Abstract
Purpose
Pelvic irradiation for the treatment of cancer can affect normal cells, such as the rapidly proliferating spermatogenic cells of the testis, leading to infertility, a common post-irradiation problem. The present study investigated the radioprotective effect of rolipram, a specific phosphodiesterase type-IV inhibitor known to increase the expression and phosphorylation of the cyclic adenosine monophosphate response element-binding protein (CREB), a key factor for spermatogenesis, with the testicular system against pelvic irradiation.
Materials and Methods
Male C57BL/6 mice were treated with pelvic irradiation (2 Gy) and rolipram, alone or in combination, and were sacrificed at 12 hours and 35 days after irradiation.
Results
Rolipram protected germ cells from radiation-induced apoptosis at 12 hours after irradiation and significantly increased testis weight compared with irradiation controls at 35 days. Rolipram also ameliorated radiation-induced testicular morphological changes, such as changes in seminiferous tubular diameter and epithelial height. Additionally, seminiferous tubule repopulation and stem cell survival indices were higher in the rolipram-treated group than in the radiation group. Moreover, rolipram treatment counteracted the radiation-mediated decrease in the sperm count and mobility in the epididymis.
Conclusions
These protective effects of rolipram treatment prior to irradiation may be mediated by the increase in pCREB levels at 12 hours post-irradiation and the attenuated decrease in pCREB levels in the testis at 35 days post-irradiation in the rolipram-treated group. These findings suggest that activation of CREB signaling by rolipram treatment ameliorates the detrimental effects of acute irradiation on testicular dysfunction and the related male reproductive functions in mice.
The testis is one of the tissues most sensitive to the damaging effects of ionizing radiation. Low doses of radiation can cause considerable functional impairment of the testis [1,2]. Irradiation can affect normal cells, particularly rapidly proliferating ones such as spermatogenic cells, and the susceptibility of the testis to radiation-induced injury often leads to infertility, which is a common post-irradiation problem [3]. Such adverse events in the testis result in several abnormalities in spermatogenesis, which can cause temporary or permanent infertility. Examples include low sperm counts, an increase in abnormal spermatozoa, and defective sperm function [4]. Consequently, most men who receive irradiation are unable to reproduce later in life [4].
The cyclic adenosine monophosphate (cAMP) response element-binding protein (CREB), a hormone-responsive regulator of mammalian cell survival and growth [5], plays an important role in spermatogenesis [6,7]. However, whether CREB upregulation can protect against radiation-induced testicular injury or not is as yet unknown. Rolipram, a specific phosphodiesterase 4 inhibitor, is known to increase cAMP levels and stimulate the CREB pathway [8,9,10], which is involved in cAMP/CREB signaling and has been implicated in protection from various injury models [8,9,10,11,12,13,14,15,16]. In this study, we investigated whether rolipram has a protective effect on spermatogenesis following focal pelvic irradiation via the induction of CREB expression.
A 7- to 8-week-old male C57BL/6 mice, which were obtained from Central Lab Animal Inc. (Seoul, Korea), were housed in a room maintained at a temperature of 23℃±2℃, relative humidity of 50%±5%, artificial lighting from 08:00 to 20:00, and 13 to 18 air changes per hour. The mice were fed a standard animal diet. All animal experiments followed a protocol approved by the Institutional Animal Care and Use Committee of the Dongnam Institute of Radiological and Medical Sciences.
The mice were anesthetized with zoletil and immobilized at their extremities on a tray. Single fractions of 2 Gy were delivered at a specific depth through a distal pelvic field measuring 2×2 cm, which completely encompassed the two testes using 60Co gamma-rays (Elekta, Stockholm, Sweden) at a dose rate of 3.8 Gy/min. Sham-irradiated mice were treated in exactly the same manner as the irradiated animals.
The animals were randomly divided into four groups, each containing 10 mice: 1) sham-irradiated group, 2) rolipram group, 3) vehicle+irradiated group, and 4) rolipram+irradiation group. The vehicle group served as a control group and was given 0.9% saline solution intraperitoneally. Pelvic irradiation (2 Gy) was applied to the irradiated groups. Rolipram was administered at 1.25 mg/kg (Sigma-Aldrich, St. Louis, MO, USA) dissolved in saline containing 1% dimethyl sulfoxide immediately before irradiation by peritoneal injection twice daily for 5 consecutive days. This rolipram dose has been reported to be optimal for radioprotection [10]. The mice were sacrificed at 12 hours and 35 days after 2-Gy irradiation (n=5 mice per group), and the testis and epididymis were then dissected from each mouse.
To ascertain the anti-apoptotic effect of rolipram, the mice were sacrificed 12 hours after 2-Gy irradiation. Sections perpendicular to the long axis of the testis were made and stained using the terminal deoxynucleotidyl transferase dUTP-biotin nick end labeling (TUNEL) method of immunohistochemical staining with a commercial kit (ApopTag Plus™; Intergen, Burlington, MA, USA). At least 50 tubular cross sections were selected randomly from each section, and two sections from each animal were examined under a microscope as described previously [17,18].
To investigate the effect of rolipram on the histopathological parameters of spermiogenesis, the mice were sacrificed 35 days post-irradiation, and the testis and cauda epididymis were immediately removed and weighed. The right testis was fixed in Bouin's fluid solution for 2 days and embedded in paraffin by using a routine method. Sections perpendicular to the long axis of the testis were made and stained with H&E. The epithelial height and diameter of the seminiferous tubules were measured in the testis sections by using an ocular micrometer. At least 30 round tubules, excluding those on the edge of the testis, were measured in each mouse. The mean tubular diameter and the epithelial depth of these 30 tubules were calculated for each mouse. These mean values were then used to calculate the tubular diameter and the epithelial depth for each treatment group (n=5 per group).
The repopulation index (RI; percentage of repopulated tubules) was used for evaluating spermatogonial stem cell survival in each group. Tubules containing three or more spermatogenic cells that had reached type-B spermatogonia or later stages were considered repopulated. Approximately 200 seminiferous tubules were scored in each testis section. The stem cell survival index (SSI) was calculated from the RI by using the following equation: SSI=-[lne (1-RI÷100)]. The SSI was proportional to the number of surviving stem cells that were capable of producing colonies in a given tubule cross section. The SSI was not calculated when the RI was 100% [17,19].
For the immunohistochemical analysis, the fixed testis tissues were processed in paraffin, cut into 4-µm-thick coronal sections, and deparaffinized. The sections were hydrated and blocked in a normal goat serum (Vectastain Elite ABC kit; Vector Laboratories, Burlingame, CA, USA) for 60 minutes, and the testis sections were incubated with rabbit monoclonal anti-phosphorylated CREB (pCREB) (Ser133; 87G3; 1:200; Cell Signaling Technology, Beverly, MA, USA) and rabbit monoclonal anti-Ki-67 (DRM004; 1:200; Acris Antibodies GmbH, Herford, Germany) antibodies at 4℃ overnight. The sections were then reacted with biotinylated goat anti-rabbit immunoglobulin G (Vectastain Elite ABC kit). Immunoreactivity was performed using the avidin-biotin peroxidase complex (Vectastain Elite ABC kit). The peroxidase reaction was developed using a diaminobenzidine substrate kit (DAB Substrate Kit SK-4100; Vector Laboratories). The sections were counterstained with hematoxylin before being mounted.
Testicular tissue was minced, homogenized, lysed in buffer H (50 mM β-glycerophosphate, 1.5 mM ethylene glycol tetraacetic acid, 0.1 mM Na3VO4, 1 mM dithiothreitol, 10 µg/mL aprotinin, 2 µg/mL pepstatin, 10 µg/mL leupeptin, 1 mM phenylmethylsulfonyl fluoride, pH 7.4), and sonicated for 10 seconds. A sodium dodecyl sulfate (SDS) sample buffer (4×) was added to each homogenized sample, and the samples were heated at 100℃ for 10 minutes. The samples were then separated by 10% SDS-polyacrylamide gel electrophoresis, transferred to a polyvinylidene fluoride membrane, and blocked with 5% skim milk in phosphate-buffered saline (PBS) containing 0.1% Tween 20 (PBS-T) for 30 minutes at room temperature. The membranes were then incubated with rabbit monoclonal pCREB (Ser133; 87G3) or total CREB (tCREB) (48H2) antibodies (both at 1:1,000 dilution; Cell Signaling Technology) in PBS-T overnight at 4℃. After extensive washing and incubation with horseradish peroxidase-conjugated goat anti-rabbit IgG (1:20,000 dilution; Pierce, Rockford, IL, USA), the signals were visualized using chemiluminescence methods (SuperSignal West Pico; Pierce). After imaging, the membranes were stripped and reprobed with anti-β-actin antibody (1:20,000; Sigma-Aldrich) using a protocol similar to that described above. Several exposure times were used to obtain signals in a linear range. Band intensities were quantified using Scion Image Beta 4.0.2 for Windows XP software (Scion Corp., Frederick, MD, USA).
For sperm counts per cauda epididymis, the right cauda epididymis of each mouse was weighed, placed in 0.5-mL saline, and homogenized for 30 seconds. A 10-µL aliquot of each sample was diluted with diluent solution (0.25% trypan blue, 5% NaHCO3, 0.35% formalin), and the sperm were counted with a hemocytometer. The left cauda epididymis of each mouse was fixed in 10% buffered formalin for 2 days and embedded in paraffin by a routine method. Sections from different treatments were H&E stained and examined for changes to the cauda epididymis using an ocular micrometer.
In TUNEL-positive cells or bodies, the stained products correlated with the typical morphologic characteristics of apoptosis observed by optical microscopy (Fig. 1A). A small number of seminiferous germ cells exhibited apoptosis in the sham-irradiated mice. Irradiation increased the number of apoptotic nuclei in the germ cells of the mice, with most of the apoptotic cells being in the spermatogonia and primary spermatocyte (stage 12) stages in the seminiferous tubules. Pretreatment with rolipram before irradiation significantly (p<0.05) decreased the number of TUNEL-positive cells 12 hours post-irradiation compared with those in the irradiation control group (Fig. 1B).
No significant changes were observed in body weight between sham-irradiated or 2-Gy-irradiated mice 35 days post-irradiation with either vehicle or rolipram treatment (Fig. 2A). However, the testis weight was larger in the rolipram-treated group than in the vehicle-treated irradiated group at 35 days post-irradiation (Fig. 2B). Rolipram treatment prior to irradiation significantly (p<0.05) limited the decrease in testis weight per body weight with respect to vehicle-treated irradiated animals (Fig. 2C).
Irradiated testes exhibited a small seminiferous tubule diameter and epithelial depth. In mice treated with rolipram alone, the diameter of the seminiferous tubules was smaller than in the controls (sham), but was larger than in the irradiated groups at 35 days post-irradiation. Untreated mice showed a seminiferous tubular diameter of 118±4.6 µm and an epithelial height of 33.4±1.7 µm, while irradiation reduced the diameter by 61.7% and the height by 50.3% compared with the controls. Pretreatment with rolipram significantly increased the diameter (17%) and the height (30%) compared with the irradiated animals (Fig. 3; p=0.05 and p=0.04, respectively).
Irradiated testes contained fewer germinal cells at 35 days post-irradiation than the sham-irradiated group; however, the testes of the rolipram-pretreated mice were less altered than those of the irradiated animals (Fig. 3). A few tubules contained germinal cells, while other tubules were devoid of germinal cells with only Sertoli cells remaining in the irradiated group. Irradiation reduced RI from 100% to 83.6%±3.3% compared with the sham-irradiated control. Pretreatment with rolipram increased the RI to 91.0%±1.0% (p=0.06) compared with the irradiated-only group (Fig. 3). In the unirradiated groups, all the stem cells survived, producing an RI of 100%; hence, the SSI was not calculated, while pretreatment with rolipram increased the SSI by 29% (p<0.05) over the irradiated-only group.
Histoanalysis revealed that the epididymis of each mouse in the sham group was packed fully with sperm, while that of mice that had been irradiated (2 Gy) had a much lower sperm count. Rolipram treatment inhibited the radiation-induced decrease in the sperm count as compared to the vehicle-treated irradiation control group (Fig. 4A). Irradiation significantly reduced the number of sperm and sperm motility in the cauda epididymis 35 days post-irradiation compared with the sham controls (p<0.01). In contrast, rolipram pretreatment significantly attenuated the loss of sperm (p<0.01) and sperm motility (p<0.05) compared with the vehicle-treated mice 35 days post-irradiation (Fig. 4B).
Representative immunoblots of pCREB and tCREB expression 12 hours and 35 days following 2-Gy pelvic irradiation are shown in Fig. 5A. The relative expression levels of both pCREB and tCREB were calculated after normalization to the β-actin bands from four different samples of irradiated mouse testes. Testis values in the sham control group were arbitrarily defined as 1, and the basal activity of CREB was normalized to the intensity of the total CREB expression. pCREB levels transiently increased by 1.7-fold 12 hours post-irradiation. Although the administration of rolipram to non-irradiated mice did not significantly change the pCREB level, rolipram in addition to irradiation increased the pCREB level as compared to the irradiated group 12 hours post-irradiation. In contrast, pCREB levels substantially decreased (47%) in the testis at 35 days post-irradiation. Administration of rolipram to both non-irradiated and irradiated animals increased the level of pCREB at 35 days post-irradiation, but rolipram administration in addition to irradiation significantly increased the level of pCREB as compared to the vehicle-treated irradiation group (p=0.01). However, irradiation and rolipram treatment were not associated with changes in tCREB at either 12 hours or 35 days post-irradiation (Fig. 5A).
In agreement with these findings, the immunohistochemical analysis indicated that pCREB expression was intense in the nuclei of the spermatogonia, pachytene spermatocytes, round spermatids, and Sertoli cells in the testis in the sham control group. In contrast, the irradiated testis had decreased levels of pCREB in the seminiferous tubule 35 days after irradiation, which correlated with testicular injury. Rolipram administration suppressed this radiation-induced decrease in pCREB (Fig. 5B).
Testicular damage may occur during direct irradiation of the testis or, more commonly, from scattered radiation during treatment directed at adjacent tissues [1]. The testis receives a significant amount of scatter irradiation during pelvic and abdominal irradiation, which may be sufficient to cause infertility [2]. We evaluated the efficacy and mechanism of action of rolipram against irradiation-induced testicular injury by using a focal pelvic-irradiated mouse model. Our findings show that rolipram can ameliorate the adverse effects on spermatogenesis associated with radiation-induced testicular injury in mice. This radioprotective effect of rolipram appears to be mediated by the activation of CREB signaling.
Rolipram is known to enhance cAMP levels and stimulate the CREB pathway. cAMP/CREB signaling mediates the antiapoptotic effect of rolipram in various organs [7,14,16]. CREB appears to be a primary transcriptional activator of the antiapoptotic gene BCL-2 [19]. Inhibition of CREB activity induces apoptosis in neurons, whereas CREB overexpression inhibits apoptosis [14,18,20]. Moreover, kinase-mediated CREB phosphorylation promotes normal cell survival and proliferation [21,22]. The loss of male germ cells after radiation exposure has been attributed to apoptosis [23]. In the present study, we found that germ cell apoptosis was increased at 2 hours post-irradiation, with a maximum frequency at 12 hours post-irradiation. These findings are consistent with those of our previous study [24]. Rolipram remarkably inhibited germ cell apoptosis after irradiation. Irradiation transiently increased pCREB in the testis after 12 hours. Importantly, rolipram treatment further increased pCREB in irradiated mice. This suggests that the radioprotective effect of rolipram on male germ cell survival seems to involve CREB activation. Our findings are consistent with those of a previous study demonstrating that endogenous CREB activation triggers a potent survival signal in irradiated cells [25]. Although further investigation is needed to better understand the mechanisms underlying the antiapoptotic effect of rolipram in irradiated testes, it is possible that activation of the cAMP/CREB pathway, which protects testicular stem cell spermatogonia and differentiating cells from radiation injury, is at least partially responsible.
We found that radiation-induced germ cell apoptosis decreased the sperm count in the cauda epididymis 35 days after irradiation. Irradiation has been shown to adversely affect spermatogonia cell proliferation, leading to the death and depletion of spermatogonia cells and their subsequent generations and testis weight loss [26]. Because of the high radiosensitivity of type-B spermatogonia, low-dose radiation can decrease spermatogenesis [27]. Radiosensitivity depends on the proliferative capacity of the cell type. Type-B spermatogonia in the adult testis actively proliferate, rendering them highly radiosensitive. Ki-67 is a very reliable endogenous marker of cell proliferation because it is expressed during mitosis and has a short half-life [28,29]. Among the different types of spermatogonia generated during normal spermatogenesis, Ki-67 immunoreactivity was found to be significantly higher in type-B spermatogonia [30]. Furthermore, Ki-67 can be easily detected by immunohistochemistry. Thus, we selected Ki-67 to detect proliferating cells in the testis. In this study, rolipram treatment protected testicular stem cell spermatogonia and differentiating cells from radiation injury. There are many tubules containing three or more spermatogenic cells (proliferating cells) in the rolipram+ irradiation group compared with the vehicle+irradiation group. Components present in rolipram may have possibly stimulated the subsequent proliferation of these protected stem cells. Together, these effects of rolipram could account for the repopulation of the seminiferous tubules and subsequent restoration of the testis weight. Because of the repopulation of the semi-niferous tubules with various orders of germ cells, rolipram was able to restore the seminiferous tubular diameter in irradiated mice.
CREB is known to play many roles in the development and normal function of the testes. Previous studies have established the cAMP-dependent signal transduction pathway as a major regulatory mechanism that operates at different stages of spermatogenesis [6]. CREB may be a key molecular regulator of testicular development and adult spermatogenesis in mice [6,7]. CREB is expressed during the mitotic phase of spermatocytogenesis and the differentiation phase of spermiogenesis, suggesting that CREB plays an important role in these processes [7]. In the present study, we showed that irradiation decreased the epithelial cell layer including the spermatogonia and the relative pCREB expression level in the testis 35 days after 2-Gy irradiation. However, rolipram treatment attenuated this decrease in pCREB, which correlated with the decrease in testicular damage. Our findings suggest that the radioprotective effects of rolipram are associated with its CREB-mediated antiapoptotic effect. Future studies using CREB inhibitors and knockout mice are needed to unravel the exact mechanism underlying the radioprotective effects of rolipram in the testes.
We demonstrated that irradiation decreases the constitutive CREB activity in the seminiferous tubules of the testes and that promotion of CREB activity by rolipram attenuates the radiation-induced reduction in spermatogenesis. Thus, rolipram may act as a radioprotector, possibly by protecting germ cells from irradiation-mediated damage. These results provide evidence that rolipram may have useful clinical applications in the prevention of testicular injury following irradiation.
ACKNOWLEDGEMENTS
This work was supported by the Nuclear R&D Program of the Ministry of Education, Science and Technology, Korea (50609-2013).
References
1. Howell SJ, Shalet SM. Spermatogenesis after cancer treatment: damage and recovery. J Natl Cancer Inst Monogr. 2005; (34):12–17. PMID: 15784814.


2. Budgell GJ, Cowan RA, Hounsell AR. Prediction of scattered dose to the testes in abdominopelvic radiotherapy. Clin Oncol (R Coll Radiol). 2001; 13:120–125. PMID: 11373874.


3. Kovacs GT, Stern K. Reproductive aspects of cancer treatment: an update. Med J Aust. 1999; 170:495–497. PMID: 10376028.


4. Costabile RA. The effects of cancer and cancer therapy on male reproductive function. J Urol. 1993; 149:1327–1330. PMID: 8479029.


5. Montminy M. Transcriptional activation. Something new to hang your HAT on. Nature. 1997; 387:654–655. PMID: 9192883.
6. Don J, Stelzer G. The expanding family of CREB/CREM transcription factors that are involved with spermatogenesis. Mol Cell Endocrinol. 2002; 187:115–124. PMID: 11988318.


7. Kim JS, Song MS, Seo HS, Yang M, Kim SH, Kim JC, et al. Immunohistochemical analysis of cAMP response element-binding protein in mouse testis during postnatal development and spermatogenesis. Histochem Cell Biol. 2009; 131:501–507. PMID: 19148668.


8. Nagakura A, Niimura M, Takeo S. Effects of a phosphodiesterase IV inhibitor rolipram on microsphere embolism-induced defects in memory function and cerebral cyclic AMP signal transduction system in rats. Br J Pharmacol. 2002; 135:1783–1793. PMID: 11934820.


9. Vitolo OV, Sant'Angelo A, Costanzo V, Battaglia F, Arancio O, Shelanski M. Amyloid beta-peptide inhibition of the PKA/CREB pathway and long-term potentiation: reversibility by drugs that enhance cAMP signaling. Proc Natl Acad Sci U S A. 2002; 99:13217–13221. PMID: 12244210.
10. Kim JS, Yang M, Cho J, Kim SH, Kim JC, Shin T, et al. Promotion of cAMP responsive element-binding protein activity ameliorates radiation-induced suppression of hippocampal neurogenesis in adult mice. Toxicol Res. 2010; 26:177–183. PMID: 24278522.


11. Li YF, Huang Y, Amsdell SL, Xiao L, O'Donnell JM, Zhang HT. Antidepressant- and anxiolytic-like effects of the phosphodiesterase-4 inhibitor rolipram on behavior depend on cyclic AMP response element binding protein-mediated neurogenesis in the hippocampus. Neuropsychopharmacology. 2009; 34:2404–2419. PMID: 19516250.


12. Gong B, Vitolo OV, Trinchese F, Liu S, Shelanski M, Arancio O. Persistent improvement in synaptic and cognitive functions in an Alzheimer mouse model after rolipram treatment. J Clin Invest. 2004; 114:1624–1634. PMID: 15578094.


13. Monti B, Berteotti C, Contestabile A. Subchronic rolipram delivery activates hippocampal CREB and arc, enhances retention and slows down extinction of conditioned fear. Neuropsychopharmacology. 2006; 31:278–286. PMID: 15988467.


14. Wang C, Yang XM, Zhuo YY, Zhou H, Lin HB, Cheng YF, et al. The phosphodiesterase-4 inhibitor rolipram reverses A β-induced cognitive impairment and neuroinflammatory and apoptotic responses in rats. Int J Neuropsychopharmacol. 2012; 15:749–766. PMID: 21733236.
15. Mammadov E, Aridogan IA, Izol V, Acikalin A, Abat D, Tuli A, et al. Protective effects of phosphodiesterase-4-specific inhibitor rolipram on acute ischemia-reperfusion injury in rat kidney. Urology. 2012; 80:1390.e1. 1390.e6. PMID: 23010343.


16. Parkkonen J, Hasala H, Moilanen E, Giembycz MA, Kankaanranta H. Phosphodiesterase 4 inhibitors delay human eosinophil and neutrophil apoptosis in the absence and presence of salbutamol. Pulm Pharmacol Ther. 2008; 21:499–506. PMID: 18282775.


17. Kim J, Lee S, Jeon B, Jang W, Moon C, Kim S. Protection of spermatogenesis against gamma ray-induced damage by granulocyte colony-stimulating factor in mice. Andrologia. 2011; 43:87–93. PMID: 21382061.


18. Kim JS, Heo K, Yi JM, Gong EJ, Yang K, Moon C, et al. Genistein mitigates radiation-induced testicular injury. Phytother Res. 2012; 26:1119–1125. PMID: 22162311.


19. Pugazhenthi S, Miller E, Sable C, Young P, Heidenreich KA, Boxer LM, et al. Insulin-like growth factor-I induces bcl-2 promoter through the transcription factor cAMP-response element-binding protein. J Biol Chem. 1999; 274:27529–27535. PMID: 10488088.


20. Walton M, Woodgate AM, Muravlev A, Xu R, During MJ, Dragunow M. CREB phosphorylation promotes nerve cell survival. J Neurochem. 1999; 73:1836–1842. PMID: 10537041.
21. Wen AY, Sakamoto KM, Miller LS. The role of the transcription factor CREB in immune function. J Immunol. 2010; 185:6413–6419. PMID: 21084670.


22. François F, Godinho MJ, Grimes ML. CREB is cleaved by caspases during neural cell apoptosis. FEBS Lett. 2000; 486:281–284. PMID: 11119719.


23. Liu G, Gong P, Zhao H, Wang Z, Gong S, Cai L. Effect of low-level radiation on the death of male germ cells. Radiat Res. 2006; 165:379–389. PMID: 16579650.


24. Lee HJ, Kim JS, Moon C, Kim JC, Jo SK, Kim SH. Relative biological effectiveness of fast neutrons in a multiorgan assay for apoptosis in mouse. Environ Toxicol. 2008; 23:233–239. PMID: 18214905.


25. Cataldi A, di Giacomo V, Rapino M, Genovesi D, Rana RA. Cyclic nucleotide Response Element Binding protein (CREB) activation promotes survival signal in human K562 erythroleukemia cells exposed to ionising radiation/etoposide combined treatment. J Radiat Res. 2006; 47:113–120. PMID: 16819137.


26. Zhang H, Zheng RL, Wei ZQ, Li WJ, Gao QX, Chen WQ, et al. Effects of pre-exposure of mouse testis with low-dose (16)O8+ ions or 60Co gamma-rays on sperm shape abnormalities, lipid peroxidation and superoxide dismutase (SOD) activity induced by subsequent high-dose irradiation. Int J Radiat Biol. 1998; 73:163–167. PMID: 9489563.
27. Kagan AR. Bladder, testicle, and prostate irradiation injury. Front Radiat Ther Oncol. 1989; 23:323–337. discussion 338-40. PMID: 2697661.


28. Scholzen T, Gerdes J. The Ki-67 protein: from the known and the unknown. J Cell Physiol. 2000; 182:311–322. PMID: 10653597.


29. Kim JS, Jung J, Lee HJ, Kim JC, Wang H, Kim SH, et al. Differences in immunoreactivities of Ki-67 and doublecortin in the adult hippocampus in three strains of mice. Acta Histochem. 2009; 111:150–156. PMID: 18649926.


30. Steger K, Aleithe I, Behre H, Bergmann M. The proliferation of spermatogonia in normal and pathological human seminiferous epithelium: an immunohistochemical study using monoclonal antibodies against Ki-67 protein and proliferating cell nuclear antigen. Mol Hum Reprod. 1998; 4:227–233. PMID: 9570268.


Fig. 1
Protective effect of rolipram from apoptotic cell death in seminiferous tubules following pelvic irrdiation (IR) (2Gy). (A) Representative images (×400) showing apoptotic cells stained with transferase dUTP-biotin nick end labeling (TUNEL) in irradiation testis with or without rolipram treatment at 12 hours post-irradiation. Apotosis was easily recognized by the presence of entire apoptotic bodiesstained by peroxidase. Irradiation increased the expression of the apoptotic nuclei in the germ cells of the mice. Most of the apoptotic cells were in the spermatogonia and primary spermatocytes. Rolipram treatment before irradiation decreased the TUNEL-positive cells compared with the vehicle-treated mice. (B) The graph depicts the number of apoptotic cells per seminiferous tubule in the testis section, as observed using the TUNEL method. Values are reported as the mean±standard deviation of five mice in each group (*p<0.05 as compared to the irradiation control group).
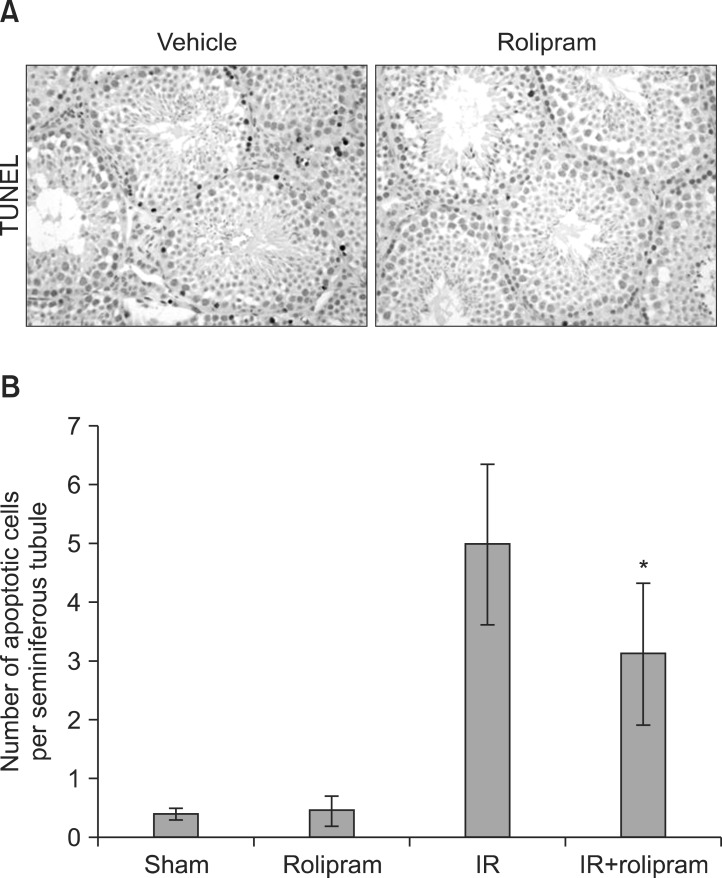
Fig. 2
Protective effect of rolipram on the body and testis weight 35 days following pelvic irrdiation (IR) (2Gy). Graphs showing body weight (A), testis weight (B), and testis per body weight (C). Values are reported as the mean±standard deviation of five mice in each group (*p<0.05 as compared with the irradiation control group).
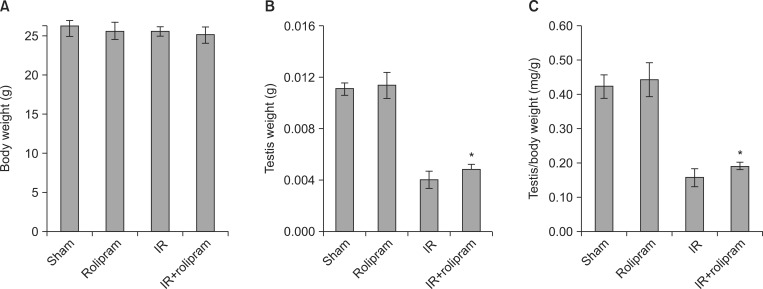
Fig. 3
Protective effect of rolipram on radiation-induced histopathological changes in the testis 35 days following pelvic irrdiation (IR) (2Gy). (A) Representative images (×400) showing H&E and Ki-67-stained testis sections from the irradiation control and rolipram+irradiation groups. Graphs showing the histological features of epithelial height (B) and seminiferous diameter (C). Ki-67 positive cells were detected primarily in the lower layer of the seminiferous tubule, and the immunopositive cells correlated with the tubule germinal cells. (D) Repopulation index (RI) was calculated to show the survival of spermatogonial stem cells. (E) The stem cell survival index (SSI) was calculated using the following equation: SSI=-[lne (1-RI÷100)]. Irradiation reduced SSI and increased RI, but rolipram attenuated these changes. Values are reported as the mean±standard deviation of five mice in each group (*p<0.05 as compared with the irradiation control group).
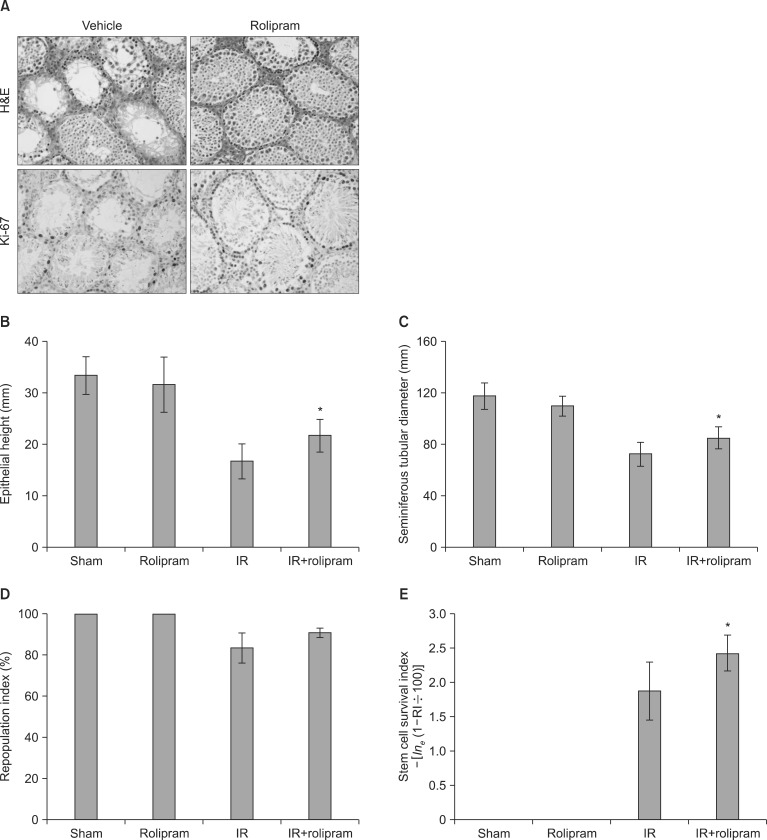
Fig. 4
Protective effect of rolipram on sperm characteristics in the testis 35 days following pelvic irrdiation (IR) (2Gy). Graphs showing the number of sperm per cauda epididymis (A), and sperm mobility (B). Values are reported as the mean±standard deviation of five mice in each group (*p<0.05 as compared with the irradiation control group).
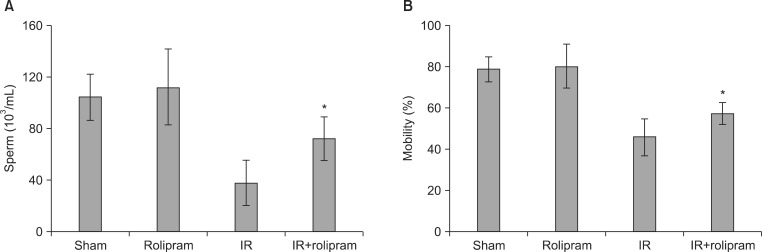
Fig. 5
Effect of rolipram on phosphorylated CREB (pCREB) and total (tCREB) expression in the testis after pelvic irradiation (IR) (2 Gy). (A) Western blot analysis of pCREB and tCREB expression after 12 hours and 35 days following pelvic irrdiation (2 Gy). Values are reported as the mean±standard deviation of five mice in each group (*p<0.05 as compared with the irradiation control group). (B) Representative images (×100) showing pCREB-stained testis sections from the sham control, rolipram+sham control, irradiation control, and rolipram+irradiation groups 35 days after irradiation. OR: odds ratio.
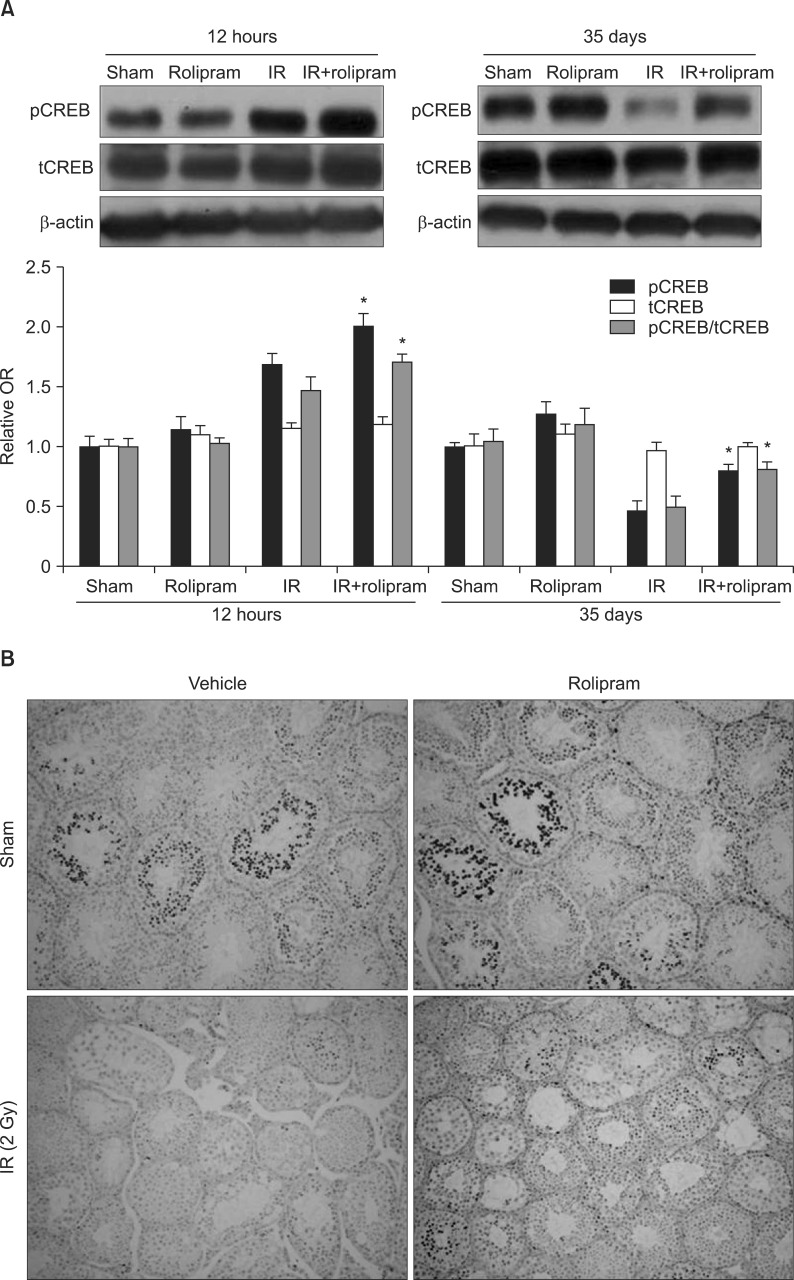