Abstract
Oxidative stress (OS) has been recognized as a significant cause of suboptimal assisted reproductive outcome. Many of the sperm preparation and manipulation procedures that are necessary in the in vitro environment can result in excessive production of reactive oxygen species (ROS) thereby exposing the gametes and growing embryos to significant oxidative damage. Antioxidants have long been utilized in the management of male subfertility as they can counterbalance the elevated levels of ROS inducing a high state of OS. Few studies have looked into the clinical effectiveness of antioxidants in patients undergoing assisted reproduction. While an overall favorable outcome has been perceived, the specific clinical indication and optimal antioxidant regimen remain unknown. The goal of our review is to explore the sources of ROS in the in vitro environment and provide a clinical scenario-based approach to identify the circumstances where antioxidant supplementation is most beneficial to enhance the outcome of assisted reproduction.
Infertility is defined as the inability of a couple to conceive after at least 12 months of regular, unprotected intercourse. It affects about 1 out of every 6 couples world-wide and is a major cause of social and emotional distress [1]. Since the delivery of the first human via in vitro fertilization (IVF) in 1978 [2], the field of assisted reproductive techniques (ART) has witnessed major advancements that allowed a good number of infertile couples to successfully conceive. However, despite all efforts, only 35% of couples attempting ART obtain a live birth delivery [3]. A myriad of physiological factors were found to influence the success rates of ART, of which reactive oxygen species (ROS) received a great deal of interest in medical literature. ROS are highly reactive oxidizing agents that, at physiologic levels, are naturally involved in various physiologic pathways essential for normal reproduction [4]. In vivo, they are equilibrated by the presence of various enzymatic and non-enzymatic antioxidants that scavenge and neutralize excessive, and therefore detrimental, amounts of ROS. However, in vitro, the unopposed exposure of gametes to excessive levels of ROS results in unfavorable effects on ART outcome. This disturbance in the redox state causes oxidative stress (OS) which elicits its detrimental effects through the exacerbation of lipid peroxidation of sperm plasma membrane lipids, DNA damage and abortive apoptosis [5].
Many of the manipulations performed during the course of ART treatment and described below expose gametes to excessive levels of ROS, which inevitably results in OS given the lack of an effective antioxidant neutralizing system in the in vitro environment of any given standard ART.
As such, efforts were made to enrich our understanding of the sources of ROS during the course of ART and to explore methods that can alleviate their detrimental role. Antioxidants have been investigated both through oral supplementation and in culture media and were found to have an inconsistent effect on ART outcome [67]. The controversy surrounding their actual clinical utility mainly stems from the variations in design and/or antioxidant formulation and dosage used in studies investigating this particular topic. In this review, we have tried to explore the effects of OS in the ART setting and offer real life clinical scenarios where antioxidant use could be most beneficial.
The handling, preparation and manipulation of gametes during the procedure of ART exposes them to a number of ROS-inducing factors (Fig. 1). The family of ROS include oxygen–centered radicals, such as the superoxide anion radical, hydroxyl radical, and nitric oxide radical, in addition to non-radical derivatives, such as hydrogen peroxide, peroxynitrite, and hypochlorous acid [8]. At physiologic levels, ROS exhibit favorable effects that enable the male germ cells to function properly and can potentiate their fertilizing capabilities. Studies have shown that the incubation of spermatozoa with hydrogen peroxide stimulates sperm capacitation, hyperactivation, acrosome reaction, and oocytes fusion [91011]. Other ROS, such as superoxide and nitric oxide have also been shown to promote sperm capacitation and acrosome reaction [12].
On the other hand, follicular and seminal fluid contain antioxidants which are capable of stabilizing or deactivating free radicals, thus ensuring their existence at optimal physiologic levels. Two antioxidant systems have been recognized; the enzymatic system which includes superoxide dismutase, catalase, and glutathione peroxidase, and the non-enzymatic system which includes ascorbic acid (vitamin C), urate, tocopherol (vitamin E), pyruvate, glutathione, and others [13].
In the ART setting, oocytes and sperm which are removed from their natural microenvironments can be exposed to excessive levels of ROS as the antioxidant defense mechanisms are lost [14], ultimately resulting in a state of OS [15]. Due to its ability to alter cellular molecules such as lipids, proteins, and nucleic acids, OS exerts detrimental effects on fertility through disrupting sperm membrane integrity and eliciting structural sperm DNA damage, mitochondrial alterations, adenosine triphosphate depletion, and apoptosis [1617].
ROS during ART procedures could either originate endogenously from gametes or be triggered by exogenous environmental factors [15].
During spermatogenesis, defects in cytoplasmic extrusion sometimes may result in the development of immature sperm that have a surplus of residual cytoplasm [1819]. Residual cytoplasmic droplets have been associated with excessive ROS generation mediated by the concurrent increase in glucose-6-phosphate-dehydrogenase (G6PD) activity seen in abnormal spermatozoa. G6PD stimulates glucose influx through the hexose monophosphate shunt raising the intracellular availability of nicotinamide adenine dinucleotide phosphate, which is a major source of electrons for spermatozoa used to fuel the generation of ROS [20].
The sperm mitochondria are another major source of ROS as they are principally involved in energy production required for normal sperm motility. Alterations in sperm mitochondria were associated with increased ROS production [21], which further promotes damage to sperm mitochondrial membranes triggering a vicious cycle of ROS production and mitochondrial dysfunction.
Leukocytospermia, or increased semen leukocytes, is a well-known cause of male infertility [22]. It is commonly encountered secondary to infection or inflammation of the prostate or seminal vesicles [22] and is associated with overproduction of ROS and consequently OS. During the course of ART, sperm damage from leukocyte-produced ROS can occur after removal of seminal plasma during the preparation methods. Several research groups documented a significant positive correlation between the number of seminal leukocytes and seminal ROS levels [2324]. Moreover, seminal leukocyte levels were inversely related to sperm concentration (p=0.0001), motility (p=0.0001), normal sperm morphology (p=0.002), and directly related to sperm DNA fragmentation (SDF) measures (p=0.0563) [25]. Finally, seminal leukocytes may in fact induce ROS production by human spermatozoa through a mechanism that is not clearly understood [26], but possibly due to disturbing the mitochondrial membrane potential and activation of caspase 3/7 which eventually leads to an increased sperm superoxide production [27].
Several environmental factors taking place during both IVF and intracytoplasmic sperm injection (ICSI) inevitably exposes sperm, oocytes, and embryos to ROS which is believed to significantly influence the outcomes of these procedures [2829]. While it is arguably relevant that the longer incubation time during IVF places the gamete and embryo at a higher risk of ROS-exposure than ICSI [143031], ICSI carries a greater risk of ROS-induced oocyte DNA damage through the direct injection of ROS containing medium along with the sperm during the procedure [32]. More importantly, ICSI bypasses the natural sperm selection opportunity. Therefore, there is a potential risk of injecting sperm with high ROS-induced DNA damage into the oocyte [33].
Any aerobic environment promotes enzyme activity and release of ROS. In vivo, early embryo development has been documented to take place in a microenvironment with low oxygen tension [34]. This has led to the assertion that attempts at mimicking the in vivo environmental conditions during the course of ART should have a favorable reproductive outcome [34]. Indeed, studies have confirmed such an assertion where embryos cultured at atmospheric oxygen concentration developed significantly higher levels of DNA fragmentation compared with embryos grown in an environment of low oxygen tension [35]. In another prospective randomized study, Kasterstein et al [36] used sibling oocytes of 258 women to compare the effect of 5% O2
vs. 20% O2 concentrations on embryo development and clinical outcome. While reporting comparable fertilization and cleavage rates between both groups, the authors observed significantly better blastomeres (p<0.05), top-quality embryos on day 3 (p<0.02), implantation (p<0.03), pregnancy rates (p<0.05), and live birth rate (p<0.05) with the 5% O2 compared to 20% O2 concentrations.
Visible light can potentially trigger ROS release and act as an exogenous source of OS during ART procedures [37]. It is therefore suggested that minimizing exposure of oocytes, zygotes and embryos to visible light should yield superior ART outcomes. While such an assumption has not been fully investigated, animal studies confirmed a protective effect for smaller amounts of short-wavelength visible light. Takenaka et al [38] exposed hamster and mouse zygotes to sunlight, and to cool or warm white fluorescent light and measured highest levels of ROS and apoptosis with sunlight exposure, followed by cool white fluorescent light and warm white fluorescent light. After transferring embryos to surrogate mothers, the authors observed that those developed from zygotes shielded from light (control) had a better rate of progress to full term compared to those exposed to warm white and cool white fluorescent lights for 15 minutes. Girotti [39] evaluated the effects of visible light and found that it could cause OS induced damage to cholesterol and unsaturated lipids contained in cell membranes.
The composition of the media used during the culture of human oocytes and embryos has a direct influence on embryo quality and subsequently ART success [14]. The presence of metallic ions, such as iron or copper in the culture medium is particularly influential as it may lead to increased ROS generation [40] by activation of relevant enzymes or simply by catalysis by Fe2+/Fe3+ ions in the Haber-Weiss and Fenton reactions to produce hydroxyl radicals [41]. Bedaiwy et al [30] reported that increased levels of ROS in the culture medium correlated with impaired blastocyst development, cleavage, and fertilization, as well as increased fragmentation rates. As such, the addition of metal chelators to culture media have been investigated with a goal to reduce the ability of metals to react and produce ROS in the in vitro setting. Transferrin, an iron chelator, has been found to decrease lipid peroxidation and formation of ROS when added to the culture media [42].
pH changes can influence cellular homeostasis affecting crucial physiologic properties, such as protein synthesis, mitochondrial function, cytoskeletal regulation, and cellular metabolism [43]. In culture media, pH alterations can negatively impact sperm motility, oocyte maturation, and embryo development [43]. Incubator temperature is another important variable which should constantly be maintained at human body temperature [44], as increasing temperatures can decrease pH levels [45] and cause ROS-induced cellular damage [46].
Centrifugation is a routine step performed during sperm preparation to remove seminal plasma and other constituents, such as dead cells, debris, and immature sperm, which are a potential source of ROS [47]. However, the centrifugation process itself can aggravate ROS levels, with a direct relationship between centrifugation time and ROS formation [47]. Longer centrifugation time exposes sperm to higher temperatures negatively affecting sperm parameters [48].
Cryopreservation involves the preservation of gametes/embryos and whole ovarian or testicular tissues by cooling to sub-zero temperatures followed by thawing for use in ART [49]. Although the use of cryoprotectants and optimized protocols, such as vitrification may offer protection, freezing, and thawing exposes sperm to extreme stress capable of modifying their structure and integrity [50]. Cryopreservation can cause sperm membrane damage as a result of lipid peroxidation [51] and cause OS induced DNA fragmentation, thereby decreasing sperm motility and viability [5253].
Several studies have evaluated the effect of OS from both male and female origins on the clinical outcome of ART. Hammadeh et al [54] assessed the concentrations of ROS and total antioxidant status in seminal plasma and sperm parameters of 26 male partners of patients undergoing IVF or ICSI. While these authors did not report any significant difference between patients who achieved pregnancy and those who did not, both groups demonstrated a negative correlation between ROS concentration and sperm vitality, membrane integrity, and morphology. More importantly, a significant negative correlation between the ROS concentration in seminal plasma and fertilization rate in both IVF/ICSI programs was detected. Ozatik et al [55] evaluated serum total oxidant and antioxidant levels prior to and after oocyte pickup and embryo transfer. Seventy normal females were enrolled who were undergoing ART due to severe male factor infertility. The authors determined that clinical pregnancy is more likely to occur in women with a higher total antioxidant status prior to and after ova pickup and prior to and after embryo transfer. OS index before ovum pickup was one of the most important determinants for clinical pregnancy, where the higher the OS index the lower the pregnancy rate.
Antioxidants have been utilized in the management of male infertility in various clinical settings (Table 1). While generally a favorable effect has been observed, considerable variability in the reported outcomes exist and is principally related to lack of uniformity of the clinical circumstances in which the antioxidant is investigated and the type, duration, and dosage of the substance used. Therefore, it is imperative to clearly recognize the circumstances where antioxidant use is most helpful. During the course of ART, the following clinical scenarios warrant treatment with antioxidant supplementation.
A 32-year-old man who was diagnosed with non-Hodgkin's lymphoma presents to the andrology laboratory for sperm cryopreservation before the start of chemotherapy. He is not married and his semen parameters were found to be normal on routine semen analysis (World Health Organization [WHO] 2010 [56]).
A suitable approach to protect human spermatozoa from the deleterious effects of ROS during the cryopreservation process is the addition of antioxidant supplements to the freezing solution [57]. Antioxidants, through their ability to quench or neutralize excess ROS could offer protection against the OS induced by the cryopreservation process [585960]. Several antioxidants have been investigated as cryoprotectants; they include quercetin, catalase, resveratrol, vitamin E, and selenium (Se).
Quercetin is a bioflavonoid with well documented antioxidant and anti-inflammatory properties [6162] that has been investigated as a potential supplement for improving cardiac health and preventing cancer. Zribi et al [58] evaluated the effect of quercetin as a cryoprotectant preventing sperm damage during the freezing-thawing process. Seventeen semen samples were divided into two aliquots, the first was cryopreserved with a freezing solution only while the second with the freezing solution+quercetin up to a concentration of 50 µM. While a significant improvement in post thaw sperm parameters was detected regarding sperm motility (p=0.007), viability (p=0.008), and DNA integrity (p=0.02) in the quercetin group compared to the control group, no effect on caspase 3 activation, a measure of OS, was observed between groups. Animal studies have also confirmed a similar protective effect of quercetin which significantly improved the sperm motility and zona binding ability, and reduced DNA fragmentation in cryopreserved stallion sperm [63].
Catalase is a common enzyme found in nearly all living organisms exposed to oxygen protecting cells from OS through catalyzing the decomposition of hydrogen peroxide (H2O2) to water and oxygen [64]. Moubasher et al [60] investigated the protective effects of catalase on semen parameters and sperm DNA integrity during cryopreservation. While there was no significant difference in sperm concentration, a significant increase in the percentages of progressive motility and sperm vitality and a significant decrease in the percentage of DNA damage were observed when catalase was added to the media before cryopreservation.
Resveratrol, a known antioxidant, is one of the most important polyphenols found in red wine. It has many health benefits, most notably alleviation of age-related diseases, such as neurodegeneration, carcinogenesis, and atherosclerosis [65]. Few human and animal studies have confirmed a potential benefit for resveratrol use during cryopreservation on sperm parameters after the thawing process. A protective effect of resveratrol against freeze induced sperm DNA damage has been identified [66]. Furthermore, the addition of resveratrol was able to prevent post-thaw lipid peroxidation in both fertile and infertile men thereby preventing the damaging effect of cryopreservation on sperm concentration and morphology [67]. On the contrary, while animal studies have confirmed a protective effect for resveratrol on sperm DNA integrity, they failed to find a significant influence on semen parameters and OS measures [6869].
Vitamin E is perhaps the most commonly utilized antioxidant in cryoprotective media due to its favorable effects on post-thaw motility and DNA integrity [70]. Taylor et al [71] divided 23 normal and 20 abnormal semen samples into three aliquots prior to cryopreservation. The first aliquot remained untreated and was mixed with cryopreservation medium while the second and third aliquots were mixed with cryopreservation medium containing either 100 or 200 µM vitamin E analogue. After a freeze/thaw process, samples were assessed for motility, vitality, and DNA integrity. A direct relationship between vitamin E and post-thaw motility (p=0.041) was observed. While post-thaw motility was significantly improved with the addition of 200 µM vitamin E (p=0.006), neither vitality nor SDF were altered. These results suggest that the addition of vitamin E to cryopreservation medium may improve post-thaw motility.
Se is an essential trace element mainly involved in normal testicular development and spermatogenesis protecting sperm DNA against OS. It is believed that Se provides protection against OS through potentiating the activity of glutathione peroxidases and thioredoxin reductases. Se deficiency has been linked to sperm mid-piece morphologic abnormalities and impairment of sperm motility [72]. Most in vitro studies examined the effects of Se on cryopreservation techniques. Early reports on the in vitro use of Se in semen extenders showed a protective effect of the element against freezing injury [73]. The addition of 1 and 2 µg/mL, respectively, of Se to the semen before freezing significantly increased the post-thaw motility compared to non-treated specimens. Similar results were seen in animal studies; where the addition of identical concentrations of Se to semen extenders significantly improved post-thaw sperm motility, viability, membrane integrity and semen total antioxidant capacity and reduced SDF in water buffaloes sperm [74].
Cryopreservation may lead to deleterious changes of sperm structure and function that occur secondary to the state of OS induced by the freezing and thawing process. While further studies are needed, the supplementation of freezing media with antioxidants in vitro appears to improve sperm quality after thawing.
A 38-year-old gentleman presents complaining of a 4-year duration of primary infertility. His semen analysis revealed a sperm concentration of 3.4 million/ml, total motility of 15% and a normal morphology of 2% (WHO 2010 [56]).
This patient is a candidate for ART due to poor semen quality. As previously explained in this article, many of the steps performed during the course of ART impact the spermatozoa, oocytes, and embryos due to OS, thus lowering the clinical outcome of ART [3747]. Consequently, a number of antioxidant molecules have been investigated with the goal to reduce OS measures during the course of ART and hoping for higher clinical outcome.
Vitamin E (α-tocopherol) is a fat soluble organic compound located mainly in cell membranes. It has the ability to quench free hydroxyl radicals and superoxide anions allowing it to reduce lipid peroxidation initiated by ROS at the level of plasma membranes. At this level, vitamin E interacts with water soluble antioxidants, such as ascorbate (vitamin C) and glutathione which regenerate the lipid soluble vitamin E.
① Oral supplementation: Studies exploring the effects of vitamin E supplementation recorded increased rates of sperm motility, in vitro sperm function, and fertilization rates in IVF, with decreased levels of malondialdehyde (MDA) compared to placebo groups [7576]. In patients with prior history of IVF failure, 3 months supplementation of 200mg vitamin E resulted in significantly higher fertilization rate and lower MDA levels in the subsequent IVF cycle [75]. The in vitro use of vitamins E and C also had favorable effects on sperm function tests, where a reduction in the levels of ROS and SDF were observed in both normozoospermic and asthenozoospermic samples [77].
② In vitro use: The in vitro antioxidant activity of vitamin E was investigated on 122 semen samples where, at a dose of 10 mmol/L, significant suppression of lipid peroxidation was detected leading to preservation of sperm motility [78].
Vitamin C (ascorbic acid) is a water–soluble compound that is highly concentrated in seminal plasma [79]. It has the ability to neutralize hydroxyl and superoxide radicals as well as the highly reactive hydrogen peroxide thereby providing sperm with protection against OS [80]. Studies have confirmed the presence of an inverse relationship between seminal vitamin C concentrations and abnormalities in sperm parameters. Lower vitamin C levels and higher ROS levels were observed in the seminal plasma of asthenozoospermic men [81]. Additionally, dose–dependent positive correlations between vitamin C levels and sperm motility [82] and the percentage of normal sperm morphology [83] were detected.
① Oral supplementation: Vitamin C has been mainly investigated in combination with other vitamins. Patients treated with vitamins C and E had a significant reduction in the percentage of SDF and a significant improvement in clinical pregnancy and implantation rates following ICSI [84]. A randomized double-blind, placebo-controlled trial investigated a combined antioxidant regimen, including vitamin C (lycopene 6 mg, vitamin E 400 IU, vitamin C 100 mg, zinc 25 mg, Se 26 µg, folate 0.5 mg, garlic 1 g) in couples undergoing ICSI. The authors reported a significant improvement in viable pregnancy rate in the treatment group, where 38.5% of transferred embryos resulted in a viable fetuses compared to 16% in the placebo group [85].
② In vitro use: Experiments designed to identify the optimal in vitro vitamin C level revealed that higher doses (>1,000 mmol/L) were found to paradoxically increase ROS levels causing worsening of sperm motility [86]. However, at therapeutic doses, a dose-dependent positive effect was observed, with optimal motility achieved after incubation in 800 mmol/L for 6 hours [86]. One animal study examined the direct effect of vitamins C and E on mouse blastocyst development. The authors reported an increase in blastocyst development when incubated in culture media containing 50 µM vitamin C or 400 µM vitamin E, however with significant levels obtained with vitamin C only [87].
Carnitine is another water-soluble antioxidant actively involved in sperm motility as it is considered as a fuel source of energy. It has anti-cytokine and anti-apoptotic actions and can prevent lipid peroxidation [88]. Its favorable effect on sperm motility was confirmed by a number of studies showing significant improvements in asthenozoospermic men [8990].
① Oral supplementation: While no specific studies were performed on the effect of oral carnitine supplementation on ART outcome, most studies involving oral carnitine therapy have demonstrated a significant benefit particularly on sperm motility [899091]. Lenzi et al [89] demonstrated significant improvement in sperm motility and pregnancy rate in patients with oligoasthenoteratozoospermia (OAT) receiving a carnitines. Similarly, Balercia et al [90] confirmed such response to therapy specifically among patients with lower baseline values of motility. A double blind, placebo controlled randomized clinical trial examining therapy with pentoxiphylline (PF, 400 mg twice daily) and/or L-carnitine (500 mg twice daily) reported an improvement in all semen parameters with the combination therapy in compSarison to PF only, L-carnitine only, or placebo [92]. The authors concluded that this combination regimen may influence ART as it caused a shift in the choice of the recommended ART treatment among infertile patients.
② In vitro use: Al-Dujaily et al [93] investigated semen from 100 infertile men dividing their samples into four groups, control, pentoxifylline only, L-carnitine only and a combination of pentoxifylline and L-carnitine. Results showed a statistically significant increase in the percentage of progressive motility, the highest of which occurred among the combination group [93].
Coenzyme Q10 (CoQ10) is an essential antioxidant that is ubiquitous to almost all body tissues. It is highly concentrated in sperm mitochondria where it plays an integral role in energy production [9495].
① Oral supplementation: When administered orally, CoQ10 is capable of attenuating OS in seminal plasma and improve semen parameters and antioxidant enzymatic activity. Studies evaluating the oral use of CoQ10 have revealed an improvement in sperm concentration and motility together with an increase in spontaneous pregnancy rates [9697]. The effect of oral CoQ10 therapy on the outcome of ICSI was addressed by Lewin and Lavon [94], who treated 17 patients with low fertilization rates after a prior ICSI trial with oral CoQ10 (60 mg/d) for a mean of 103 days before a subsequent ICSI trial. No significant changes were noted in most sperm parameters, however a significant improvement in fertilization rates from a mean of 10.3%±10.5% to 26.3%±22.8% after CoQ10 therapy (p<0.05) was detected.
② In vitro use: in the same previous study, Lewin and Lavon [94] evaluated 38 semen samples from infertile men with prior failed IVF/ICSI. Samples were incubated for 24 hours in HAM's medium alone, HAM's with 1% dimethyl sulfoxide and HAM's with 5 or 50 µM CoQ10, respectively. A significant increase in motility was observed in the 50 µM CoQ10 subgroup of sperm from asthenozoospermic men (p<0.05) [94]. More importantly, CoQ10 supplementation increased fertilization rates in the subsequent cycle. Another study, assessed the effects of an antioxidant formula containing zinc, D-aspartic acid, and CoQ10 on sperm parameters and OS measures in vitro [98]. The authors observed, that after incubating semen samples from 12 normozoospermic and 12 asthenozoospermic patients for 3 hours, the antioxidant formula maintained sperm motility in normozoospermic men (37.7%±1.2% vs. 35.8%±2.3% at time zero) and improved it significantly in asthenozoospermic patients (26.5%±1.9% vs. 18.8%±2.0% at time zero) when compared to controls (p<0.01). Moreover, a statistically significant lower sperm lipid peroxidation level was detected in both normozoospermic men and asthenozooseprmic patients (p<0.05).
N-Acetyl cysteine (NAC) is a nucleophilic amino acid that is converted in the body to cysteine, a precursor to glutathione which is the main endogenous antioxidant against lipid peroxidation in the epididymis and testes [99]. Through scavenging lipid peroxides and H2O2, it confers protection for lipid constituents, thus preserving sperm viability and motility [100]. Deficiency of glutathione was found to be associated with sperm mid-piece instability and abnormal motility [101].
① Oral supplementation: A direct dose- and time-dependent reduction of seminal ROS after exposure of human spermatozoa to NAC was reported by Oeda et al [102] concluding that treatment of patients with NAC might be a useful option in reducing OS. Oral treatment with NAC (600 mg daily) was associated with significant improvement in sperm motility in comparison to placebo in 120 patients with idiopathic infertility [103]. Moreover, the authors confirmed its beneficial effect on measures of OS through reporting higher serum total antioxidant capacity and lower total peroxide and OS index in the NAC-treated group compared with the control group.
② In vitro use: Prolonged in vitro incubation of human spermatozoa is associated with activation of mitochondrial ROS and loss of sulfhydryl (-SH) groups. Aitken et al [104] demonstrated that the addition of nucleophilic compounds to semen samples, provided protection against ROS-induced motility reduction. NAC was also found to exhibit an inhibitory effect on germ cell apoptosis in the human seminiferous tubules in vitro [105].
OS occurring during the course of IVF/ICSI significantly impairs the procedures' outcome. The oral and in vitro use of vitamin E, vitamin C, carnitines, CoQ10, NAC, and PF appear to convey protection against OS and improve the utilized sperm quality. Despite this potential benefit, additional high-quality studies are required before adopting the routine use of antioxidants by laboratories before ART. However, one has to caution against a possible over-dosage of antioxidants as this might throw the fine balance of the bodily redox potential from OS into reductive stress which is as dangerous as OS [106107].
A 25-year-old married man presents complaining of secondary infertility of 8 years' duration. The couple has had recurrent first trimester spontaneous pregnancy loss. His semen analysis revealed oligozoospermia, however, with elevated sperm DNA fragmentation index of 54% as determined with the sperm chromatin structure assay.
Elevated SDF has been recognized as an influential factor in cases of recurrent spontaneous pregnancy loss. Recent evidence indicate that such patients are better managed with ICSI due to a superior reproductive outcome [108]. However, the high SDF in patients undergoing ICSI puts them at an increased risk of pregnancy loss in comparison to patients with normal SDF [108].
While advanced sperm selection techniques could carry a potential benefit for patients with high SDF undergoing ICSI [109110], it is best to aim for strategies that may reduce the level of SDF even before performing the procedure. As such, few studies have reported the effect of multiple antioxidants on measures of SDF during the course of ART.
Kodama et al [111] assessed the effect of oral vitamin E supplement (200 mg/d) combined with vitamin C (200 mg/d) and glutathione (400 mg/d) in 19 infertile men over a 2-month period. A significant increase in sperm concentration and a decrease in oxidative DNA damage (measured by 8-hydroxy-2′-deoxyguanosine levels) were detected. Greco et al [112] assessed the effect of oral antioxidant supplementation on males with more than 15% SDF undergoing ICSI and who had at least one unsuccessful previous ICSI trial. After a treatment regimen consisting of 1 g of vitamin C and vitamin E for 2 months, a 76% decrease in sperm DNA damage was reported in the treatment group, while pregnancy rates increased from 7% to 48%, and implantation rates from 2% to 19%. Furthermore, Abad et al [113] examined semen parameters and SDF levels following different incubation times in samples obtained prior to and 3 months after treatment with antioxidants in 20 infertile men with asthenoteratozoospermia. L-carnitine (1,500 mg), vitamin C (60 mg), CoQ10 (20 mg), vitamin E (10 mg), vitamin B9 (200 µg), vitamin B12 (1 µg), zinc (10 mg), and Se (50 µg) were the antioxidants investigated by these authors. In addition to detecting a significant increase in sperm concentration (p=0.04), motility (p=0.04), vitality (p=0.002), and morphology (p=0.04) after antioxidant therapy, significant improvements in DNA integrity were also witnessed at all incubation points (p<0.01).
The combination of vitamin C (600 µM), vitamin E (30 and 60 µM), and urate (400 µM) provided significant protection against SDF when added under in vitro conditions to sperm wash media or during IVF procedures [114].
Folic acid is a vitamin B that is known to play a vital role in nucleic acid synthesis and amino acid metabolism. It also carries free radical scavenging abilities. Low levels of folic acid in seminal plasma has been associated with increased levels of SDF [115]. Studies investigating the efficacy of folic acid in reversing SDF have been contradictory [116117118].
A 34-year-old gentleman presents complaining of primary infertility of 2 years' duration. His semen analysis reveals a total motile sperm count (TMC) of 12 million. His wife is 26 years of age and her gynecologic evaluation was normal.
The patient in this clinical scenario may be a candidate for intrauterine insemination (IUI). The criteria for patient selection for IUI depends principally on the TMC together with the presence of normal female ovulation and patent reproductive tract. In general, at least 5 million motile sperm should be present in fresh semen samples and at least 1 million sperm should be inseminated after sperm preparation [119]. While the patient in this clinical scenario can be offered IUI, attempts at further increasing his TMC or protect his sperm parameters against OS induced injury by sperm preparation methods should be favored to improve his IUI outcome. Few studies on antioxidant supplementation have evaluated their effect on the TMC or on IUI outcome.
In one randomized controlled study, Attallah et al [120], particularly enrolled 60 patients with idiopathic asthenozoospermia undergoing IUI. Patients were equally randomized into two groups; treatment group: 600 mg NAC for 12 weeks and a control group: no treatment. The authors reported significantly higher sperm concentration and motility in the treatment group compared with the control group, p=0.04 and p=0.03, respectively. However, this study failed to find a significant effect on the clinical pregnancy rate between both groups. Several other antioxidants were found to influence the TMC in patients with oligoasthenozoospermia. L-carnitine (2 g/d) given to 30 men for a period of 3 months showed a statistically significant improvement in sperm concentration and total motility compared with the placebo [121]. The combination of 600 mg NAC and 200 µg Se resulted in a significant improvement in all semen parameters with a dose-dependent positive correlation between the sum of Se and NAC concentrations, and mean sperm concentration, motility and the percentage of normal sperm morphology [103]. Safarinejad [96] randomly assigned 212 infertile men with idiopathic OAT to receive either 300 mg CoQ10 or a placebo orally for a period of 26 weeks. He demonstrated a significant increase in sperm density and motility with CoQ10 therapy (p=0.01). Finally, Eskenazi et al [122] evaluated the nutrient intake of 97 healthy non-smoking men comparing the results with the participant's semen parameters. They observed statistically significant direct relationship between vitamins C (p=0.009) and E (p=0.05) intake and measures of total progressive motile sperm count.
In addition to the female partner's status, the IUI outcome is dependent on the quality of the sperm being injected. The single study investigating the use of NAC in patients undergoing IUI demonstrated a significant improvement in sperm quality prior to the procedure without documented improvement in pregnancy outcome. The oral administration of vitamins C and E, carnitines, and CoQ10 was found to significantly improve TMC thereby theoretically improving the chances of IUI success. Further studies are still required in this particular situation in order to withdraw sound conclusions.
A 38-year-old patient with non-obstructive azoospermia has underwent microsurgical testicular sperm extraction coupled with a fresh ICSI cycle. Analysis of testicular tissues revealed the presence of 2~3 immotile sperm per high power field.
The understanding that patients with non-obstructive azoospermia may still harbor sperm in selective areas of their testes that can be harvested and used for ICSI provided such patients with hope to father biologic children. Nonetheless, work with testicular sperm is slightly more demanding. In many instances, testicular sperm are immotile as they have not achieved this characteristic which is usually obtained during epididymal transit [123]. Therefore, in order to identify viable testicular sperm for ICSI methods, such as prolonged incubation or hypo-osmotic swelling test were utilized before the procedure [124125]. While these methods were proven to be successful, they also may illicit harm to the sperm [126127128]. As such efforts were made to investigate antioxidant supplements that can trigger motility in vitro and help in identification of sperm for ICSI.
PF has been specifically utilized to improve the quality of testicular sperm obtained surgically. It is a xanthene derivative that has anti-inflammatory and muscle relaxing capabilities which allowed it to be used for the treatment of peripheral vascular disease [129]. Its ability to inhibit phosphodiesterase was investigated as a potential inducer of sperm motility in vitro [130131]. Kovacic et al [132] in their retrospective study assessed the effect of short time exposure of testicular samples containing only immotile sperm to PF. Seventy seven testicular sperm samples were included and divided into 2 groups. In group 1 (n=30), ICSI was performed with untreated testicular sperm; while in group 2 (n=47), the samples were treated for 20 minutes with PF (1.76 mmol/L) before ICSI. Almost all samples that were treated with PF (95.7%) started to move after treatment. A shorter ICSI time (30 min vs. 120 min), higher fertilization rate (66% vs. 50.9%; p<0.005) and better number of embryos per cycle (4.7±3.3 vs. 2.7±2.1; p<0.01) were observed in the PF vs. the non-PF groups. The clinical pregnancy rate per cycle was 38.3% in the PF group and 26.7% in the non-PF group.
In another comparative study, Griveau et al [133] performed 108 ICSI cycles with testicular sperm obtained from men with non-obstructive azoospermia. In 64 samples, no motile sperm were observed and hence the samples were treated with PF (1.5 mmol/L) for 10 minutes, whereas the other 44 ICSI cycles were performed using spontaneously motile spermatozoa and served as a control group. In 100% of treated samples, PF was successful in initiating sperm motility. A fertilization rate of 54.2% was observed in the PF treated group versus 66.7% in the control group (p<0.02). Pregnancy occurred in 29 out of the 64 PF cycles (45.3%/cycle), whereas 12 pregnancies occurred in the control group (27.3%/cycle). This ability of PF to enhance sperm motility in vitro has also been reproduced in several other studies [132134135]. On the other hand, an excessive treatment of spermatozoa with PF, particularly for IVF, should be avoided as PF due to its ability to inhibit phosphodiesterase activity results in subsequent increase in cyclic adenosine monophosphate levels and stimulation of acrosome reaction. Since perfect timing of acrosome reaction is essential for IVF, an over-stimulation with PF can result in an premature acrosome reaction and therefore, fertilization failure.
Sperm retrieval procedures allowed patients with azoospermia to conceive using testicular sperm. In many occasions, the motility of testicular sperm may be altered causing difficulty in selecting viable sperm for ICSI. PF can stimulate motility of testicular sperm, allow easier identification of vital sperm, shorten the procedure, improve fertilization rates, and increase the number of embryos that can be used for ICSI.
It has always been puzzling to realize that the single molecule supporting aerobic life, oxygen, is not just essential for survival, but is also involved in the pathophysiology of numerous diseases and degenerative states due to OS resulting from ROS generation. This is due to the very nature of the element oxygen as it in itself is a reactive biradical which will form highly reactive intermediates. The damaging effects of ROS is prevented due to the counterbalancing effect of the body's enzymatic and non-enzymatic antioxidant systems. The antioxidant supplementation has been proven to offer a protective effect against OS. Nonetheless, the uncontrolled or exaggerated use of antioxidants should be discouraged to avoid a condition called “antioxidant paradox” leading to ‘reductive stress’ [136], which is as dangerous for cells as OS [107137138139] as it can also be the cause of various diseases and infertility. Henkel [107] and Chen et al [139] highlighted this problem as over-dosage of patients might result in reductive stress and infertility as antioxidants have the potential to cause both, positive and seriously harmful effects on male fertility and wellbeing. It is believed that an overdose of antioxidants may disrupt the fine bodily balance between oxidation and reduction. One example is the increase in sperm DNA decondensation that has been reported to occur with exaggerated use of supra-physiologic doses of antioxidants [140141]. Such a condition may induce chromosomal abnormalities, lead to cytoplasmic fragments in the embryo and be deleterious for early development.
Excessive ROS resulting in OS can have a serious negative impact on the outcome of ART. Since the generation of ROS during the course of ART cannot be completely avoided, strategies that can minimize their detrimental effects on ART outcomes should be utilized. The in vitro use of antioxidants in the clinical laboratory setting during ART procedures should be considered, along with the constant evolvement and optimization of the laboratory techniques and environment. Several antioxidants are available with varying evidence of benefit. The identification of best antioxidant molecule and its optimal dosage suitable in different forms of ART remain an area of ongoing research. In addition, a thorough evaluation of the bodily redox potential is crucial for proper diagnosis and treatment of patients with antioxidants. However, as long as scientists and clinicians do not know what the normal redox potential in the male reproductive tract and in semen is, it will be difficult to suggest a rational evidence-based antioxidant therapy. Recently researchers at the Cleveland Clinic's American Center for Reproductive Medicine have established an oxidation-reduction potential assay (ORP) using a novel galvanostat-based technology as an effective method for measuring OS in semen and distinguishing normal men from male factor infertility patients. ORP captures a reliable, complete, and rapid picture of OS in a given semen sample, providing a functional component not contained within the semen analysis. Identification of abnormal levels of OS can enhance clinical understanding related to poor sperm function, especially in idiopathic and unexplained male infertility cases, and help optimize treatment strategies for male factor infertility [142143144145].
References
1. Sharlip ID, Jarow JP, Belker AM, Lipshultz LI, Sigman M, Thomas AJ, et al. Best practice policies for male infertility. Fertil Steril. 2002; 77:873–882. PMID: 12009338.


2. Zhu T. In vitro fertilization [Internet]. Arizona: Embryo Project Encyclopedia;c2009. cited 2017 Mar 14. Available from: http://embryo.asu.edu/handle/10776/1665.
3. Brezina PR, Zhao Y. The ethical, legal, and social issues impacted by modern assisted reproductive technologies. Obstet Gynecol Int. 2012; 2012:686253. PMID: 22272208.


4. Agarwal A, Saleh RA, Bedaiwy MA. Role of reactive oxygen species in the pathophysiology of human reproduction. Fertil Steril. 2003; 79:829–843. PMID: 12749418.


5. Agarwal A, Virk G, Ong C, du Plessis SS. Effect of oxidative stress on male reproduction. World J Mens Health. 2014; 32:1–17. PMID: 24872947.


6. Zini A, Al-Hathal N. Antioxidant therapy in male infertility: fact or fiction? Asian J Androl. 2011; 13:374–381. PMID: 21516118.


7. Agarwal A, Majzoub A. Role of antioxidants in male infertility. BJUI. 2016; DOI: 10.18591/BJUIK.0510.


8. Halliwell B. Free radicals and vascular disease: how much do we know? BMJ. 1993; 307:885–886. PMID: 8241848.


9. de Lamirande E, Jiang H, Zini A, Kodama H, Gagnon C. Reactive oxygen species and sperm physiology. Rev Reprod. 1997; 2:48–54. PMID: 9414465.


10. Aitken RJ, Paterson M, Fisher H, Buckingham DW, van Duin M. Redox regulation of tyrosine phosphorylation in human spermatozoa and its role in the control of human sperm function. J Cell Sci. 1995; 108:2017–2025. PMID: 7544800.


11. de Lamirande E, Gagnon C. Impact of reactive oxygen species on spermatozoa: a balancing act between beneficial and detrimental effects. Hum Reprod. 1995; 10(Suppl 1):15–21. PMID: 8592032.


12. Zini A, De Lamirande E, Gagnon C. Low levels of nitric oxide promote human sperm capacitation in vitro. J Androl. 1995; 16:424–431. PMID: 8575982.
14. Agarwal A, Said TM, Bedaiwy MA, Banerjee J, Alvarez JG. Oxidative stress in an assisted reproductive techniques setting. Fertil Steril. 2006; 86:503–512. PMID: 16860798.


15. du Plessis SS, Makker K, Desai NR, Agarwal A. Impact of oxidative stress on IVF. Expet Rev Obstet Gynecol. 2008; 3:539–554.


16. Agarwal A, Makker K, Sharma R. Clinical relevance of oxidative stress in male factor infertility: an update. Am J Reprod Immunol. 2008; 59:2–11. PMID: 18154591.
17. Agarwal A, Majzoub A. Free radicals in andrology. In : Balercia G, Gandini L, Lenzi A, Lombardo F, editors. Antioxidants in andrology. Cham: Springer International Publishing;2017. p. 1–21.
18. Gil-Guzman E, Ollero M, Lopez MC, Sharma RK, Alvarez JG, Thomas AJ Jr, et al. Differential production of reactive oxygen species by subsets of human spermatozoa at different stages of maturation. Hum Reprod. 2001; 16:1922–1930. PMID: 11527899.


19. Tanphaichitr N, Kongmanas K, Kruevaisayawan H, Saewu A, Sugeng C, Fernandes J, et al. Remodeling of the plasma membrane in preparation for sperm-egg recognition: roles of acrosomal proteins. Asian J Androl. 2015; 17:574–582. PMID: 25994642.


20. Aitken RJ, Baker MA. Reactive oxygen species generation by human spermatozoa: a continuing enigma. Int J Androl. 2002; 25:191–194. PMID: 12121567.


21. Schatten H, Sun QY, Prather R. The impact of mitochondrial function/dysfunction on IVF and new treatment possibilities for infertility. Reprod Biol Endocrinol. 2014; 12:111. PMID: 25421171.


22. Sandoval JS, Raburn D, Mausher S. Leukocytospermia: overview of diagnosis, implications, and management of a controversial finding. Middle East Fertil Soc J. 2013; 18:129–134.


23. Henkel R, Schill WB. Sperm separation in patients with urogenital infections. Andrologia. 1998; 30(Suppl 1):91–97.


24. Lobascio AM, De Felici M, Anibaldi M, Greco P, Minasi MG, Greco E. Involvement of seminal leukocytes, reactive oxygen species, and sperm mitochondrial membrane potential in the DNA damage of the human spermatozoa. Andrology. 2015; 3:265–270. PMID: 25598385.


25. Henkel R, Kierspel E, Stalf T, Mehnert C, Menkveld R, Tinneberg HR, et al. Effect of reactive oxygen species produced by spermatozoa and leukocytes on sperm functions in non-leukocytospermic patients. Fertil Steril. 2005; 83:635–642. PMID: 15749492.


26. Saleh RA, Agarwal A, Kandirali E, Sharma RK, Thomas AJ, Nada EA, et al. Leukocytospermia is associated with increased reactive oxygen species production by human spermatozoa. Fertil Steril. 2002; 78:1215–1224. PMID: 12477515.


27. Mupfiga C, Fisher D, Kruger T, Henkel R. The relationship between seminal leukocytes, oxidative status in the ejaculate, and apoptotic markers in human spermatozoa. Syst Biol Reprod Med. 2013; 59:304–311. PMID: 23898825.


28. Seino T, Saito H, Kaneko T, Takahashi T, Kawachiya S, Kurachi H. Eight-hydroxy-2′-deoxyguanosine in granulosa cells is correlated with the quality of oocytes and embryos in an in vitro fertilization-embryo transfer program. Fertil Steril. 2002; 77:1184–1190. PMID: 12057726.


29. Lampiao F. Free radicals generation in an in vitro fertilization setting and how to minimize them. World J Obstet Gynecol. 2012; 1:29–34.
30. Bedaiwy MA, Falcone T, Mohamed MS, Aleem AA, Sharma RK, Worley SE, et al. Differential growth of human embryos in vitro: role of reactive oxygen species. Fertil Steril. 2004; 82:593–600. PMID: 15374701.


31. Rakhit M, Gokul SR, Agarwal A, du Plessis SS. Antioxidant strategies to overcome OS in IVF-embryo transfer. In : Agarwal A, Azia N, Rizk B, editors. Studies on women's health. New York: Humana Press;2013. p. 237–262.
32. Agarwal A, Said TM. Role of sperm chromatin abnormalities and DNA damage in male infertility. Hum Reprod Update. 2003; 9:331–345. PMID: 12926527.


33. Gandini L, Lombardo F, Paoli D, Caponecchia L, Familiari G, Verlengia C, et al. Study of apoptotic DNA fragmentation in human spermatozoa. Hum Reprod. 2000; 15:830–839. PMID: 10739828.


34. Bedaiwy MA, Falcone T, Sharma RK, Goldberg JM, Attaran M, Nelson DR, et al. Prediction of endometriosis with serum and peritoneal fluid markers: a prospective controlled trial. Hum Reprod. 2002; 17:426–431. PMID: 11821289.


35. Bavister B. Oxygen concentration and preimplantation development. Reprod Biomed Online. 2004; 9:484–486. PMID: 15588462.


36. Kasterstein E, Strassburger D, Komarovsky D, Bern O, Komsky A, Raziel A, et al. The effect of two distinct levels of oxygen concentration on embryo development in a sibling oocyte study. J Assist Reprod Genet. 2013; 30:1073–1079. PMID: 23835722.


37. Beehler BC, Przybyszewski J, Box HB, Kulesz-Martin MF. Formation of 8-hydroxydeoxyguanosine within DNA of mouse keratinocytes exposed in culture to UVB and H2O2. Carcinogenesis. 1992; 13:2003–2007. PMID: 1423868.


38. Takenaka M, Horiuchi T, Yanagimachi R. Effects of light on development of mammalian zygotes. Proc Natl Acad Sci U S A. 2007; 104:14289–14293. PMID: 17709739.


39. Girotti AW. Photosensitized oxidation of membrane lipids: reaction pathways, cytotoxic effects, and cytoprotective mechanisms. J Photochem Photobiol B. 2001; 63:103–113. PMID: 11684457.


40. Guérin P, El Mouatassim S, Ménézo Y. Oxidative stress and protection against reactive oxygen species in the preimplantation embryo and its surroundings. Hum Reprod Update. 2001; 7:175–189. PMID: 11284661.
41. Kehrer JP. The Haber-Weiss reaction and mechanisms of toxicity. Toxicology. 2000; 149:43–50. PMID: 10963860.


42. Nasr-Esfahani MH, Johnson MH. How does transferrin overcome the in vitro block to development of the mouse preimplantation embryo? J Reprod Fertil. 1992; 96:41–48. PMID: 1432973.


43. Will MA, Clark NA, Swain JE. Biological pH buffers in IVF: help or hindrance to success. J Assist Reprod Genet. 2011; 28:711–724. PMID: 21614519.


44. Suzuki N, Mittler R. Reactive oxygen species and temperature stresses: a delicate balance between signaling and destruction. Physiol Plant. 2006; 126:45–51.


45. Ferguson WJ, Braunschweiger KI, Braunschweiger WR, Smith JR, McCormick JJ, Wasmann CC, et al. Hydrogen ion buffers for biological research. Anal Biochem. 1980; 104:300–310. PMID: 7446957.


46. Larkindale J, Knight MR. Protection against heat stress-induced oxidative damage in Arabidopsis involves calcium, abscisic acid, ethylene, and salicylic acid. Plant Physiol. 2002; 128:682–695. PMID: 11842171.


47. Shekarriz M, DeWire DM, Thomas AJ Jr, Agarwal A. A method of human semen centrifugation to minimize the iatrogenic sperm injuries caused by reactive oxygen species. Eur Urol. 1995; 28:31–35. PMID: 8521891.
48. Henkel RR, Schill WB. Sperm preparation for ART. Reprod Biol Endocrinol. 2003; 1:108. PMID: 14617368.
50. Di Santo M, Tarozzi N, Nadalini M, Borini A. Human sperm cryopreservation: update on techniques, effect on DNA integrity, and implications for ART. Adv Urol. 2012; 2012:854837. PMID: 22194740.


51. Alvarez JG, Storey BT. Evidence for increased lipid peroxidative damage and loss of superoxide dismutase activity as a mode of sublethal cryodamage to human sperm during cryopreservation. J Androl. 1992; 13:232–241. PMID: 1601742.
52. Thomson LK, Fleming SD, Aitken RJ, De Iuliis GN, Zieschang JA, Clark AM. Cryopreservation-induced human sperm DNA damage is predominantly mediated by oxidative stress rather than apoptosis. Hum Reprod. 2009; 24:2061–2070. PMID: 19525298.


53. Zribi N, Feki Chakroun N, El Euch H, Gargouri J, Bahloul A, Ammar Keskes L. Effects of cryopreservation on human sperm deoxyribonucleic acid integrity. Fertil Steril. 2010; 93:159–166. PMID: 19027111.


54. Hammadeh ME, Al Hasani S, Rosenbaum P, Schmidt W, Fischer Hammadeh C. Reactive oxygen species, total antioxidant concentration of seminal plasma and their effect on sperm parameters and outcome of IVF/ICSI patients. Arch Gynecol Obstet. 2008; 277:515–526. PMID: 18026972.


55. Ozatik O, Aydin Y, Hassa H, Ulusoy D, Ogut S, Sahin F. Relationship between oxidative stress and clinical pregnancy in assisted reproductive technology treatment cycles. J Assist Reprod Genet. 2013; 30:765–772. PMID: 23666546.


56. Cooper TG, Noonan E, von Eckardstein S, Auger J, Baker HW, Behre HM, et al. World Health Organization reference values for human semen characteristics. Hum Reprod Update. 2010; 16:231–245. PMID: 19934213.


57. Foote RH, Brockett CC, Kaproth MT. Motility and fertility of bull sperm in whole milk extender containing antioxidants. Anim Reprod Sci. 2002; 71:13–23. PMID: 11988368.


58. Zribi N, Chakroun NF, Ben Abdallah F, Elleuch H, Sellami A, Gargouri J, et al. Effect of freezing-thawing process and quercetin on human sperm survival and DNA integrity. Cryobiology. 2012; 65:326–331. PMID: 23010483.


59. Breininger E, Beorlegui NB, O'Flaherty CM, Beconi MT. Alpha-tocopherol improves biochemical and dynamic parameters in cryopreserved boar semen. Theriogenology. 2005; 63:2126–2135. PMID: 15826678.


60. Moubasher AE, El Din AM, Ali ME, El-sherif WT, Gaber HD. Catalase improves motility, vitality and DNA integrity of cryopreserved human spermatozoa. Andrologia. 2013; 45:135–139. PMID: 22591546.


61. Amália PM, Possa MN, Augusto MC, Francisca LS. Quercetin prevents oxidative stress in cirrhotic rats. Dig Dis Sci. 2007; 52:2616–2621. PMID: 17431769.


62. Shaik YB, Castellani ML, Perrella A, Conti F, Salini V, Tete S, et al. Role of quercetin (a natural herbal compound) in allergy and inflammation. J Biol Regul Homeost Agents. 2006; 20:47–52. PMID: 18187018.
63. Gibb Z, Butler TJ, Morris LH, Maxwell WM, Grupen CG. Quercetin improves the postthaw characteristics of cryopreserved sex-sorted and nonsorted stallion sperm. Theriogenology. 2013; 79:1001–1009. PMID: 23453253.


64. Chelikani P, Fita I, Loewen PC. Diversity of structures and properties among catalases. Cell Mol Life Sci. 2004; 61:192–208. PMID: 14745498.


65. Willcox JK, Ash SL, Catignani GL. Antioxidants and prevention of chronic disease. Crit Rev Food Sci Nutr. 2004; 44:275–295. PMID: 15462130.


66. Branco CS, Garcez ME, Pasqualotto FF, Erdtman B, Salvador M. Resveratrol and ascorbic acid prevent DNA damage induced by cryopreservation in human semen. Cryobiology. 2010; 60:235–237. PMID: 19895799.


67. Garcez ME, dos Santos Branco C, Lara LV, Pasqualotto FF, Salvador M. Effects of resveratrol supplementation on cryopreservation medium of human semen. Fertil Steril. 2010; 94:2118–2121. PMID: 20189559.


68. Bucak MN, Ataman MB, Başpınar N, Uysal O, Taşpınar M, Bilgili A, et al. Lycopene and resveratrol improve post-thaw bull sperm parameters: sperm motility, mitochondrial activity and DNA integrity. Andrologia. 2015; 47:545–552. PMID: 24909239.


69. Silva EC, Cajueiro JF, Silva SV, Soares PC, Guerra MM. Effect of antioxidants resveratrol and quercetin on in vitro evaluation of frozen ram sperm. Theriogenology. 2012; 77:1722–1726. PMID: 22289215.


70. Kalthur G, Raj S, Thiyagarajan A, Kumar S, Kumar P, Adiga SK. Vitamin E supplementation in semen-freezing medium improves the motility and protects sperm from freeze-thaw-induced DNA damage. Fertil Steril. 2011; 95:1149–1151. PMID: 21067726.


71. Taylor K, Roberts P, Sanders K, Burton P. Effect of antioxidant supplementation of cryopreservation medium on post-thaw integrity of human spermatozoa. Reprod Biomed Online. 2009; 18:184–189. PMID: 19192337.


72. Noack-Füller G, De Beer C, Seibert H. Cadmium, lead, selenium, and zinc in semen of occupationally unexposed men. Andrologia. 1993; 25:7–12. PMID: 8427423.


73. Siegel RB, Murray FA, Julien WE, Moxon AL, Conrad HR. Effect of in vitro selenium supplementation on bovine sperm motility. Theriogenology. 1980; 13:357–367. PMID: 16725505.
74. Dorostkar K, Alavi-Shoushtari SM, Mokarizadeh A. Effects of in vitro selenium addition to the semen extender on the spermatozoa characteristics before and after freezing in water buffaloes (Bubalus bubalis). Vet Res Forum. 2012; 3:263–268. PMID: 25653769.
75. Geva E, Bartoov B, Zabludovsky N, Lessing JB, Lerner-Geva L, Amit A. The effect of antioxidant treatment on human spermatozoa and fertilization rate in an in vitro fertilization program. Fertil Steril. 1996; 66:430–434. PMID: 8751743.


76. Suleiman SA, Ali ME, Zaki ZM, el-Malik EM, Nasr MA. Lipid peroxidation and human sperm motility: protective role of vitamin E. J Androl. 1996; 17:530–537. PMID: 8957697.
77. Sierens J, Hartley JA, Campbell MJ, Leathem AJ, Woodside JV. In vitro isoflavone supplementation reduces hydrogen peroxide-induced DNA damage in sperm. Teratog Carcinog Mutagen. 2002; 22:227–234. PMID: 11948633.


78. Aitken RJ, Clarkson JS. Significance of reactive oxygen species and antioxidants in defining the efficacy of sperm preparation techniques. J Androl. 1988; 9:367–376. PMID: 3215823.


79. Jacob RA, Pianalto FS, Agee RE. Cellular ascorbate depletion in healthy men. J Nutr. 1992; 122:1111–1118. PMID: 1564563.


80. Fraga CG, Motchnik PA, Shigenaga MK, Helbock HJ, Jacob RA, Ames BN. Ascorbic acid protects against endogenous oxidative DNA damage in human sperm. Proc Natl Acad Sci U S A. 1991; 88:11003–11006. PMID: 1763015.


81. Lewis SE, John Aitken R, Conner SJ, Iuliis GD, Evenson DP, Henkel R, et al. The impact of sperm DNA damage in assisted conception and beyond: recent advances in diagnosis and treatment. Reprod Biomed Online. 2013; 27:325–337. PMID: 23948450.


82. Dawson EB, Harris WA, Teter MC, Powell LC. Effect of ascorbic acid supplementation on the sperm quality of smokers. Fertil Steril. 1992; 58:1034–1039. PMID: 1426355.
83. Thiele JJ, Friesleben HJ, Fuchs J, Ochsendorf FR. Ascorbic acid and urate in human seminal plasma: determination and interrelationships with chemiluminescence in washed semen. Hum Reprod. 1995; 10:110–115. PMID: 7745037.


84. Greco E, Iacobelli M, Rienzi L, Ubaldi F, Ferrero S, Tesarik J. Reduction of the incidence of sperm DNA fragmentation by oral antioxidant treatment. J Androl. 2005; 26:349–353. PMID: 15867002.
85. Tremellen K, Miari G, Froiland D, Thompson J. A randomised control trial examining the effect of an antioxidant (Menevit) on pregnancy outcome during IVF-ICSI treatment. Aust N Z J Obstet Gynaecol. 2007; 47:216–221. PMID: 17550489.


86. Verma A, Kanwar KC. Human sperm motility and lipid peroxidation in different ascorbic acid concentrations: an in vitro analysis. Andrologia. 1998; 30:325–329. PMID: 9835946.


87. Wang X, Sharma RK, Gupta A, George V, Thomas AJ, Falcone T, et al. Alterations in mitochondria membrane potential and oxidative stress in infertile men: a prospective observational study. Fertil Steril. 2003; 80(Suppl 2):844–850. PMID: 14505763.


88. Mongioi L, Calogero AE, Vicari E, Condorelli RA, Russo GI, Privitera S, et al. The role of carnitine in male infertility. Andrology. 2016; 4:800–807. PMID: 27152678.


89. Lenzi A, Sgrò P, Salacone P, Paoli D, Gilio B, Lombardo F, et al. A placebo-controlled double-blind randomized trial of the use of combined l-carnitine and l-acetyl-carnitine treatment in men with asthenozoospermia. Fertil Steril. 2004; 81:1578–1584. PMID: 15193480.


90. Balercia G, Regoli F, Armeni T, Koverech A, Mantero F, Boscaro M. Placebo-controlled double-blind randomized trial on the use of L-carnitine, L-acetylcarnitine, or combined L-carnitine and L-acetylcarnitine in men with idiopathic asthenozoospermia. Fertil Steril. 2005; 84:662–671. PMID: 16169400.


91. Cavallini G, Ferraretti AP, Gianaroli L, Biagiotti G, Vitali G. Cinnoxicam and L-carnitine/acetyl-L-carnitine treatment for idiopathic and varicocele-associated oligoasthenospermia. J Androl. 2004; 25:761–770. discussion 771-2. PMID: 15292108.
92. Moslemi Mehni N, Ketabchi AA, Hosseini E. Combination effect of Pentoxifylline and L-carnitine on idiopathic oligo-asthenoteratozoospermia. Iran J Reprod Med. 2014; 12:817–824. PMID: 25709639.
93. Al-Dujaily SS, Al-Sultani YK, Shams Alddin NN. DNA normality following in vitro sperm preparation with pentoxifylline and L-Carnitine for asthenozoospermic infertile men. Glob J Med Res. 2013; 13:25–30.
94. Lewin A, Lavon H. The effect of coenzyme Q10 on sperm motility and function. Mol Aspects Med. 1997; 18(Suppl):S213–S219. PMID: 9266524.


95. Nadjarzadeh A, Shidfar F, Amirjannati N, Vafa MR, Motevalian SA, Gohari MR, et al. Effect of Coenzyme Q10 supplementation on antioxidant enzymes activity and oxidative stress of seminal plasma: a double-blind randomised clinical trial. Andrologia. 2014; 46:177–183. PMID: 23289958.


96. Safarinejad MR. Efficacy of coenzyme Q10 on semen parameters, sperm function and reproductive hormones in infertile men. J Urol. 2009; 182:237–248. PMID: 19447425.


97. Safarinejad MR. The effect of coenzyme Q10 supplementation on partner pregnancy rate in infertile men with idiopathic oligoasthenoteratozoospermia: an open-label prospective study. Int Urol Nephrol. 2012; 44:689–700. PMID: 22081410.


98. Giacone F, Condorelli RA, Mongioì LM, Bullara V, La Vignera S, Calogero AE. In vitro effects of zinc, D-aspartic acid, and coenzyme-Q10 on sperm function. Endocrine. 2016; DOI: 10.1007/s12020-016-1013-7. [Epub].


99. Mora-Esteves C, Shin D. Nutrient supplementation: improving male fertility fourfold. Semin Reprod Med. 2013; 31:293–300. PMID: 23775385.


100. Lanzafame FM, La Vignera S, Vicari E, Calogero AE. Oxidative stress and medical antioxidant treatment in male infertility. Reprod Biomed Online. 2009; 19:638–659. PMID: 20021713.


101. Lenzi A, Picardo M, Gandini L, Lombardo F, Terminali O, Passi S, et al. Glutathione treatment of dyspermia: effect on the lipoperoxidation process. Hum Reprod. 1994; 9:2044–2050. PMID: 7868672.
102. Oeda T, Henkel R, Ohmori H, Schill WB. Scavenging effect of N-acetyl-L-cysteine against reactive oxygen species in human semen: a possible therapeutic modality for male factor infertility? Andrologia. 1997; 29:125–131. PMID: 9197915.


103. Ciftci H, Verit A, Savas M, Yeni E, Erel O. Effects of N-acetylcysteine on semen parameters and oxidative/antioxidant status. Urology. 2009; 74:73–76. PMID: 19428083.


104. Aitken RJ, Gibb Z, Mitchell LA, Lambourne SR, Connaughton HS, De Iuliis GN. Sperm motility is lost in vitro as a consequence of mitochondrial free radical production and the generation of electrophilic aldehydes but can be significantly rescued by the presence of nucleophilic thiols. Biol Reprod. 2012; 87:110. PMID: 22933515.


105. Erkkilä K, Hirvonen V, Wuokko E, Parvinen M, Dunkel L. N-acetyl-L-cysteine inhibits apoptosis in human male germ cells in vitro. J Clin Endocrinol Metab. 1998; 83:2523–2531. PMID: 9661638.
106. Castagné V, Lefèvre K, Natero R, Clarke PG, Bedker DA. An optimal redox status for the survival of axotomized ganglion cells in the developing retina. Neuroscience. 1999; 93:313–320. PMID: 10430495.


107. Henkel RR. Leukocytes and oxidative stress: dilemma for sperm function and male fertility. Asian J Androl. 2011; 13:43–52. PMID: 21076433.


108. Agarwal A, Majzoub A, Esteves SC, Ko E, Ramasamy R, Zini A. Clinical utility of sperm DNA fragmentation testing: practice recommendations based on clinical scenarios. Transl Androl Urol. 2016; 5:935–950. PMID: 28078226.


109. López G, Lafuente R, Checa MA, Carreras R, Brassesco M. Diagnostic value of sperm DNA fragmentation and sperm high-magnification for predicting outcome of assisted reproduction treatment. Asian J Androl. 2013; 15:790–794. PMID: 23912311.


110. Luna D, Hilario R, Dueñas-Chacón J, Romero R, Zavala P, Villegas L, et al. The IMSI procedure improves laboratory and clinical outcomes without compromising the aneuploidy rate when compared to the classical ICSI procedure. Clin Med Insights Reprod Health. 2015; 9:29–37. PMID: 26609251.


111. Kodama H, Yamaguchi R, Fukuda J, Kasai H, Tanaka T. Increased oxidative deoxyribonucleic acid damage in the spermatozoa of infertile male patients. Fertil Steril. 1997; 68:519–524. PMID: 9314926.


112. Greco E, Romano S, Iacobelli M, Ferrero S, Baroni E, Minasi MG, et al. ICSI in cases of sperm DNA damage: beneficial effect of oral antioxidant treatment. Hum Reprod. 2005; 20:2590–2594. PMID: 15932912.


113. Abad C, Amengual MJ, Gosálvez J, Coward K, Hannaoui N, Benet J, et al. Effects of oral antioxidant treatment upon the dynamics of human sperm DNA fragmentation and subpopulations of sperm with highly degraded DNA. Andrologia. 2013; 45:211–216. PMID: 22943406.


114. Hughes CM, Lewis SE, McKelvey-Martin VJ, Thompson W. The effects of antioxidant supplementation during Percoll preparation on human sperm DNA integrity. Hum Reprod. 1998; 13:1240–1247. PMID: 9647554.


115. Boxmeer JC, Smit M, Utomo E, Romijn JC, Eijkemans MJ, Lindemans J, et al. Low folate in seminal plasma is associated with increased sperm DNA damage. Fertil Steril. 2009; 92:548–556. PMID: 18722602.


116. Swayne BG, Kawata A, Behan NA, Williams A, Wade MG, Macfarlane AJ, et al. Investigating the effects of dietary folic acid on sperm count, DNA damage and mutation in Balb/c mice. Mutat Res. 2012; 737:1–7. PMID: 22824165.


117. da Silva TM, Silva Maia MC, Arruda JT, Approbato FC, Mendonça RC, Approbato MS. Folic acid does not improve semen parametrs in subfertile men: a double-blin, randomized, placebo-controlled study. JBRA Assist Reprod. 2013; 17:152–157.


118. Raigani M, Yaghmaei B, Amirjannti N, Lakpour N, Akhondi MM, Zeraati H, et al. The micronutrient supplements, zinc sulphate and folic acid, did not ameliorate sperm functional parameters in oligoasthenoteratozoospermic men. Andrologia. 2014; 46:956–962. PMID: 24147895.


119. Lee VM, Wong JS, Loh SK, Leong NK. Sperm motility in the semen analysis affects the outcome of superovulation intrauterine insemination in the treatment of infertile Asian couples with male factor infertility. BJOG. 2002; 109:115–120. PMID: 11905427.


120. Attallah D, El-Nashar IH, Mahmoud R, Shaaban OM, Salman SA. N-acytelcysteine prior to intrauterine insemination in couples with isolated athenozospermia: a randomized controlled trial. Fertil Steril. 2013; 100:S462.


121. Peivandi S, Karimpour A, Moslemizadeh N. Effects of L-carnitine on infertile men's spermogram; a randomized clinical trial. J Reprod Infertil. 2010; 10:245–251.
122. Eskenazi B, Kidd SA, Marks AR, Sloter E, Block G, Wyrobek AJ. Antioxidant intake is associated with semen quality in healthy men. Hum Reprod. 2005; 20:1006–1012. PMID: 15665024.


123. Fernandez CD, Porto EM, Arena AC, Kempinas Wde G. Effects of altered epididymal sperm transit time on sperm quality. Int J Androl. 2008; 31:427–437. PMID: 17822422.


124. Wood S, Sephton V, Searle T, Thomas K, Schnauffer K, Troup S, et al. Effect on clinical outcome of the interval between collection of epididymal and testicular spermatozoa and intracytoplasmic sperm injection in obstructive azoospermia. J Androl. 2003; 24:67–72. PMID: 12514085.
125. Verheyen G, Joris H, Crits K, Nagy Z, Tournaye H, Van Steirteghem A. Comparison of different hypo-osmotic swelling solutions to select viable immotile spermatozoa for potential use in intracytoplasmic sperm injection. Hum Reprod Update. 1997; 3:195–203. PMID: 9322097.


126. Dalzell LH, McVicar CM, McClure N, Lutton D, Lewis SE. Effects of short and long incubations on DNA fragmentation of testicular sperm. Fertil Steril. 2004; 82:1443–1445. PMID: 15533376.


127. Nabi A, Khalili MA, Halvaei I, Roodbari F. Prolonged incubation of processed human spermatozoa will increase DNA fragmentation. Andrologia. 2014; 46:374–379. PMID: 24689689.


128. Dalzell LH, Thompson-Cree ME, McClure N, Traub AI, Lewis SE. Effects of 24-hour incubation after freeze-thawing on DNA fragmentation of testicular sperm from infertile and fertile men. Fertil Steril. 2003; 79(Suppl 3):1670–1672. PMID: 12801581.


129. Salhiyyah K, Senanayake E, Abdel-Hadi M, Booth A, Michaels JA. Pentoxifylline for intermittent claudication. Cochrane Database Syst Rev. 2012; 1:CD005262. PMID: 22258961.


130. Aparicio NJ, de Turner EA, Schwarzstein L, Turner D. Effect of the phosphodiesterase inhibitor Pentoxyfylline on human sperm motility. Andrologia. 1980; 12:49–54. PMID: 7377553.


131. Tournaye H, Janssens R, Devroey P, van Steirteghem A. The influence of pentoxifylline on motility and viability of spermatozoa from normozoospermic semen samples. Int J Androl. 1994; 17:1–8. PMID: 8005702.


132. Kovacic B, Vlaisavljevic V, Reljic M. Clinical use of pentoxifylline for activation of immotile testicular sperm before ICSI in patients with azoospermia. J Androl. 2006; 27:45–52. PMID: 16400077.


133. Griveau JF, Lobel B, Laurent MC, Michardière L, Le Lannou D. Interest of pentoxifylline in ICSI with frozen-thawed testicular spermatozoa from patients with non-obstructive azoospermia. Reprod Biomed Online. 2006; 12:14–18. PMID: 16454927.


134. Taşdemir I, Taşdemir M, Tavukçuoğlu S. Effect of pentoxifylline on immotile testicular spermatozoa. J Assist Reprod Genet. 1998; 15:90–92. PMID: 9513848.


135. Terriou P, Hans E, Giorgetti C, Spach JL, Salzmann J, Urrutia V, et al. Pentoxifylline initiates motility in spontaneously immotile epididymal and testicular spermatozoa and allows normal fertilization, pregnancy, and birth after intracytoplasmic sperm injection. J Assist Reprod Genet. 2000; 17:194–199. PMID: 10955242.
136. Wendel A. Measurement of in vivo lipid peroxidation and toxicological significance. Free Radic Biol Med. 1987; 3:355–358. PMID: 3319801.


137. Ufer C, Wang CC, Borchert A, Heydeck D, Kuhn H. Redox control in mammalian embryo development. Antioxid Redox Signal. 2010; 13:833–875. PMID: 20367257.


138. Naviaux RK. Oxidative shielding or oxidative stress? J Pharmacol Exp Ther. 2012; 342:608–618. PMID: 22700427.


139. Chen SJ, Allam JP, Duan YG, Haidl G. Influence of reactive oxygen species on human sperm functions and fertilizing capacity including therapeutical approaches. Arch Gynecol Obstet. 2013; 288:191–199. PMID: 23543240.


140. Ménézo YJ, Hazout A, Panteix G, Robert F, Rollet J, Cohen-Bacrie P, et al. Antioxidants to reduce sperm DNA fragmentation: an unexpected adverse effect. Reprod Biomed Online. 2007; 14:418–421. PMID: 17425820.


141. Giustarini D, Dalle-Donne I, Colombo R, Milzani A, Rossi R. Is ascorbate able to reduce disulfide bridges? A cautionary note. Nitric Oxide. 2008; 19:252–258. PMID: 18675931.


142. Agarwal A, Wang SM. Clinical relevance of oxidation-reduction potential in the evaluation of male infertility. Urology. 2017; DOI: 10.1016/j.urology.2017.02.016. [Epub].


143. Agarwal A, Roychoudhury S, Sharma R, Gupta S, Majzoub A, Sabanegh E. Diagnostic application of oxidation-reduction potential assay for measurement of oxidative stress: clinical utility in male factor infertility. Reprod Biomed Online. 2017; 34:48–57. PMID: 27839743.


144. Agarwal A, Roychoudhury S, Bjugstad KB, Cho CL. Oxidation-reduction potential of semen: what is its role in the treatment of male infertility? Ther Adv Urol. 2016; 8:302–318. PMID: 27695529.


145. Agarwal A, Sharma R, Roychoudhury S, Du Plessis S, Sabanegh E. MiOXSYS: a novel method of measuring oxidation reduction potential in semen and seminal plasma. Fertil Steril. 2016; 106:566–573. PMID: 27260688.


Fig. 1
Sources of reactive oxygen species (ROS) in the assisted reproduction setting. IVF-ET: in vitro fertilization-embryo transfer. Data from Cleveland Clinic Foundation (CCF) with CCF's permission.
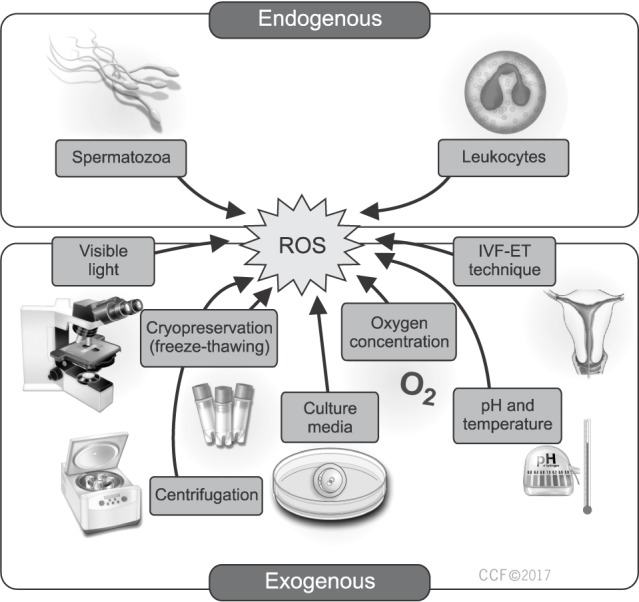
Table 1
Antioxidant use in the assisted reproduction setting
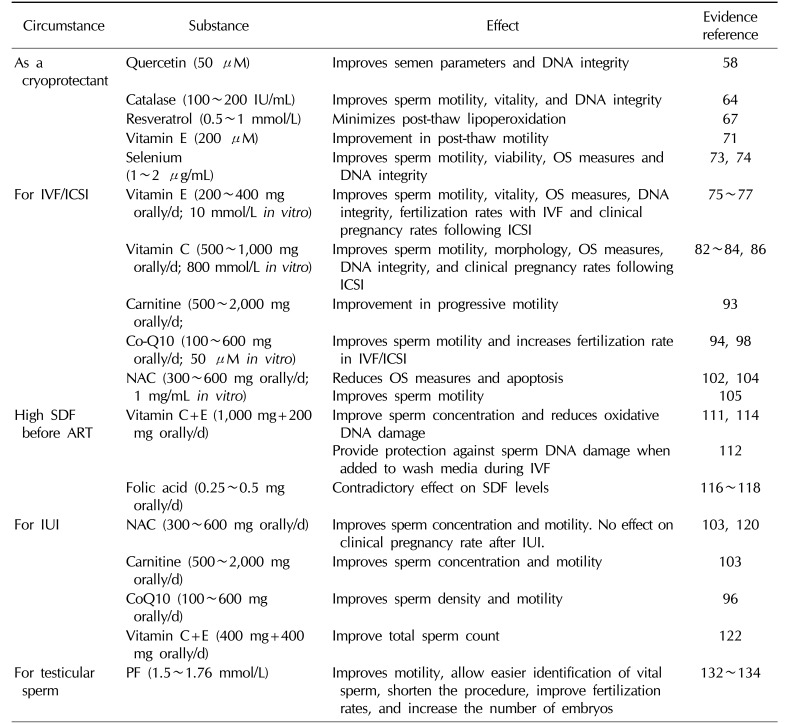