3 mm in various head positions, mainly seen in the extension position and in the posterior areas.
The range of discrepancy in horizontal measurements was less than 1 mm at most sites in various head positions except for that of the upper posterior site in the extension position, which was deemed insignificant. One possible explanation would be that since vertical measurements are generally greater than horizontal measurements, in eccentric positions, the dimension that is more severely affected is the vertical dimension. Furthermore, the volume of bone that is displaced in the image layer in an eccentric position is greater along the vertical axis.
The second part of the present study described the percentage of software correction using the tools in the InVivo software program. The correction percentages in vertical and horizontal measurements in the flexion and extension positions ranged between 83-88%. This percentage in the vertical measurement in right flexion position was 75%. It is important to note that that in our study, we only corrected three head positions, and we did not differentiate between site locations in the jaws. That means that software can correct the measurement error by close to 90% in most cases. Our results indicated that the software does not have the ability to completely correct the measurement error to normal; however, in the majority of cases, the correction is close to normal.
The limitation of this pilot study is that observer reliability was not performed due to the large number of measurements that were recorded; therefore, we feel further studies with inter- and intra-observer reliability should be performed. Furthermore, there were certain areas in which correction was not optimally achieved, which may have been due to a discrepancy in more than one axis of the head orientation, panoramic and cross-sectional reconstruction that was sensitive to software manipulation, and inter- and intra-examiner variability.
In conclusion, head orientation and position can significantly affect the vertical and horizontal measurements in CBCT scans. The two main head positions influencing the measurements are extension and flexion. The measurement discrepancy is more frequently seen in the posterior mandibular region. It is recommended that in the lack of appropriate head position during scan acquisition, correction of the Z- axis parallel to the Frankfort's horizontal plane may minimize the measurement error.
Figures and Tables
Fig. 1
Various head positions. A and B. Centric: The three tripod angles marked at zero degrees; it demonstrates zero x, y, and z axes. The occlusal plane and the Frankfort's horizontal plane is parallel to the floor. This position is considered to be the gold standard position. C. Flexion: The skull is tilted downward anteriorly by 20 degrees. D. Extension: The skull is tilted upward and backward by 20 degrees. E. Right flexion: The skull is moved 20 degrees laterally, away from the midline towards the right side. F. Left flexion: The skull is moved 20 degrees laterally, away from the midline towards the left side. G. Right lateral: The skull is directed 15 degrees towards the right shoulder. H. Left lateral: The skull is directed 15 degrees towards the left shoulder.
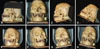
Fig. 2
Vertical and horizontal measurements of the alveolar ridge at a molar site at various head positions. A. Extension, B. Flexion, C. Right, D. Centric, E. Left, F. Right flexion, G. Left flexion.
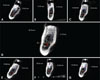
Fig. 3
Mean error of vertical and horizontal measurements for each head position at different implant sites.
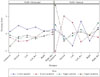
Table 1
Mean and standard deviations of errors for vertical measurements at all implant sites (excluding the premolars) in various head positions (mm)

References
1. Benavides E, Rios HF, Ganz SD, An CH, Resnik R, Reardon GT, et al. Use of cone beam computed tomography in implant dentistry: the International Congress of Oral Implantologists consensus report. Implant Dent. 2012; 21:78–86.
2. Das KP, Jahangiri L, Katz RV. The first-choice standard of care for an edentulous mandible: a Delphi method survey of academic prosthodontists in the United States. J Am Dent Assoc. 2012; 143:881–889.
3. Yim JH, Ryu DM, Lee BS, Kwon YD. Analysis of digitalized panorama and cone beam computed tomographic image distortion for the diagnosis of dental implant surgery. J Craniofac Surg. 2011; 22:669–673.


4. El-Beialy AR, Fayed MS, El-Bialy AM, Mostafa YA. Accuracy and reliability of cone-beam computed tomography measurements: influence of head orientation. Am J Orthod Dentofacial Orthop. 2011; 140:157–165.


5. Raes F, Renckens L, Aps J, Cosyn J, De Bruyn H. Reliability of circumferential bone level assessment around single implants in healed ridges and extraction sockets using cone beam CT. Clin Implant Dent Relat Res. 2013; 15:661–672.


6. Pertl L, Gashi-Cenkoglu B, Reichmann J, Jakse N, Pertl C. Preoperative assessment of the mandibular canal in implant surgery: comparison of rotational panoramic radiography (OPG), computed tomography (CT) and cone beam computed tomography (CBCT) for preoperative assessment in implant surgery. Eur J Oral Implantol. 2013; 6:73–80.
7. Ganguly R, Ruprecht A, Vincent S, Hellstein J, Timmons S, Qian F. Accuracy of linear measurement in the Galileos cone beam computed tomography under simulated clinical conditions. Dentomaxillofac Radiol. 2011; 40:299–305.


8. Tsutsumi K, Chikui T, Okamura K, Yoshiura K. Accuracy of linear measurement and the measurement limits of thin objects with cone beam computed tomography: effects of measurement directions and of phantom locations in the fields of view. Int J Oral Maxillofac Implants. 2011; 26:91–100.
9. Tarazona B, Llamas JM, Cibrian R, Gandia JL, Paredes V. A comparison between dental measurements taken from CBCT models and those taken from a digital method. Eur J Orthod. 2013; 35:1–6.


10. Leung CC, Palomo L, Griffith R, Hans MG. Accuracy and reliability of cone-beam computed tomography for measuring alveolar bone height and detecting bony dehiscences and fenestrations. Am J Orthod Dentofacial Orthop. 2010; 137:S109–S119.


11. Swennen GR, Schutyser F. Three-dimensional cephalometry: spiral multi-slice vs cone-beam computed tomography. Am J Orthod Dentofacial Orthop. 2006; 130:410–416.


12. Loubele M, Maes F, Schutyser F, Marchal G, Jacobs R, Suetens P. Assessment of bone segmentation quality of cone-beam CT versus multislice spiral CT: a pilot study. Oral Surg Oral Med Oral Pathol Oral Radiol Endod. 2006; 102:225–234.


13. Hassan B, van der Stelt P, Sanderink G. Accuracy of three-dimensional measurements obtained from cone beam computed tomography surface-rendered images for cephalometric analysis: influence of patient scanning position. Eur J Orthod. 2009; 31:129–134.


14. Araki K, Maki K, Seki K, Sakamaki K, Harata Y, Sakaino R, et al. Characteristics of a newly developed dentomaxillofacial X-ray cone beam CT scanner (CB MercuRay): system configuration and physical properties. Dentomaxillofac Radiol. 2004; 33:51–59.


15. Stratemann SA, Huang JC, Maki K, Miller AJ, Hatcher DC. Comparison of cone beam computed tomography imaging with physical measures. Dentomaxillofac Radiol. 2008; 37:80–93.


16. Moreira CR, Sales MA, Lopes PM, Cavalcanti MG. Assessment of linear and angular measurements on three-dimensional cone-beam computed tomographic images. Oral Surg Oral Med Oral Pathol Oral Radiol Endod. 2009; 108:430–436.


17. Frongia G, Piancino MG, Bracco P. Cone-beam computed tomography: accuracy of three-dimensional cephalometry analysis and influence of patient scanning position. J Craniofac Surg. 2012; 23:1038–1043.