Abstract
Purpose
Computed tomography (CT) is the gold standard imaging modality for the diagnosis and follow-up of urolithiasis. Before the use of CT, intravenous urography (IVU) was the imaging modality of choice. CT remains contentious because of the cancer risk related to radiation exposure above a threshold level. We aimed to compare the radiation exposure dose to the average patient with urolithiasis in the era of CT with that of IVU.
Materials and Methods
Our hospital medical records database was searched for patients who presented to the Emergency Department over a 1-month period in 1990 with a diagnosis of renal colic. Patients with the same presentation, from the same month, in 2013 were also identified. A total of 14 patients from each year fulfilled the inclusion criteria. The estimated effective radiation exposure dose for each patient was calculated by using data from population-based studies.
Results
The median effective radiation dose per patient in the 1990 group, for initial diagnosis and subsequent follow-up, was 4.05 mSv (interquartile range [IQR], 3.7–4.4 mSv). The corresponding median dose in the 2013 group was 4.2 mSv (IQR, 4.2–4.9 mSv), and there was no evidence of a statistical difference between the groups (p=0.8).
Conclusions
Despite the contentiousness related to the use of serial CT scanning, our study demonstrated that for radiological investigation and follow-up of urolithiasis, the estimated effective radiation exposure dose to each patient is only marginally higher than in the era of IVU, with improvements in length of hospital stay and time to definitive diagnosis.
Noncontrast abdominal computed tomography (CTKUB) is the gold standard imaging modality in the diagnosis and follow-up of urolithiasis [1]. Prior to the use of computed tomography (CT), intravenous urography (IVU) was the imaging modality of choice [2]. With the development of helical CT scanning, there has been a shift away from the use of labor-intensive and less accurate IVU [3]. However, a perception exists that much higher radiation doses are being delivered to patients in the era of CT. Even with modern CT scanners, some believe that patients are being exposed to unacceptably high doses of radiation, largely as a result of a combination of "over scanning" and failing to appropriately utilize alternative imaging modalities.
Although technological advances and further understanding of radiation physics have led to reductions in patient radiation dose exposure per CT series, the widespread use of serial CT scanning for urolithiasis remains contentious because of the cancer risk related to radiation exposure above a threshold level [4]. We sought to quantify the approximate radiation exposure dose that the average patient received following presentation to our hospital with renal colic in the era of widespread helical CT scanning and to compare this with the radiation exposure dose a patient received in the era of IVU. We then reviewed the current literature on this topic.
Using our institutional medical records database, we searched for all patients who presented to the Emergency Department of our hospital over an arbitrarily chosen 1-month period (March) in 1990 with a diagnosis of presumed renal colic and were subsequently investigated. All patients who had a final diagnosis of renal colic from the Emergency Department were included, regardless of how this diagnosis was made. We repeated the search for patients who presented to the emergency department in March 2013. The acquisition of these data, and their subsequent analysis, did not require Human Research Ethics Committee (HREC) review on the basis that the project was categorized as a quality activity in accordance with the definition of research outlined in the Australian National Statement. An HREC number was provided for publication purposes (HREC/15/QGC/221).
A total of 14 patients were identified from March 1990 and 14 patients were identified from March 2013. The imaging at presentation and any other follow-up imaging of these patients was reviewed. We noted the time to definitive diagnosis, length of hospital stay, interventions required if any, and any adverse events associated with this presentation. We also noted the length of follow-up of each episode of stone disease. The estimated effective radiation dose exposure for each patient was calculated by using data from population-based studies. The assumed effective radiation doses for each individual CT, IVU, and plain abdominal X-ray (XRKUB) were 3.5, 3, and 0.7 mSv, respectively [56].
We compared the effective radiation dose between the 2 cohorts by using the nonparametric Wilcoxon rank sum test owing to the inherent discontinuities in the dose variable caused by the discrete values of radiation from each imaging modality. Medians and interquartile ranges (IQRs) for dose are reported. Mean values for number of follow-up imaging episodes were reported instead of median values because noninteger values were considered more illustrative. Analysis was performed by using Stata 12.0 (StataCorp LP., College Station, TX, USA).
The median effective radiation dose per patient in the 1990 group, for initial diagnosis and subsequent follow-up, was 4.05 mSv (IQR, 3.7–4.4 mSv). The corresponding median dose in the 2013 group was 4.2 mSv (IQR, 4.2–4.9 mSv), and no evidence of a statistical difference was observed between the groups (p=0.8).
The majority of patients in the 1990 cohort (79%) received an XRKUB on first presentation to the hospital in contrast with the 2013 cohort in which 43% received an XRKUB at presentation.
There was weak evidence (p=0.14) suggesting a higher radiation exposure from imaging at initial presentation in the 2013 cohort (median, 3.5 mSv; IQR, 3.5–4.2 mSv) versus the 1990 patients (median, 3.35 mSv; IQR, 0.7–3.7 mSv). This higher radiation exposure was accounted for by a proportionally higher number of higher-dose imaging modalities (CTKUB in 2013 or IVP in 1990) being performed in the 2013 group. For example, 93% of patients in the 2013 cohort had a CTKUB series at initial presentation and only 64% of patients in the 1990 cohort had an IVP at initial presentation. In the balance of 1990 cases, diagnosis was alternatively made on clinical history in lieu of IVP imaging or based on positive plain film findings. For the patients in 1990 who had an IVP at initial visit, there was a delay to receiving this imaging, and thus definitive diagnosis, that ranged from 0 to 3 days (mean, 0.93 days).
In the 2013 group, 57% of patients had a CTKUB as a first-line investigation in the absence of any prior plain film imaging. A total of 36% had a concomitant XRKUB and one patient (23-year-old male) in the 2013 group had a renal tract ultrasound for diagnosis. Unlike in the 1990 group, there was no delay to definitive diagnostic imaging in 2013. Diagnosis of urolithiasis in all patients in this group was made within the emergency department and did not exceed 24 hours in any case. All patients in the 2013 cohort who underwent CT scanning had a noncontrast CT. No patient had helical imaging that involved multiple phases or contrast media.
Follow-up imaging was more common in the 1990 cohort than in the 2013 cohort (mean: 1.71 vs. 1.36 follow-up imaging episodes per patient). Furthermore, a higher radiation dose follow-up scan (CTKUB or IVP) was more likely in the 1990 cohort (mean: 0.43 vs. 0.14 imaging episodes per patient), although neither difference reached conventional significance. The study findings are summarized in Table 1.
The patients in this series did not appear to receive greater radiation dosages contemporaneously in the management of urolithiasis than they did in 1990, despite the now widespread use of noncontrast helical CT scanning. This observation should allay some concerns about the potential for current best-practice management of urolithiasis to result in future complications related to radiation exposure. These data do suggest, however, that contemporary imaging practices tend to deliver slightly higher radiation doses in the initial assessment and diagnosis of urolithiasis. The earlier higher radiation burden in the 2013 cohort did appear to be associated with fewer follow-up images. This may reflect the ability of higher-sensitivity imaging, in the form of CTKUB, to more accurately guide follow-up and management of urolithiasis compared with plain film imaging. Cumulative radiation exposure in the 1990 cohort, although similar to the 2013 cohort, tended to be focused more in the follow-up period.
The authors acknowledge the innate limitations of this study given the small study population. It was not our intention, however, to make a precise and definitive measure of the difference in radiation exposure between the 2 cohorts but rather to highlight whether there was a large disparity between the groups, because this is the view of many clinicians and nonclinicians.
Multiple publications have addressed cancer risk related to radiation exposure from radiological procedures and investigations [78]. Data from population-based studies collected from victims of nuclear disasters would suggest that an effective radiation dose of 50 to 200 mSv is linked to an increased risk of various malignancies [9]. Evidence of carcinogenicity with effective radiation doses at these levels has led to paradigm shifts in the management of certain malignancies, such as stages I and IIa testicular seminoma, where there has been a decline in the use of routine radiation therapy in favor of chemotherapy alone following surgery [1011]. Evidence of carcinogenicity for effective doses between 10 and 50 mSv is less definitive, however [12]. The estimated average yearly radiation exposure dose from background radiation in Australia, the United Kingdom, and the United States is 2, 2.7, and 3 mSv, respectively [1314].
The annual permitted occupational radiation dose exposures in Europe and the United States are 20 mSv and 50 mSv, respectively [1516]. In both the contemporary and the historic renal stone evaluation cohorts we reviewed, no patient in either group reached radiation exposure doses approximating these levels.
The perception of increased radiation dosages related to contemporary CT-based imaging practices has been widely publicized and reviewed [1718]. Studies have suggested that some medical practitioners have suboptimal knowledge of the radiation exposure related to various investigations and of the consequent associated cumulative patient risk [1920]. This may lead to over-utilization of CT as an imaging modality. The clinical indication for serial scanning has been appropriately questioned. It has also been noted that many publications on this topic suggesting an overuse of CT were authored by nonclinicians, which therefore calls into question whether data on "unjustified" scans are indeed accurate [21].
It appears also that many of the studies discussing the potential over-utilization of CT and the resultant increased cancer risk to patients were population-based studies where all sources of referral were captured [2223]. These studies therefore contain data where abdominal CT scans, for example, are ordered in an emergency department setting as a "catchall" approach to the investigation of acute abdominal pain. Many of the CTs in these studies include contrast-enhanced multiphase scans with the resultant increased radiation exposure dose to the patient. It is debatable whether conclusions drawn from these studies are directly applicable to the investigation of urolithiasis in a urology department, where imaging is most often ordered by a urologist for a clear stone-related indication.
The use of magnetic resonance imaging (MRI) has been proposed as an alternative to CT for the investigation of many conditions as a way of avoiding the risk of malignancy related to CT scanning. Although MRI does have applicability as an alternative to CT in certain clinical contexts, such as ureteric calculi in pregnant women, it has not been found to be equivocal in the diagnosis and follow-up of urolithiasis, and for this reason has not been adopted routinely [24]. Renal tract ultrasound can play a major role in the diagnosis and follow-up of urolithiasis and has also been suggested as the initial imaging modality in certain clinical contexts [25]. The combination of renal tract ultrasound and XRKUB has been suggested as an option for follow-up of radio-opaque ureteric calculi. It has been shown that a normal renal tract ultrasound, without evidence of hydronephrosis, as an initial investigation in the setting of ureteric calculi is related to a high rate of success with conservative management [26]. The use of renal tract ultrasound in this context may negate the need for crosssectional imaging and the resultant radiation exposure.
However, renal tract ultrasound can overestimate the size of renal and ureteric calculi in up to 87% of cases, particularly for calculi less than 5 mm in size. This may lead to inappropriately high rates of urological intervention for smaller stones. It has also been shown that skin-to-stone distances are discordant between renal tract ultrasound and CT, making ultrasound less appropriate for planning prior to extracorporeal shockwave lithotripsy and percutaneous nephrolithotomy [27]. The 2014 European Association of Urology Guidelines state that contrast-enhanced CT is recommended in the planning of urological intervention if the anatomy of the renal collecting system needs to be assessed.
Newer imaging formats such as low-dose CT protocols can now achieve high-quality imaging with effective radiation doses as low as 0.5 mSv, which is similar to that of an XRKUB [28]. Technological advances, such as dual-energy CT with split bolus contrast means that "virtual" noncontrast and contrast-enhanced imaging can also be generated from a single scan with high sensitivity, thus reducing the radiation exposure dose to the patient while gleaning significant information about stone burden and renal collecting system anatomy [29]. Such techniques may serve to further reduce radiation dosages in future.
Although the sensitivity of CT has been shown to be superior to other imaging modalities such as renal tract ultrasound for visualization of renal tract calculi, its superiority in terms of improved clinical outcomes has not been proven in a large-scale comparative effectiveness trial. The Study of Tomography of Nephrolithiasis Evaluation is such a study in progress [30]. The results of this trial should assist urologists in choosing the most appropriate imaging modality in the management of urolithiasis in the future.
Despite the contentiousness related to the use of serial CT scanning, our study has demonstrated that for radiological investigation and follow-up of urolithiasis, in a single urology unit, the average estimated effective radiation exposure dose to each patient is only marginally higher than in the era of IVU, with improvements in length of hospital stay and time to definitive diagnosis. With continuing improvements in technology, the effective radiation dose from abdominal CT is likely to be reduced even further. In combination with the judicious use of CT scanning for appropriate clinical indications, this also should permit safe levels of radiation exposure in the management of urolithiasis in the future.
Figures and Tables
Table 1
Summary of the study findings
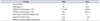
References
1. Sourtzis S, Thibeau JF, Damry N, Raslan A, Vandendris M, Bellemans M. Radiologic investigation of renal colic: unenhanced helical CT compared with excretory urography. AJR Am J Roentgenol. 1999; 172:1491–1494.
2. Smith RC, Rosenfield AT, Choe KA, Essenmacher KR, Verga M, Glickman MG, et al. Acute flank pain: comparison of non-contrast-enhanced CT and intravenous urography. Radiology. 1995; 194:789–794.
3. Lim GS, Jang SH, Son JH, Lee JW, Hwang JS, Lim CH, et al. Comparison of non-contrast-enhanced computed tomography and intravenous pyelogram for detection of patients with urinary calculi. Korean J Urol. 2014; 55:120–123.
4. Brenner DJ, Hall EJ. Computed tomography: an increasing source of radiation exposure. N Engl J Med. 2007; 357:2277–2284.
5. Mettler FA Jr, Huda W, Yoshizumi TT, Mahesh M. Effective doses in radiology and diagnostic nuclear medicine: a catalog. Radiology. 2008; 248:254–263.
6. Drake T, Jain N, Bryant T, Wilson I, Somani BK. Should low-dose computed tomography kidneys, ureter and bladder be the new investigation of choice in suspected renal colic? A systematic review. Indian J Urol. 2014; 30:137–143.
7. Linet MS, Slovis TL, Miller DL, Kleinerman R, Lee C, Rajaraman P, et al. Cancer risks associated with external radiation from diagnostic imaging procedures. CA Cancer J Clin. 2012; 62:75–100.
8. Mettler FA Jr, Wiest PW, Locken JA, Kelsey CA. CT scanning: patterns of use and dose. J Radiol Prot. 2000; 20:353–359.
9. United Nations Scientific Committee on the Effects of Atomic Radiation. UNSCEAR 2000 REPORT Vol. I. Sources and effects of ionizing radiation. UNSCEAR 2000 Report to the General Assembly, with scientific annexes. Annex D: medical radiation exposure. Vienna: UNSCEAR;2007. p. 375–377.
10. Horwich A, Fossa SD, Huddart R, Dearnaley DP, Stenning S, Aresu M, et al. Second cancer risk and mortality in men treated with radiotherapy for stage I seminoma. Br J Cancer. 2014; 110:256–263.
11. Chung P, Warde P. Contemporary management of stage I and II seminoma. Curr Urol Rep. 2013; 14:525–533.
12. Shore RE. Radiation impacts on human health: certain, fuzzy, and unknown. Health Phys. 2014; 106:196–205.
13. National Council on Radiation Protection and Measurements (NCRPM). Ionising radiation exposure of the population of the Unites States (2009). NCRP report No. 160. Bethesda (MD): NCRPM;2009.
14. Public Health England. Guidance: Ionising radiation: dose comparisons. London: Public Health England;Published 18 March 2011 [Internet]. cited 2015 Feb 14. Available from: https://www.gov.uk/government/publications/ionising-radiation-dose-comparisons/ionising-radiation-dose-comparisons.
15. International basic safety standards for protection against ionizing radiation and for the safety of radiation sources. Vienna: International Atomic Energy Agency;1996. cited 2015 Feb 14. http://www.ilo.org/wcmsp5/groups/public/@ed_protect/@protrav/@safework/documents/publication/wcms_152685.pdf.
16. NRC Regulations (10 CFR) Part 20: standards for protection against radiation: subpart C, occupational dose limits. Washington, DC: US Nuclear Regulatory Commission;cited 2015 Feb 14. Available from: http://www.nrc.gov/reading-rm/doc-collections/cfr/part020/full-text.html.
17. Smith-Bindman R, Miglioretti DL, Johnson E, Lee C, Feigelson HS, Flynn M, et al. Use of diagnostic imaging studies and associated radiation exposure for patients enrolled in large integrated health care systems, 1996-2010. JAMA. 2012; 307:2400–2409.
18. Berrington de Gonzalez A, Mahesh M, Kim KP, Bhargavan M, Lewis R, Mettler F, et al. Projected cancer risks from computed tomographic scans performed in the United States in 2007. Arch Intern Med. 2009; 169:2071–2077.
19. Keijzers GB, Britton CJ. Doctors' knowledge of patient radiation exposure from diagnostic imaging requested in the emergency department. Med J Aust. 2010; 193:450–453.
20. Barbic D, Barbic S, Dankoff J. An exploration of Canadian emergency physicians' and residents' knowledge of computed tomography radiation dosing and risk. CJEM. 2015; 17:131–139.
21. Schwartz DT. Counter-Point: are we really ordering too many CT scans? West J Emerg Med. 2008; 9:120–122.
22. Mathews JD, Forsythe AV, Brady Z, Butler MW, Goergen SK, Byrnes GB, et al. Cancer risk in 680,000 people exposed to computed tomography scans in childhood or adolescence: data linkage study of 11 million Australians. BMJ. 2013; 346:f2360.
23. Smith-Bindman R, Lipson J, Marcus R, Kim KP, Mahesh M, Gould R, et al. Radiation dose associated with common computed tomography examinations and the associated lifetime attributable risk of cancer. Arch Intern Med. 2009; 169:2078–2086.
24. Kalb B, Sharma P, Salman K, Ogan K, Pattaras JG, Martin DR. Acute abdominal pain: is there a potential role for MRI in the setting of the emergency department in a patient with renal calculi? J Magn Reson Imaging. 2010; 32:1012–1023.
25. Türk C, Knoll T, Petrik A, Sarica K, Skolarikos A, Straub M, et al. Guidelines on urolithiasis [Internet]. Anheim (NL): European Association of Urology;c2014. cited 2015 Aug 5. Available from:http://uroweb.org/wp-content/uploads/22-Urolithiasis_LR.pdf.
26. Yan JW, McLeod SL, Edmonds ML, Sedran RJ, Theakston KD. Normal renal sonogram identifies renal colic patients at low risk for urologic intervention: a prospective cohort study. CJEM. 2015; 17:38–45.
27. Ray AA, Ghiculete D, Pace KT, Honey RJ. Limitations to ultrasound in the detection and measurement of urinary tract calculi. Urology. 2010; 76:295–300.
28. McLaughlin PD, Murphy KP, Hayes SA, Carey K, Sammon J, Crush L, et al. Non-contrast CT at comparable dose to an abdominal radiograph in patients with acute renal colic; impact of iterative reconstruction on image quality and diagnostic performance. Insights Imaging. 2014; 5:217–230.
29. Toepker M, Kuehas F, Kienzl D, Herwig R, Spazierer E, Krauss B, et al. Dual energy computerized tomography with a split bolus-a 1-stop shop for patients with suspected urinary stones? J Urol. 2014; 191:792–797.
30. Valencia V, Moghadassi M, Kriesel DR, Cummings S, Smith-Bindman R. Study of tomography of nephrolithiasis evaluation (STONE): methodology, approach and rationale. Contemp Clin Trials. 2014; 38:92–101.
This article compared the amount and frequency of radiation exposure of patients between the year 1990 and 2013 during diagnostic procedures due to the change in imaging equipment. Considering the rise in interest of the safety issues concerning over-exposure of radiation, this paper merits further consideration.
Though it is difficult to draw a definitive conclusion due to the small case numbers, the authors were unable to show that there was a clear disparity in radiation exposure between the 2 groups. Simple comparison may show that patients undergoing computed tomography (CT) scans are exposed to higher amounts of radiation than intravenous urography (IVU). However CT scans allow faster diagnosis and requires less concurrent studies and thus the overall exposure is not very different. I believe this the message that the authors wish to present to the readers. If possible, remarks on the composition of stones that were examined are advised.
The authors mention in their manuscript that the radiation exposure during CT scans were approximately 3.5 mSV, which is probably a protocol for low dose CT scans. The amount of radiation exposure for traditional renal stone protocol is approximately 9–16 mSV which is considerably higher [1]. Many patients will likely have recurrences and will need additional scans, adding to radiation exposure. A study on the frequency of CT scans reports that patients with acute stone episodes will require an average of 1.7 CT scans within the following year [2].
The diagnostic imaging method the authors used for comparison in 1990 was in fact an IVU. The IVU is a relatively invasive procedure with risks concerning complication due to contrast. The IVU is also slow in diagnosis and is currently no longer used in practice.
No studies have yet reported the effects of continuous low dose radiation exposure, but due to advancement of diagnostic imaging tools, the amount of radiation exposure is increasing. It has been reported in the United States there has been a 600% (from 0.54 to 3.0) increase in radiation exposure from medical sources per capita from the year 1982 to 2006 [3]. This concern is augmented in ureter stone patients where radiation is frequently used during diagnosis, treatment and follow-up. Considering the high rate of recurrence in ureter stones, urologists should always be concerned of the risk of overexposure in their patients and try to minimize the amount of radiation during procedures. Recent studies on low dose (3 mSv), and ultra-low dose (1–2 mSv) CT scans show that even with low amounts of radiation, stones larger than 3 mm in patients with body mass index less than 30 can be detected with comparable sensitivity and specificity to standard CT scans [456]. Fluoroscopy used during percutaneous nephrolithotomy, ureteroscopy or extracorporeal shock-wave lithotripsy is also a major source of medical radiation and should follow the "as low as reasonably achievable" principle whenever possible during procedures to lessen the chance of radiation exposure.
References
1. Cabrera F, Preminger GM, Lipkin ME. As low as reasonably achievable: Methods for reducing radiation exposure during the management of renal and ureteral stones. Indian J Urol. 2014; 30:55–59.
2. Ferrandino MN, Bagrodia A, Pierre SA, Scales CD Jr, Rampersaud E, Pearle MS, et al. Radiation exposure in the acute and short-term management of urolithiasis at 2 academic centers. J Urol. 2009; 181:668–672.
3. Mettler FA Jr, Thomadsen BR, Bhargavan M, Gilley DB, Gray JE, Lipoti JA, et al. Medical radiation exposure in the U.S. in 2006: preliminary results. Health Phys. 2008; 95:502–507.
4. Tack D, Sourtzis S, Delpierre I, de Maertelaer V, Gevenois PA. Low-dose unenhanced multidetector CT of patients with suspected renal colic. AJR Am J Roentgenol. 2003; 180:305–311.
5. Kim BS, Hwang IK, Choi YW, Namkung S, Kim HC, Hwang WC, et al. Low-dose and standard-dose unenhanced helical computed tomography for the assessment of acute renal colic: prospective comparative study. Acta Radiol. 2005; 46:756–763.
6. Poletti PA, Platon A, Rutschmann OT, Schmidlin FR, Iselin CE, Becker CD. Low-dose versus standard-dose CT protocol in patients with clinically suspected renal colic. AJR Am J Roentgenol. 2007; 188:927–933.