Abstract
Purpose
ANSI/ADA has established standards for adequate radiopacity. This study was aimed to assess the changes in radiopacity of composite resins according to various tube-target distances and exposure times.
Materials and Methods
Five 1-mm thick samples of Filtek P60 and Clearfil composite resins were prepared and exposed with six tube-target distance/exposure time setups (i.e., 40 cm, 0.2 seconds; 30 cm, 0.2 seconds; 30 cm, 0.16 seconds, 30 cm, 0.12 seconds; 15 cm, 0.2 seconds; 15 cm, 0.12 seconds) performing at 70 kVp and 7 mA along with a 12-step aluminum stepwedge (1 mm incremental steps) using a PSP digital sensor. Thereafter, the radiopacities measured with Digora for Windows software 2.5 were converted to absorbencies (i.e., A=-log (1-G/255)), where A is the absorbency and G is the measured gray scale). Furthermore, the linear regression model of aluminum thickness and absorbency was developed and used to convert the radiopacity of dental materials to the equivalent aluminum thickness. In addition, all calculations were compared with those obtained from a modified 3-step stepwedge (i.e., using data for the 2nd, 5th, and 8th steps).
Results
The radiopacities of the composite resins differed significantly with various setups (p<0.001) and between the materials (p<0.001). The best predicted model was obtained for the 30 cm 0.2 seconds setup (R2=0.999). Data from the reduced modified stepwedge was remarkable and comparable with the 12-step stepwedge.
Radiopacity is a prerequisite of dental materials, specifically for the restorative composite resins.1 Indeed, contrasting with the adjacent recurrent caries and other applied dental materials, over hangs, and voids would be more feasible when a dental material with adequate radiopacity is used.2-4 By the most recent recommendation of ISO 40490:2009, a 1-mm thick sample of dental material should have a radiopacity equal to or greater than a 1-mm-thick sample of aluminum.1
Various researchers have attempted to describe and compare the radiopacity of dental materials in terms of their type (e.g., prosthetic crown, implant cement, composites, endodontic sealers, endodontic core material, dowel core, and core build-ups),5-10 processing (e.g., digital, digitalized, and conventional film),11-14 device setup parameters (e.g., exposure time, voltage, and target-tube distance),2,6,11 and environmental effects (e.g., radiotherapy).15
Briefly, there have been different methods for assessing the radiopacity of dental materials including densitometer, digital or digitalized radiography by means of an aluminum stepwedge or the attenuation coefficient method.2,11 According to ISO 4049:2009, a monochromatic X-ray source performing within the range of 65±5 kVp using a D-speed and #4 sized film should be applied. Moreover, the stepwedge should have a purity of at least 98% aluminum, not consisting of more than 0.1% copper or 1% iron.1
Nearly all of the existing investigations have devoted most of their attention to adopting the minimum radiopacity requirement; meanwhile, there was only a very small group of studies that has critically evaluated the consequent changes in composite resins' radiopacity in the case of violation of other ISO 40490:2009 assumptions.2,6,11 The aim of the present study was to evaluate the effect of setup changes in terms of exposure time and tube-target distance on digital radiopacity of composite resins applying a photostimulable phosphor (PSP) plate.
One-millimeter-thick samples of Filtek P60 (3M/ESPE, St. Paul, MN, USA) and Clearfil (Kuraray, Tokyo, Japan) composite resins were prepared (i.e., five samples of each composite resin with A2 shade). Samples were made using a 15×15×1 mm brass mold. Five samples of each composite resin were created in order to reduce the sampling bias. Thereafter, each sample was light cured with a quartztungsten-halogen (QTH) device and a continuous 400mW/cm2 intensity was applied. In addition, a 12-step wedge (1 mm incremental steps) was made from a 93%-pure alumina alloy ingot (Radravesh Shomal Co., Babolsar, Iran). Deficient samples with large apparent voids in radiography were replaced by sound ones. An attempt was made to keep the sensor perpendicular to the tube direction in order to reduce the heel effect.
Various sets (each composed from a random selection of three samples) were exposed along with the 12-step aluminum step wedge and six different target exposure time/target distance setups (i.e., setup 1: 40 cm, 0.2 seconds; setup 2: 30 cm, 0.2 seconds; setup 3: 30 cm, 0.16 seconds, setup 4: 30 cm, 0.12 seconds; setup 5: 15 cm, 0.2 seconds; setup 6: 15 cm, 0.12 seconds). All of the digital radiographs were taken using a dental X-ray device (Minray, Soredex, Tuusula, Finland) performing at 70 kVp, 7 mA with total filtration of 2 mm aluminum. An occlusal PSP digital sensor (Soredex, Tuusula Finland) was applied. The captured images were processed by a Digora PCT device (Soredex, Tuusula, Finland) with 40-85 µm pixel size, 4-6 lp/mm and 16 bit color depth. The pictures were saved as bitmap images and viewed on a monitor with 15 inch, 32 bit color depth and 1024×768 screen resolution (Syncmaster Dfx 1793, Samsung, Seoul Korea). Digital radiopacities were measured and read in Digora for Windows 2.5 software (Soredex, Tuusula, Finland) at the program default status with no enhancement or further manipulation of contrast. The radiopacities were measured from five different areas (i.e., four corner poles plus the central area), each with a 40×40 pixel area, in order to reduce the measurement bias. Then, the average calculated radiopacity of each sample was taken into account. Thereafter, the obtained radiopacities were transformed to the final digital radiopacity (absorbance) using the following equation: A=-log (T)=-log (1-G/255); where A was the absorbance, T was the transmission, and G was the gray scale (0-255).11 For each set of exposure time/tube-target distance, the radiopacity of aluminum alloy was plotted against the corresponding thickness and a specific linear model was calculated. Briefly, the Y=a+bX equation was obtained from plotting the radiopacity of aluminum steps against their thickness, where "Y" was the radiopacity, "a" was the constant of regression, "b" was the coefficient of the line and "X" was the thickness. Equivalent aluminum thickness of each composite resin sample (X) specific for each setup was calculated by the transformed equation of [(Y-a)/b]. The reliability of the aforementioned software was measured by retesting the random specimens and comparison to the previous measures after two weeks. Also, the reliability of the device and method had been previously investigated.16
Equivalent aluminum thicknesses were expressed as mean (±standard deviation). Comparison of equivalent aluminum thickness by different setups and dental materials was performed by means of a general linear model (GLM) and univariate ANOVA. The homogeneity of variances was tested with further multiple comparisons by Games-Howell. A linear regression model was built for each specific setup. The model descriptive was reported as "a" and "b" parameter and model fit determined to be R2 and the standard error of the estimate. For the assessment of the relationship between the steps and corresponding model errors, various thicknesses of the aluminum step wedge were plotted against studentized residues. Furthermore, to evaluate the hypothesis of accuracy of the reduced step wedge, all analyses were again performed using the information of only the 2nd, 5th, and 8th steps.
The measured equivalent thicknesses of Filtek P60 and Clearfil samples exposed with various setups are displayed in Table 1. There were significant differences (R2=0.905) in equivalent thickness observed for various setups (F (5, 11)=64.093, p<0.001) and between the materials (F (1, 11)=86,449, p<0.001). Also, a significant interaction of the material with the setup existed (F (5, 11)=9.58, p< 0.001). The post-hoc analyses revealed that the equivalent thicknesses of dental materials were significantly lower at an exposure time of 0.12 second and a distance of 15 cm than at the other setups (p<0.001). Linear regression models from plotting of incremental steps of the aluminum stepwedge thicknesses against the relevant digital radiopacities are shown in Figure 1 and Table 2. Regression analyses for the reduced stepwedge are demonstrated in Table 3. Moreover, the distribution of the average magnitude of the errors over the different thicknesses of the stepwedge with an acquired R2=0 is plotted in Figure 2. All measures were performed by a single researcher and the calculated intra-observer R2=0.56 was observed.
The present study investigated the changes in the digital radiopacities of a composite resin according to the exposure time and/or tube-target distance. Our findings revealed that manipulation of these parameters could remarkably alter the obtained radiopacity. Considerable regression models obtained from the various setups confirmed that linear regression of aluminum thickness and equivalent radiopacity/absorbance was a reliable method. Gu et al similarly clarified this conclusion.11 The obtained equation from a 4th degree polynomial curve was slightly better than that of the linear regression model. This is probably due to the bresmsstrahlaung phenomenon that inevitably induces some polychromatic bands, which may explain the small superiority of non-linear models over the linear model.11 Moreover, even with a standard (>98% purity) stepwedge, such small differences were previously reported and expected.11 In addition, when the whole area was uniformly irradiated with almost parallel beams, it lowered the error of the 30 cm and 0.2 seconds setup when compared with the same exposure time with a target-tube distance of 15 cm. This might be explained by the fact that the sensor was more uniformly irradiated over the whole surface when more parallel beams reached its surface with d=30 cm; meanwhile, a distance of 15 cm was not far enough for the exiting rays to change from divergent to a more parallel form.11 Our study had some limitations. Inconsonant with ISO4049 specifications, the aluminum stepwedge that we used was only 93% pure. However, previously, Watts and McCabe disputed that the results of stepwedge were heavily related to its purity, and non-standard stepwedges have been widely applied in research.17 Also, Nomoto et al claimed that this method was not suitable for materials with low radiopacity.18 According to the negligible residue of 0.047, the results from our low purity aluminum stepwedges may be satisfactorily reliable. The main reason for using such a low purity stepwedge in the present study was the lack of access to a high-purity one.
In addition to the low-purity stepwedge, another violation of ISO4049 specifications was that we used a digital system instead of conventional films. Based on the confirmatory results of previous studies, we opted to apply a digital system.2,11,19 The current specifications did not include any parts specified for a digital sensor, which is recommended to be improved, as digital systems have been becoming more popular for reduced patient dose, convenient film processing, and options for manipulating films for better visualizing the area of interest.2,11
Based on ISO4049, the aluminum stepwedge should be made from a single block, or alternatively several 1-mm thick strips of aluminum could be attached together to decrease the processing cost.1,11 More feasibly, a modified stepwedge with reduced steps might be applicable, first proposed by Gu et al.11 We recalculated our models based on data of 2nd, 5th, and 8th steps of the stepwedge. Surprisingly, the model errors were reduced when compared to that obtained from the 12-step aluminum stepwedge. Some processing deficits within higher steps might be responsible for such contrasting data.
Unlike Gu et al11 and Sur et al,2 we determined that the setup changes had influenced the effect on the radiopacities of dental materials. Applying a charge-coupled device (CCD), Gu et al11 concluded that altering the exposure time and tube-target distance would not significantly change the radiopacity.11 Moreover, using D, E, and F speed films and by means of the attenuation coefficient method, Sur et al reported that the different exposure times would not remarkably modify the radiopacity of dental materials.2 Notably, they did not consider target-tube distance as another confounder. Hence, their findings were limited by a fixed distance for various exposure times.
The latitude of the CCD and complementary metal oxide semiconductor sensor (CMOS) receptors, similar to conventional films, are limited, and they cover 0.5-2.5 on the scale of optical density. PSP devices, however, provide a wider range and higher latitude in addition to the linear correlation between the exposure and the gray scale (0-5 on the scale of optical density).20 This major difference may provide a rationale for the significant differences in radiopacities assessed with different setups as compared to the insignificant changes using a CCD receptor and conventional films applied by Gu et al11 and Sur et al.2
The present study supported the reliability of a PSP device to detect the radiopacity of dental materials equivalent to a maximum 12th step radiopacity (absorbance of 1.5, data not shown). The mean residual error exhibited that these measures were widely reliable, accounting for all steps (Fig. 2). Nevertheless, ongoing production of highly opaque materials (e.g., endodontic sealer with 11 mm aluminum equivalent radiopacity) should be taken into account when a digital system with limited gray scale range of 0-255 was considered for the assessment of radiopacity.11
Further, triple modification of kVp, exposure time, and tube-target distance would better determine how a practitioner should modify other parameters in the case of alterations of the rest. As another suggestion, simultaneous investigation of all digital systems including CCD, CMOS, and PSP with conventional films compared to a radiodensitometer might improve our understanding of how these various devices could be differentiated from each other to quantify the radiopacity of dental materials.
In conclusion, based on our findings, we were able to determine that the tube-target distance and exposure time might considerably change the calculated digital radiopacity of dental materials, when a PSP sensor was used. In addition, a modified aluminum stepwedge with reduced steps could be reliably used to calculate the radiopacity more economically than a standard step wedge.
Figures and Tables
![]() | Fig. 1Linear models with corresponding R2 obtained from various distance/exposure time setups for aluminum step wedge. |
Table 1
Equivalent thickness of FiltekP60 and Clearfil measured at various exposure time and target distance designs (unit: mm, mean±SD)
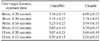
Acknowledgements
Our special thanks to Dr. Sina Haghanifar and Dr. Farida Abesi, assistant professors in the Dentomaxillofacial Radiology Department for their support. We want to express our appreciation to Dr. Homayoon Alaghemand, Dean of the Dental Materials Research Center, for providing composite resins. Also, we would like to acknowledge Mr. Akbarein (CEO, Radravesh Shomal Co., Babolsar, Iran) for his kindness in preparing the stepwedge.
References
1. International Organization for Standardization. ISO 4049: 2009. Dentistry-Polymer based restorative materials. 2009. 4th ed. Geneva: ISO.
2. Sur J, Endo A, Matsuda Y, Itoh K, Katoh T, Araki K, et al. A measure for quantifying the radiopacity of restorative resins. Oral Radiol. 2011. 27:22–27.


3. Wenzel A, Hintze H, Hørsted-Bindslev P. Discrimination between restorative dental materials by their radiopacity measured in film radiographs and digital images. J Forensic Odontostomatol. 1998. 16:8–13.
4. Pedrosa RF, Brasileiro IV, dos Anjos Pontual ML, dos Anjos Pontual A, da Silveira MM. Influence of materials radiopacity in the radiographic diagnosis of secondary caries: evaluation in film and two digital systems. Dentomaxillofac Radiol. 2011. 40:344–350.


5. Martinez-Rus F, Garcia AM, de Aza AH, Pradies G. Radiopacity of zirconia-based all-ceramic crown systems. Int J Prosthodont. 2011. 24:144–146.
6. Wadhwani C, Hess T, Faber T, Piñeyro A, Chen CS. A descriptive study of the radiographic density of implant restorative cements. J Prosthet Dent. 2010. 103:295–302.


7. Ergücü Z, Türkün LS, Onem E, Güneri P. Comparative radiopacity of six flowable resin composites. Oper Dent. 2010. 35:436–440.


8. Taşdemir T, Yesilyurt C, Yildirim T, Er K. Evaluation of the radiopacity of new root canal paste/sealers by digital radiography. J Endod. 2008. 34:1388–1390.


9. Bodrumlu E, Gungor K. Radiopacity of an endodontic core material. Am J Dent. 2009. 22:157–159.
10. Rasimick BJ, Gu S, Deutsch AS, Musikant BL. Measuring the radiopacity of luting cements, dowels, and core build-up materials with a digital radiography system using a CCD sensor. J Prosthodont. 2007. 16:357–364.


11. Gu S, Rasimick BJ, Deutsch AS, Musikant BL. Radiopacity of dental materials using a digital X-ray system. Dent Mater. 2006. 22:765–770.


12. Tanomaru-Filho M, da Silva GF, Duarte MA, Gonçalves M, Tanomaru JM. Radiopacity evaluation of root-end filling materials by digitization of images. J Appl Oral Sci. 2008. 16:376–379.


13. Rasimick BJ, Shah RP, Musikant BL, Deutsch AS. Radiopacity of endodontic materials on film and a digital sensor. J Endod. 2007. 33:1098–1101.


14. Baksi BG, Ermis RB. Comparison of conventional and digital radiography for radiometric differentiation of dental cements. Quintessence Int. 2007. 38:e532–e536.
15. Catelan A, Padilha AC, Salzedas LM, Coclete GA, dos Santos PH. Effect of radiotherapy on the radiopacity and flexural strength of a composite resin. Acta Odontol Latinoam. 2008. 21:159–162.
16. Poorsattar Bejeh Mir A, Poorsattar Bejeh Mir M. How does duration of curing affect radiopacity of dental materials? Imaging Sci Dent. 2012. 42:89–93.
17. Watts DC, McCabe JF. Aluminium radiopacity standards for dentistry: an international survey. J Dent. 1999. 27:73–78.


18. Nomoto R, Mishima A, Kobayashi K, McCabe JF, Darvell BW, Watts DC, et al. Quantitative determination of radioopacity: equivalence of digital and film X-ray systems. Dent Mater. 2008. 24:141–147.


19. Vivan RR, Ordinola-Zapata R, Bramante CM, Bernardineli N, Garcia RB, Hungaro Duarte MA, et al. Evaluation of the radiopacity of some commercial and experimental root-end filling materials. Oral Surg Oral Med Oral Pathol Oral Radiol Endod. 2009. 108:e35–e38.


20. White SC, Pharoah MJ. Oral radiology: principles and interpretation. 2009. 6th ed. St. Louis: Mosby-Elsevier;84.