Abstract
The work-up of a febrile urinary tract infection is generally performed to detect vesicoureteral reflux (VUR) and its possible complications. The imaging modalities most commonly used for this purpose are renal-bladder ultrasound, voiding cystourethrogram and dimercapto-succinic acid scan. These studies each contribute valuable information, but carry individual benefits and limitations that may impact their efficacy. Biochemical markers are not commonly used in pediatric urology to diagnose or differentiate high-risk disease, but this is the emerging frontier, which will hopefully change our approach to VUR in the future. As it becomes more apparent that there is tremendous clinical variation within grades of VUR, the need to distinguish clinically significant from insignificant disease grows. The unfortunate truth about VUR is that recommendations for treatment may be inconsistent. Nuances in clinical decision-making will always exist, but opinions for medical versus surgical intervention should be more standardized, based on risk of injury to the kidney.
The purpose of this review is to assess the current literature on imaging modalities and biochemical markers as it pertains to the detection of clinically meaningful vesicoureteral reflux (VUR). Thus, we will be looking at studies to ascertain which patients with reflux may be susceptible to renal damage or recurrent febrile urinary tract infection (FUTI). This is the population that may develop complications of VUR, such as hypertension, proteinuria and reduced renal function. Historically, the presence of reflux was used as a proxy for renal parenchymal injury because it was assumed that renal scarring would only occur in response to VUR. That relationship is now being challenged. Scars may develop in the absence of reflux, and a reflux associated urinary tract infection (UTI) does not always lead to scarring. How do we distinguish between VUR, which may never have associated sequelae, from that which leads to complications? What radiographic tools do we have available to us now, and what can we expect to include in our armamentarium from the new field of biochemical markers?
UTIs in young children are common with an overall prevalence of 7.0% among infants presenting with fever and a pooled prevalence of 7.8% among children with urinary symptoms [1]. This finding usually prompts a radiographic investigation in an effort to identify which children are at risk for recurrent infections and renal damage. In theory, this allows a therapy to be instituted to prevent future illness or injury. The 10% to 20% of patients with repeat UTIs and subsequent scarring develop hypertension [2]. Furthermore, in severe cases of reflux nephropathy, 10%–25% of patients may develop end-stage renal disease such that they require dialysis or kidney transplant [2345]. The controversy surrounds the starting point of the work-up: should we focus on the condition of the kidneys (“top-down”) or the presence of VUR (“bottom-up”).
Historically, the presence of VUR was used to guide major management decisions. The “bottom-up” approach relies on a voiding cystourethrogram (VCUG) to determine if VUR or other lower tract anomalies exist, along with a renal-bladder ultrasound (RBUS) to detect renal parenchymal defects or dilation suggestive of obstruction. Alternatively, the “top-down” approach draws the focus to renal parenchymal injury from the beginning with a dimercapto-succinic acid (DMSA) scan (Fig. 1). An abnormal DMSA scan shows cortical defects or areas of decreased tracer uptake suggestive of tissue inflammation. These patients are recommended to undergo VCUG to diagnose VUR. Delayed DMSA scan (>6–12 months after the FUTI) may delineate permanent damage [6]. The sequence of events varies within each approach, but a normal test precludes further studies. For the bottom-up approach, fewer DMSA scans will be performed. The same will be true for VCUG studies in the top-down approach. Why do 2 distinct methodologies exist? Catheterization during a VCUG can be traumatic for the patient and family [78]. Also, the test does not differentiate which patients will experience repercussions from their VUR. Some encourage starting with a DMSA scan to determine which patients are most vulnerable to progressive injuries, and thus avoid catheterization in the process. There are strengths and limitations associated with each algorithm.
The modern day approach to FUTI was originally codified by the 1999 American Academy of Pediatrics (AAP) practice guidelines, which recommended the regimen we now recognize as the “bottom-up” method, based on the association between UTIs and urinary tract abnormalities [9]. Thus, a 2- to 24-month old child with a FUTI was advised to undergo RBUS followed by VCUG. At the time, renal cortical scintigraphy with a DMSA study was mentioned as an option, but its role was considered “unclear” [9].
The relationship between VUR and renal scarring is clearly not 1:1; however some association undeniably exists and this formed the basis for the VCUG recommendation: to identify those individuals with VUR that may be at subsequent risk for secondary scarring. However, in subsequent research, it was found that only 30%–40% of children with a UTI will have VUR, which suggests that over 60% of VCUG tests ordered for this indication may be unnecessary [1011]. Furthermore, the ability of antimicrobial prophylaxis to prevent recurrent FUTI was challenged by several controversial studies [1213141516]. Thus, in 2011, the AAP revised their guidelines to limit the initial work-up to RBUS alone. VCUG was recommended only for specific abnormalities identified on RBUS, recurrent FUTI, or in “other atypical or complex clinical circumstances” (although the latter was not specified) since the utility of prophylaxis as a VUR management strategy was in doubt [17]. Once again, the role of DMSA was not mentioned. In 2014, the first multi-institutional, randomized, double-blind, placebo-controlled trial of prophylactic antibiotics (the RIVUR study) demonstrated a clear reduction in recurrence of FUTI in children with VUR [18]. This reinforced the findings of similar trials out of Australia and Sweden, which support the use of prophylaxis [1619]. Accordingly, the foundation of the 2011 AAP guideline recommendation is ambiguous as it is based on an incorrect premise.
Similarly, the National Institute for Health and Care Excellence (NICE) in the United Kingdom published recommendations in 2007, which called for limiting the use of VCUG and DMSA [20]. This stood in contrast to the original advice given in a 1991 publication of the Royal College of Physicians, London, which suggested the original “top-down” approach. In this methodology, an RBUS and DMSA study was deemed appropriate for all children with a diagnosed FUTI, but limited VCUG to those with FUTI under 12 months of age, abnormal initial studies (RBUS, DMSA) or recurrent FUTI [21]. This tactic determines whether the kidney is involved during a FUTI. Patients with cortical defects or regions of poor tracer uptake are recommended to undergo a VCUG to assess for VUR, in addition to a late DMSA to delineate persistent injury or damage [6].
Finally, the American Urological Association released a guideline in 2010 on the management and screening of primary VUR. This guideline emphasized the use of RBUS and VCUG every 12–24 months in the follow-up of VUR, as a recommendation. DMSA scans were recommended only when the RBUS was abnormal or if there was a greater concern for scarring or elevated creatinine [10]. DMSA was considered an option to detect new renal scarring, especially after a FUTI, but was not deemed an essential part of the work-up for straightforward cases.
In the wake of each guideline, studies were performed assessing how retrospective populations may have been impacted by the recommendations, emphasizing the significant populations that may have been missed by limitations on exams [222324]. Due to the considerable conflicting information, many physicians remain skeptical of the guidelines and are unsure of how or when to implement the various radiographic studies. Furthermore, although the guidelines specified a particular age range, it was widely recognized that the recommendations were commonly extrapolated to other populations in the clinical setting and therefore had wider implications. Thus, we remain in search of secondary clinical or biochemical markers to assess and predict the severity of renal involvement. This will hopefully bring some clarity to the persistent controversy of VUR.
Three studies are commonly employed in the work-up of FUTI: RBUS, VCUG, and DMSA. Newer techniques and technologies using magnetic resonance urography (MRU) and contrast-enhanced voiding urosonography (CEVUS) are also emerging. These innovative studies may impact management strategies in the future, but they are not routinely used to examine patients with FUTI or differentiate between patients with clinically significant VUR.
RBUS is the most common initial intervention for a child with febrile or afebrile UTI. It does not use ionizing radiation and it is generally well tolerated since it is painless and noninvasive. It can quickly and broadly show the urinary tract anatomy. However, many children with congenital urinary tract abnormalities are now identified with prenatal ultrasounds and undergo medical or surgical interventions before a FUTI may occur [825]. RBUS is often seen as a fundamental part of urologic consultation or surveillance; but for infants with rigorous prenatal care, it may offer only a negligible benefit.
It has been repeatedly shown that RBUS results do not significantly alter the treatment plan for a patient in the same manner as a abnormal DMSA scan or VCUG [25]. RBUS only retains a sensitivity and specificity of 40% and 76%, respectively for VUR [26]. Although it is widely available, the quality of the study is technician dependent, it does not provide a quantitative assessment of renal function and it is not sensitive enough to detect all scarring. On the other hand, it is often comforting to the family to have a safe, noninvasive exam that grossly reveals the condition of the kidneys.
In the era of reduced utilization of ionizing radiation, and multiple guidelines emphasizing a reliance on RBUS over other studies, many investigations have been published assessing the accuracy of RBUS as a screening test for genitourinary anomalies (including VUR). The largest of these studies examined a retrospective cohort of 2,259 patients <60 months of age who underwent both VCUG and RBUS for a history of FUTI. In this population, participants received both studies on the same day. Obtaining one study was not contingent upon the results of the other. Regardless of criteria used for positive or negative tests, RBUS was found to have poor sensitivity and had it had low positive predictive value. Negative predictive values were only notable for the highest grades of VUR. Specificity was high (up to 97%) but only when sensitivity was low (<10%). Thus, a “normal” RBUS does not rule out significant grades of VUR. Similarly, a “positive” test is not predictive of pathology [24]. Thus, the authors conclude that both RBUS and VCUG studies are needed for complete anatomic information about a patient.
This same population was assessed to determine if sophisticated predictive models could be constructed using a combination of “positive” RBUS findings, such as renal dilation, urothelial thickening, ureteral dilation, parenchymal abnormalities, and bladder abnormalities. Despite employing multivariate logistic models and neural network machine learning algorithms, these various findings could not be combined into a predictive model that would accurately screen for abnormalities detected by VCUG. They found that RBUS had no predictive value for even high-grade VUR and thus was a poor screening test for this purpose [27]. Once again, the authors concluded that the RBUS study was complementary to a VCUG, rather than providing adequate information in isolation.
VCUG is the gold-standard exam to assess for VUR. This test will readily identify reflux, but it is difficult to predict which subset of patients will have harmful sequelae of their disease, such as renal scarring. Thus, a proportion of patients with VUR will undergo the morbidity of surveillance and potentially be subjected to over-treatment if they rely on VCUG alone.
In recent years, researchers have found there are various nuances to the VCUG test that can be exploited to give us more information than simply the presence or absence of VUR and a reflux grade. Features of the VCUG that hold predictive value for spontaneous resolution of VUR, as well as for risk of FUTI recurrence, include a calculated ureteral diameter ratio and the bladder volume (as a percentage of the predicted capacity) at the onset of reflux. These parameters proved more predictive than reflux grade alone [282930], which most studies have used exclusively in the past. In fact, a computational model has been created using these features, along with some clinical variables, which has >75% accuracy at predicting the odds of a breakthrough FUTI. This will likely be available for public use on the internet in the near future [31].
A crucial fact to bear in mind is that VUR does not necessarily produce renal parenchymal damage after a FUTI [23233]. Similarly, one-third of infants and 37% of children aged 1–5 years may exhibit renal injury in the absence of VUR [3334]. VCUG cannot completely define the at-risk population. VUR may spontaneously resolve and not all VUR + FUTI = scar. Therefore, this test will overlook the cohort of children that experience renal scarring without VUR.
DMSA is now the gold-standard to assess for kidney injury and persistent renal scars, replacing the intravenous urogram. The fundamental idea of the “top-down” approach is to detect which kidneys are vulnerable to subsequent injury with an early DMSA scan. A normal DMSA scan will preclude the need to order a VCUG. This will isolate the cohort with VUR that is most at-risk and reduce the need for widespread VCUG testing. This perspective places the emphasis on renal parenchymal involvement, rather than VUR, as the stimulus for accumulated damage [323536]. Subsequent DMSA scans demonstrate new scars in the sites of previous inflammation.
A 2016 meta-analysis of published studies was performed by the Cochrane Database of Systematic Reviews to determine whether RBUS or DMSA had greater accuracy in diagnosing any grade VUR or high-grade VUR (grade III-V VUR), such that they could be used as a screening tool. The authors only reviewed studies in which children underwent both the index test (RBUS or DMSA) and a VCUG in the context of a culture-proven FUTI. Summary sensitivity and specificity estimates were determined for the target conditions. There was substantial heterogeneity between the studies and none of them reported high values individually. The authors concluded that neither RBUS nor DMSA has been shown to have sufficient accuracy to detect any grade VUR or high-grade VUR. Although a negative DMSA study had a summary sensitivity of 0.93, the specificity was low (0.44), such that it limits its utility as a screening test for VUR [37].
When considering the evidence, one should remember that there is a lack of consensus about the etiology and significance of scars seen on initial DMSA or RBUS. Congenital dysplasia may account for cortical defects rather than new injuries from infection and/or VUR. Newborns with prenatally-diagnosed hydronephrosis and no personal history of UTI can have an abnormal DMSA study [3538]. As with VCUGs, an abnormal DMSA scan may erroneously isolate a cohort of children as being vulnerable to acquired renal scarring when, in fact, their defects are representative of static congenital dysplasia. Still, persistent renal scarring is identified in at least 50% of patients with evidence of inflammation on DMSA at the time of a FUTI [3639]. In either scenario, a subset of children will potentially be subjected to over-treatment. However, the morbidity of surveillance in a child with clinically insignificant VUR is arguably greater than the conservative management of a child with a congenitally dysplastic kidney.
Both VCUG and DMSA scans expose children to potentially detrimental ionizing radiation, which is unappealing for families. In the past, VCUGs were found to convey a larger dose of radiation to the gonads [40]. However, DMSA carries a 10 fold higher radiation dose than pulsed fluoroscopy, which is currently used in most centers for VCUG exams [41]. Still, radiation during a DMSA scan is dispersed throughout the body with concentration in the kidneys while the energy during fluoroscopy is focused on the pelvis. Serial exams would increase this exposure. Other drawbacks to the DMSA scan include the need for intravenous access and possibly sedation, lack of availability at all centers, inconsistent and delayed interpretation of the exam and the longer duration of the test (1–3 hours). A major limitation of VCUG is the requirement for urethral catheterization, which can be a source of distress for the patient and family. Thus, the search continues for alternative technology that can overcome the risks and flaws outlined above.
As the diagnostic paradigm for FUTI is evolving, so is the technology available to practitioners. The debate may be altered significantly by the radiographic options on the horizon as they change diagnostic capabilities and the understanding of disease processes.
MRU can provide both anatomic and functional data in one study. Due to the improved spatial and contrast resolution, congenital renal dysplasia can be differentiated from acquired renal damage on MRU [42]. Reports have shown an association between VUR grade and the degree and volume of renal parenchymal damage detected by MRU [43]. In a retrospective review of 114 patients with VUR and 21 nonrefluxing controls, MRU was able to detect a renal size discrepancy between the 2 groups. This size discrepancy persisted in the comparison of contralateral nonrefluxing kidneys with nonrefluxing controls and occurred in the absence of focal scarring (p<0.0001). This data supports the notion that patients with VUR can have abnormal embryological development or hypoplasia before birth and the first insult of a FUTI. Alternatively, the contralateral nonrefluxing kidney may be impacted by bilateral pyelonephritis initiated by the refluxing kidney. The etiology is still unclear, but the association between VUR and FUTI could be characterized more completely if MRU assessment is included in future studies.
It would be ideal to perform a detailed evaluation of renal morphologic abnormalities and test for VUR at the same time without the hazards of irradiation. Magnetic resonance imaging (MRI) has the potential to do both, as demonstrated by several recent studies. One of the earliest studies of magnetic resonance voiding cystography (MRVC) using intraurethral gadolinium found excellent concordance between this technique and the detection of VUR with VCUG and renal damage with DMSA [44], with the added benefit of detecting additional urinary tract anomalies.
This method was refined in a report on interactive MRVC (iMRVC), which involves using a pulse sequence and rapid switching between views to permit prolonged dynamic imaging of the urinary tract [45]. A feasibility study in unsedated infants was performed on 12 patients with a first FUTI or abnormalities on early postnatal ultrasound [45]. VUR was identified in 5 children using iMRVC versus 3 using conventional VCUG (sensitivity 100%, specificity 83% for iMRVC). One limitation worth mentioning, however, is that the iMRVC studies followed a single cycle VCUG study. VUR detection is known to increase with successive rounds of bladder filling. The iMRVC studies obtained adequate images of the urethra and the technique was refined over the course of the trial such that the imaging time dropped from 60 minutes to 20 minutes, commensurate with the time required for a VCUG [45].
The newest technique reported involves intravoxel incoherent motion (IVIM) diffusion-weighted imaging in MRI. Only one report has been published so far on 83 kidneys from 57 patients. A specific index measure of IVIM perfusion and diffusion parameters was found to have a reported accuracy of 78% (sensitivity 0.81 and specificity 0.77) [46]. This is a small but promising study that requires validation and testing in a larger population. This method is considered noninvasive as it does not require contrast media or catheterization, and like other MR techniques, is free of ionizing radiation.
Although these testing modalities offer elegant structural features in conjunction with dynamic, functional information, they are costly, require sophisticated processing techniques, and may necessitate sedation in younger patient populations. Contrast agents in MRU studies are associated with an unusual but disabling complication known as nephrogenic systemic fibrosis, and the hazards may be enhanced for patients with chronic kidney disease [42]. Perhaps these emerging technologies can help to uncover the connection between VUR, FUTI, and renal parenchymal injury in a research setting. Unfortunately, they do not yet elucidate the best algorithm or methodology to study a patient with a FUTI. In terms of clinical practice, the demand for new imaging modalities may overtake the research to support it, as the present era is distinguished by the rapid adoption of new technology.
Returning to our starting point, ultrasound may have a renaissance at certain centers. In the past 2 decades, harmonic imaging and second-generation contrast agents have refined contrast CEVUS to improve accuracy. A published comparison of CEVUS with VCUG assessed children with FUTI, upper tract dilation or previously diagnosed VUR [47]. New or persistent VUR was diagnosed in the presence of contrast material in the ureter or pelvicalyceal system during the exam, but only a fraction of these were detected by VCUG; most were identified with CEVUS. This may be the result of continuous imaging using sonography versus intermittent fluoroscopy. The greatest drawback of this modality is inadequate imaging or visualization of the urethra. However, recent reports have demonstrated new techniques that have allowed the identification of posterior urethral valves, diverticula of the prostatic utricle and the anterior urethra in boys [48]. The bladder neck and urethra were adequately visualized in all of the examinations. This is consistent with prior reports using these same techniques [4950]. Similar to MRVC, the CEVUS study still requires urethral catheterization. In contrast to MRVC and MRU, CEVUS is less expensive and may be more accessible to a wider population. However, the techniques are still operator-dependent and require highly skilled sonographers.
Biological substances that can be accurately (and reproducibly) measured as an indicator of normal or pathogenic states or conditions are termed “biochemical markers.” They should be objective and quantifiable characteristics of a biological process. In order to be used as a surrogate endpoint for a clinical outcome, biochemical markers must undergo rigorous scientific validation to ensure that all possible variations of disease states are represented. It is not enough to seek statistical significance or prove the negative and/or positive predictive value of such a test [5152]. It is rare to find a biomarker that can reliably diagnose disease in isolation, but when multiplexed with other tests, their value may be enhanced. More broadly, certain biochemical markers can be employed to monitor disease burden or progression and to predict response to a therapeutic intervention, as we do with particular cancers [5152]. In the setting of VUR, it would be ideal to identify reliable noninvasive biochemical markers that could differentiate between low versus high-risk populations to supplement clinical decision-making.
Biochemical markers have been explored in nearly every aspect of VUR, from trying to evaluate susceptibility to UTI, to associations between VUR and renal scarring, to markers of acute kidney injury [52]. Classically, metabolic parameters such as serum creatinine, creatinine clearance, cystatin C and radiologic findings were used to try to identify patients with renal damage, but these values did not adequately detect a loss of function before the injury was significantly advanced. Urinary substances are of particular interest as the collection method is noninvasive. However, an entire review article could be devoted to urinary biochemical markers that have not reliably demonstrated clinical validity or studies that provide conflicting evidence [53545556]. Serum procalcitonin is an excellent example of this phenomenon [57].
Recent publications have investigated a variety of noninvasive urinary biochemical markers for reflux nephropathy in children with primary VUR [5356]. The systemic host reaction to acute pyelonephritis supports the release of molecules and cytokines into the serum and urinary tract that can initiate or modulate the inflammatory response. Genetic polymorphisms have been identified in children who appear to be prone to pyelonephritis, even in the absence of VUR [58]. It has been suggested that if one marker cannot be identified in exclusion, perhaps panels or combinations of biochemical markers will augment performance in a clinical setting [52], but those analyses have not matured to the point of clinical utility. Small study sample sizes continue to limit applicability to a clinical setting so far. It is currently impossible to provide definitive reproducible evidence for any of these factors. Despite 20 years of investigation, the field remains in its infancy.
The tapered work-up now being advocated by multiple guideline entities represents a striking departure from earlier paradigms [172059]. A recent meta-analysis revealed that the rates of children with reflux nephropathy progressing to end-stage renal disease remain similar despite our current intervention strategies [60]. That statistic will not improve with the emerging trend to reduce the evaluation of patients with FUTI. Undoubtedly, it would be ideal to concentrate on patients most vulnerable to renal damage, but that cohort has not been satisfactorily defined at this time. Although the diagnosis of VUR or scarring carries an unknown long-term predictive value, it heightens awareness among practitioners and the family such that the child is properly monitored and treated for further febrile events. Currently, the only noncontroversial statement one can make is that more evidence-based medicine is needed to elucidate which groups have clinically meaningful VUR and find ways to prevent the associated potential complications.
Figures and Tables
Fig. 1
Graphical representation of the top-down versus bottom-up approach to the work-up of a febrile urinary tract infection (FUTI). DMSA, dimercapto-succinic acid scan; VCUG, voiding cystourethrogram; RBUS, renal-bladder ultrasound; VUR, vesicoureteral reflux. The “+” symbol indicates that the study had a significant finding. The “−” symbol indicates that the study was normal.
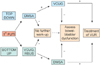
References
1. Shaikh N, Morone NE, Bost JE, Farrell MH. Prevalence of urinary tract infection in childhood: a meta-analysis. Pediatr Infect Dis J. 2008; 27:302–308.
2. Herz D, Merguerian P, McQuiston L, Danielson C, Gheen M, Brenfleck L. 5-year prospective results of dimercapto-succinic acid imaging in children with febrile urinary tract infection: proof that the top-down approach works. J Urol. 2010; 184:4 Suppl. 1703–1709.
3. Smellie JM, Barratt TM, Chantler C, Gordon I, Prescod NP, Ransley PG, et al. Medical versus surgical treatment in children with severe bilateral vesicoureteric reflux and bilateral nephropathy: a randomised trial. Lancet. 2001; 357:1329–1333.
4. Coulthard MG, Verber I, Jani JC, Lawson GR, Stuart CA, Sharma V, et al. Can prompt treatment of childhood UTI prevent kidney scarring? Pediatr Nephrol. 2009; 24:2059–2063.
5. Coulthard MG. Vesicoureteric reflux is not a benign condition. Pediatr Nephrol. 2009; 24:227–232.
6. Riccabona M, Avni FE, Blickman JG, Dacher JN, Darge K, Lobo ML, et al. Imaging recommendations in paediatric uroradiology: minutes of the ESPR workgroup session on urinary tract infection, fetal hydronephrosis, urinary tract ultrasonography and voiding cystourethrography, Barcelona, Spain, June 2007. Pediatr Radiol. 2008; 38:138–145.
7. Völkl-Kernstock S, Felber M, Schabmann A, Inschlag N, Karesch L, Ponocny-Seliger E, et al. Comparing stress levels in children aged 2-8 years and in their accompanying parents during first-time versus repeated voiding cystourethrograms. Wien Klin Wochenschr. 2008; 120:414–421.
8. Lee HY, Soh BH, Hong CH, Kim MJ, Han SW. The efficacy of ultrasound and dimercaptosuccinic acid scan in predicting vesicoureteral reflux in children below the age of 2 years with their first febrile urinary tract infection. Pediatr Nephrol. 2009; 24:2009–2013.
9. American Academy of Pediatrics. Committee on Quality Improvement. Subcommittee on Urinary Tract Infection. Practice parameter: the diagnosis, treatment, and evaluation of the initial urinary tract infection in febrile infants and young children. Pediatrics. 1999; 103(4 Pt 1):843–852.
10. Peters CA, Skoog SJ, Arant BS Jr, Copp HL, Elder JS, Hudson RG, et al. Summary of the AUA guideline on management of primary vesicoureteral reflux in children. J Urol. 2010; 184:1134–1144.
11. Peters CA. Vesicoureteral reflux: seeing the trees in the forest. J Urol. 2010; 184:8–9.
12. Pennesi M, Travan L, Peratoner L, Bordugo A, Cattaneo A, Ronfani L, et al. Is antibiotic prophylaxis in children with vesicoureteral reflux effective in preventing pyelonephritis and renal scars? A randomized, controlled trial. Pediatrics. 2008; 121:e1489–e1494.
13. Garin EH, Olavarria F, Garcia Nieto V, Valenciano B, Campos A, Young L. Clinical significance of primary vesicoureteral reflux and urinary antibiotic prophylaxis after acute pyelonephritis: a multicenter, randomized, controlled study. Pediatrics. 2006; 117:626–632.
14. Montini G, Rigon L, Zucchetta P, Fregonese F, Toffolo A, Gobber D, et al. Prophylaxis after first febrile urinary tract infection in children? A multicenter, randomized, controlled, noninferiority trial. Pediatrics. 2008; 122:1064–1071.
15. Roussey-Kesler G, Gadjos V, Idres N, Horen B, Ichay L, Leclair MD, et al. Antibiotic prophylaxis for the prevention of recurrent urinary tract infection in children with low grade vesicoureteral reflux: results from a prospective randomized study. J Urol. 2008; 179:674–679.
16. Craig JC, Simpson JM, Williams GJ, Lowe A, Reynolds GJ, Mc-Taggart SJ, et al. Antibiotic prophylaxis and recurrent urinary tract infection in children. N Engl J Med. 2009; 361:1748–1759.
17. Subcommittee on Urinary Tract Infection. Steering Committee on Quality Improvement and Management. Roberts KB. Urinary tract infection: clinical practice guideline for the diagnosis and management of the initial UTI in febrile infants and children 2 to 24 months. Pediatrics. 2011; 128:595–610.
18. RIVUR Trial Investigators. Hoberman A, Greenfield SP, Mattoo TK, Keren R, Mathews R, et al. Antimicrobial prophylaxis for children with vesicoureteral reflux. N Engl J Med. 2014; 370:2367–2376.
19. Brandström P, Esbjörner E, Herthelius M, Swerkersson S, Jodal U, Hansson S. The Swedish reflux trial in children: III. Urinary tract infection pattern. J Urol. 2010; 184:286–291.
20. National Institute for Health and Clinical Excellence (NICE). Urinary tract infection in under 16s: diagnosis and management. Clinical guideline 54. August 2007 [Internet]. London: National Institute for Health and Clinical Excellence;c2017. cited 2016 Dec 10. Available from: https://www.nice.org.uk/guidance/cg54.
21. Guidelines for the management of acute urinary tract infection in childhood. Report of a Working Group of the Research Unit, Royal College of Physicians. J R Coll Physicians Lond. 1991; 25:36–42.
22. Bush NC, Keays M, Adams C, Mizener K, Pritzker K, Smith W, et al. Renal damage detected by DMSA, despite normal renal ultrasound, in children with febrile UTI. J Pediatr Urol. 2015; 11:126.e1–126.e7.
23. Narchi H, Marah M, Khan AA, Al-Amri A, Al-Shibli A. Renal tract abnormalities missed in a historical cohort of young children with UTI if the NICE and AAP imaging guidelines were applied. J Pediatr Urol. 2015; 11:252.e1–252.e7.
24. Logvinenko T, Chow JS, Nelson CP. Predictive value of specific ultrasound findings when used as a screening test for abnormalities on VCUG. J Pediatr Urol. 2015; 11:176.e1–176.e7.
25. Hoberman A, Charron M, Hickey RW, Baskin M, Kearney DH, Wald ER. Imaging studies after a first febrile urinary tract infection in young children. N Engl J Med. 2003; 348:195–202.
26. Mahant S, Friedman J, MacArthur C. Renal ultrasound findings and vesicoureteral reflux in children hospitalised with urinary tract infection. Arch Dis Child. 2002; 86:419–420.
27. Nelson CP, Johnson EK, Logvinenko T, Chow JS. Ultrasound as a screening test for genitourinary anomalies in children with UTI. Pediatrics. 2014; 133:e394–e403.
28. Cooper CS, Alexander SE, Kieran K, Storm DW. Utility of the distal ureteral diameter on VCUG for grading VUR. J Pediatr Urol. 2015; 11:183.e1–183.e6.
29. Alexander SE, Arlen AM, Storm DW, Kieran K, Cooper CS. Bladder volume at onset of vesicoureteral reflux is an independent risk factor for breakthrough febrile urinary tract infection. J Urol. 2015; 193:1342–1346.
30. Knudson MJ, Austin JC, McMillan ZM, Hawtrey CE, Cooper CS. Predictive factors of early spontaneous resolution in children with primary vesicoureteral reflux. J Urol. 2007; 178(4 Pt 2):1684–1688.
31. Arlen AM, Alexander SE, Wald M, Cooper CS. Computer model predicting breakthrough febrile urinary tract infection in children with primary vesicoureteral reflux. J Pediatr Urol. 2016; 12:288.e1–288.e5.
32. Herz DB. The top-down approach: an expanded methodology. J Urol. 2010; 183:856–857.
33. Wu HY, Shortliffe LD. Top-down approach for evaluation of urinary tract infection. Urology. 2010; 75:514–515.
34. Gleeson FV, Gordon I. Imaging in urinary tract infection. Arch Dis Child. 1991; 66:1282–1283.
35. Pohl HG, Belman AB. The "top-down" approach to the evaluation of children with febrile urinary tract infection. Adv Urol. 2009; 783409.
36. Polito C, La Manna A, Rambaldi PF, Valentini N, Marte A, Lama G. Long-term evolution of renal damage associated with unilateral vesicoureteral reflux. J Urol. 2007; 178(3 Pt 1):1043–1047.
37. Shaikh N, Spingarn RB, Hum SW. Dimercaptosuccinic acid scan or ultrasound in screening for vesicoureteral reflux among children with urinary tract infections. Cochrane Database Syst Rev. 2016; 7:CD010657.
38. Mathews R, Carpenter M, Chesney R, Hoberman A, Keren R, Mattoo T, et al. Controversies in the management of vesicoureteral reflux: the rationale for the RIVUR study. J Pediatr Urol. 2009; 5:336–341.
39. Brandström P, Nevéus T, Sixt R, Stokland E, Jodal U, Hansson S. The Swedish reflux trial in children: IV. Renal damage. J Urol. 2010; 184:292–297.
40. Lim R. Vesicoureteral reflux and urinary tract infection: evolving practices and current controversies in pediatric imaging. AJR Am J Roentgenol. 2009; 192:1197–1208.
41. Parvex P, Willi JP, Kossovsky MP, Girardin E. Longitudinal analyses of renal lesions due to acute pyelonephritis in children and their impact on renal growth. J Urol. 2008; 180:2602–2606.
42. Grattan-Smith JD, Little SB, Jones RA. Evaluation of reflux nephropathy, pyelonephritis and renal dysplasia. Pediatr Radiol. 2008; 38:Suppl 1. S83–S105.
43. Chang SL, Caruso TJ, Shortliffe LD. Magnetic resonance imaging detected renal volume reduction in refluxing and nonrefluxing kidneys. J Urol. 2007; 178:2550–2554.
44. Lee SK, Chang Y, Park NH, Kim YH, Woo S. Magnetic resonance voiding cystography in the diagnosis of vesicoureteral reflux: comparative study with voiding cystourethrography. J Magn Reson Imaging. 2005; 21:406–414.
45. Arthurs OJ, Edwards AD, Joubert I, Graves MJ, Set PA, Lomas DJ. Interactive magnetic resonance voiding cystourethrography (iMRVC) for vesicoureteric reflux (VUR) in unsedated infants: a feasibility study. Eur Radiol. 2011; 21:1874–1881.
46. Kim JW, Lee CH, Yoo KH, Je BK, Kiefer B, Park YS, et al. Intravoxel incoherent motion magnetic resonance imaging to predict vesicoureteral reflux in children with urinary tract infection. Eur Radiol. 2016; 26:1670–1677.
47. Kis E, Nyitrai A, Várkonyi I, Máttyus I, Cseprekál O, Reusz G, et al. Voiding urosonography with second-generation contrast agent versus voiding cystourethrography. Pediatr Nephrol. 2010; 25:2289–2293.
48. Duran C, Valera A, Alguersuari A, Ballesteros E, Riera L, Martin C, et al. Voiding urosonography: the study of the urethra is no longer a limitation of the technique. Pediatr Radiol. 2009; 39:124–131.
49. Giordano M, Marzolla R, Puteo F, Scianaro L, Caringella DA, Depalo T. Voiding urosonography as first step in the diagnosis of vesicoureteral reflux in children: a clinical experience. Pediatr Radiol. 2007; 37:674–677.
50. Tse KS, Wong LS, Lau HY, Fok WS, Chan YH, Tang KW, et al. Paediatric vesicoureteric reflux imaging: where are we? Novel ultrasound-based voiding urosonography. Hong Kong Med J. 2014; 20:437–443.
51. Strimbu K, Tavel JA. What are biomarkers? Curr Opin HIV AIDS. 2010; 5:463–466.
52. Lee RS. Biomarkers for pediatric urological disease. Curr Opin Urol. 2009; 19:397–401.
53. Kitao T, Kimata T, Yamanouchi S, Kato S, Tsuji S, Kaneko K. Urinary biomarkers for screening for renal scarring in children with febrile urinary tract infection: Pilot Study. J Urol. 2015; 194:766–771.
54. Ai JW, Liu Y, Zeng XT, Lei Q, Zou L, Pei B. Angiotensin converting enzyme gene insertion/deletion polymorphism and vesicoureteral reflux in children: a meta-analysis of 14 casecontrol studies. Medicine (Baltimore). 2015; 94:e2421.
55. Mahyar A, Ayazi P, Yarigarravesh MH, Khoeiniha MH, Oveisi S, Sahmani AA, et al. Serum interleukin -8 is not a reliable marker for prediction of vesicoureteral reflux in children with febrile urinary tract infection. Int Braz J Urol. 2015; 41:1160–1166.
56. Parmaksız G, Noyan A, Dursun H, İnce E, Anarat R, Cengiz N. Role of new biomarkers for predicting renal scarring in vesicoureteral reflux: NGAL, KIM-1, and L-FABP. Pediatr Nephrol. 2016; 31:97–103.
57. Leroy S, Bouissou F, Fernandez-Lopez A, Gurgoze MK, Karavanaki K, Ulinski T, et al. Prediction of high-grade vesicoureteral reflux after pediatric urinary tract infection: external validation study of procalcitonin-based decision rule. PLoS One. 2011; 6:e29556.
58. Simões e, Valério FC, Vasconcelos MA, Miranda DM, Oliveira EA. Interactions between cytokines, congenital anomalies of kidney and urinary tract and chronic kidney disease. Clin Dev Immunol. 2013; 2013:597920.
59. Coulthard MG. Do kidneys outgrow the risk of reflux nephropathy? Pediatr Nephrol. 2002; 17:477–480.
60. Craig JC, Wheeler DM, Irwig L, Howman-Giles RB. How accurate is dimercaptosuccinic acid scintigraphy for the diagnosis of acute pyelonephritis? A meta-analysis of experimental studies. J Nucl Med. 2000; 41:986–993.