Abstract
Purpose
To identify independent patient factors associated with an increased radiation dose, and to evaluate the effect of patient position on the effective dose in abdominal digital radiography.
Materials and Methods
We retrospectively evaluated the effective dose for abdominal digital radiography in 222 patients. The patients were divided into two groups based on the cut-off dose value of 0.311 mSv (the upper third quartile of dose distribution): group A (n = 166) and group B (n = 56). Through logistic regression, independent factors associated with a larger effective dose were identified. The effect of patient position on the effective dose was evaluated using a paired t-test.
Results
High body mass index (BMI) (≥ 23 kg/m2), presence of ascites, and spinal metallic instrumentation were significantly associated with a larger effective dose. Multivariate logistic regression analysis revealed that high BMI [odds ratio (OR), 25.201; p < 0.001] and ascites (OR, 25.132; p < 0.001) were significantly associated with a larger effective dose. The effective dose was significantly lesser (22.6%) in the supine position than in the standing position (p < 0.001).
Radiation exposure during diagnostic radiologic examinations is an emerging problem in the United States (1). Among the various types of diagnostic radiologic examinations, digital radiography has become one of the most commonly used techniques. By using automatic exposure control (AEC), some clinical investigators have reported that digital radiography provides a substantial reduction in the radiation dose while maintaining sufficient diagnostic image quality (2345). However, there is a potential risk of a very high radiation dose being given to oversized patients when an AEC mode is used. Since the exposure time in the AEC mode increases in accordance with the thickness of the patient's body in order to maintain image quality, obese patients are exposed to a greater radiation dose than thinner patients (67).
Previous studies have shown that a patient's body size, including the abdominal fat thickness, can influence the radiation dose in abdominal radiologic examinations (789101112); however, only a few studies have reported about the influence of patient factors on the effective dose in plain radiography. In addition, metallic devices, such as pancreaticobiliary or gastrointestinal stents, endovascular devices, or spinal metallic instruments, may cause an increase in the radiation dose in patients undergoing abdominal radiography. Therefore, the purpose of this study was to identify independent factors associated with an increased radiation dose and to evaluate the effect of the patient's position on the effective dose in abdominal digital radiography.
From October 2013 to December 2013, we retrospectively analyzed the data from 242 patients who underwent digital abdominal radiography at our hospital. This retrospective study was approved by the institutional review board of our hospital, and the requirement for obtaining informed consent was waived owing to the retrospective nature of the study.
After excluding 20 patients aged < 15 years, 222 adult patients who underwent abdominal radiography in both the supine and standing positions were included in this study. Since there are no reported standardized values to indicate a “high effective dose in abdominal digital radiography” in previous studies, we divided the study patients by using the upper third quartile value (0.311 mSv) of the effective dose in all study patients: group A (n = 166, effective dose < 0.311 mSv) and group B (n = 56, effective dose ≥ 0.311 mSv). We then evaluated the two groups according to the following factors: patient age, body mass index (BMI), sex, ascites, presence of an abdominal stent or tube, and spinal metallic instrumentation. In our study, BMI was classified into two categories by using the cut-off value of 23 kg/m2 for statistical analysis according to the International Association for the Study of Obesity, the International Obesity Task Force, because the criteria for overweight and obesity for adult Asians are not consistent with those for non-Asians.
We used a commercial digital radiography system with cesium iodide-amorphous silicon, flat-panel detector (Definium 8000; GE Healthcare, Chalfont St. Giles, UK) in this study. The AEC of the flat-panel detector system was adjusted to a speed class of 200. The three AEC chambers were used in all patients. To compare the effective dose in terms of BMI, presence of ascites, or overlapping metallic instruments in the body area, we also measured the output parameters including milliampere second (mAs) and the dose-area product (DAP). DAP was measured by the DAP meter that was mounted on the tube collimator, and mAs was recorded from Digital Imaging and Communications in Medicine (DICOM) images. This study was performed with 80 kVp, field size (35 × 41 cm), and inherent beam filtration of 3.1-mm aluminum at a fixed source-to-image distance of 120 cm. We performed phantom equipment testing and calibration for both DAP and AEC every 3 months to ensure stability of the systems.
DAP is defined as the product of the irradiated area multiplied by the absorbed dose in air (1314). The DAP value was obtained from the DAP meter (PD-8100; Toreck Co. Ltd., Yokohama, Japan) that was mounted on the tube collimator. The effective dose for each patient was calculated by using a PC-based, Monte Carlo (PCXMC version 2.0; STUK, Helsinki, Finland) program. PCXMC is based on Monte Carlo simulation and is intended for calculating patients' organ doses and the effective dose in radiologic examinations (1516). In PCXMC, the exposure techniques, patient height, patient weight, and irradiation geometry were entered, including the DAP value. The simulation was performed with 2 × 104 photons. The simulation conditions were identical to the actual exposure conditions. The effective dose was determined by using the revised tissue-weighting factors of the International Commission on Radiological Protection (publication 103) (17).
In this study, the mean effective dose in one patient was defined as the mean value of the effective doses obtained in the supine and standing positions. The presence of ascites was defined in accordance with the diagnosis from prior computed tomography (CT) or ultrasound (US) examinations. The patient age, BMI, and sex, and the presence of ascites, abdominal stent/tube or spinal metallic instrumentation were analyzed in groups A and B by using the chi-square test or Fisher's exact test. To compare the effects of patient position on the effective dose, the paired t-test was used. Continuous variables, including age and BMI, were classified into two subgroups by using the cut-off values of 65 years for age and 23 kg/m2 for BMI, and were analyzed with the chi-square test or Fisher's exact test. Logistic regression analysis was performed to identify independent factors associated with patients who received a high effective dose. Factors with a p value < 0.05 were entered into the multivariate logistic regression analysis. All statistical analyses were performed with Statistical Package for Social Sciences (SPSS) version 21 (SPSS Inc., Chicago, IL, USA). A two-sided p value < 0.05 indicated statistical significance.
The patient characteristics, effective dose, and DAP value in both groups are summarized in Table 1. The median effective dose for the total patient material was 0.224 mSv (interquartile range, 0.172–0.311 mSv). In group A, the types of abdominal stents or tubes used in the patients were as follows: percutaneous transhepatic biliary drainage (PTBD) tube (n = 9); endoscopic nasobiliary drainage (ENBD) tube (n = 7); pancreatico-biliary stent (n = 7); ureteral stent (n = 4); gastrointestinal stent (n = 3); and inferior vena cava (IVC) stent (n = 1). In group B, the types of stents or tubes used were as follows: pancreaticobiliary stent (n = 6); PTBD tube (n = 4); ENBD tube (n = 2); IVC stent (n = 1); and ureteral stent (n = 1). In the nine patients with spinal metallic instrumentation, screws and instruments were used for posterior fixation of the lumbar spine. The median time interval from CT (n = 10) or US (n = 6) showing ascites and study radiographs was 3 days (interquartile range, 1.00–5.25 days). All values including mAs, DAP value, and effective dose in group A were significantly lower than those in group B (p < 0.001). The effective dose for the total patient population, group A, and group B was 0.255, 0.196, and 0.431 mSv, respectively. In group B, the mean BMI was significantly higher than that in group A. The distribution of the effective dose for both the supine and standing positions according to BMI in the total patient population is shown in Fig. 1. Compared with the effective dose for patients with the lowest BMI (13.8 kg/m2), the effective dose for patients with the highest BMI (31.3 kg/m2) was nine times higher (0.069 mSv vs. 0.653 mSv). Ascites and spinal metallic instrumentation were significantly more frequent in group B than in group A. The highest value of the mean effective dose per patient was 1.127 mSv and the value was 17 times higher than the lowest value (0.069 mSv) of the mean effective dose in this study.
On univariate analysis, patient age, sex, and presence of an abdominal stent or tube were not associated with an increase in the effective dose. However, high BMI [odds ratio (OR), 14.327; 95% confidence interval (CI), 6.314–32.509; p < 0.001], presence of ascites (OR, 7.871; 95% CI, 2.601–23.826; p < 0.001), and spinal metallic instrumentation (OR, 6.520; 95% CI, 1.573–27.019; p = 0.009) were significantly associated with an increase in the effective dose (p < 0.001, p < 0.001, and p = 0.009, respectively) (Table 2). Multivariate logistic regression analysis revealed that high BMI (OR, 25.201; 95% CI, 8.576–74.051; p < 0.001) and ascites (OR, 25.132; 95% CI, 5.381–117.376; p < 0.001) were significantly associated with an increase in the effective dose.
The distribution of the effective doses according to the patient position in groups A and B is demonstrated in the box plot presented in Fig. 2. In the total patient population, a significant effective dose reduction (22.6%) was noted when the patient was placed in the supine position than in the standing position (p < 0.001). Among them, patients with BMI 23 kg/m2 in the supine position received a lower effective dose than those in the standing position (supine: 0.296 mSv vs. standing: 0.382 mSv). In addition, the effective dose in patients with ascites was significantly lower (15.3%) in the supine position than in the standing position (supine: 0.384 mSv vs. standing: 0.453 mSv). The difference in the effective doses was statistically significant (p < 0.001) (Table 3) not only in the comparison of the effective dose for patients with BMI < 23 kg/m2 both in the supine and standing positions, but also in the comparison of the effective dose for patients without ascites in both positions.
In this study, we showed that the presence of ascites and a high BMI are independent factors for an increase in the effective dose in patients undergoing abdominal digital radiography. High density materials, such as an abdominal stent/tube or spinal metallic instruments in the abdominal area, were not significantly associated with an increased effective dose.
In previous studies, BMI was an important determinant of the radiation dose and overweight patients generally received a larger radiation dose during radiographic examinations (1118). Not only in abdominal radiography but also in ureteroscopy, patients with severe obesity received a three-fold higher radiation dose than patients without obesity (12).
The presence of ascites was also significantly associated with an increased effective dose. This finding is useful for identifying the methods for reducing patients' radiation dose as no study has reported that ascites has an influence on the effective dose in abdominal digital radiography. For example, in this study, a patient with massive ascites received the highest effective dose (1.127 mSv) despite having a relatively normal BMI (22.7 kg/m2). Considering the influence of BMI and ascites on abdominal radiography, these factors should be considered in order to reduce the effective dose.
With respect to the effect of patient position on the effective dose, there was a significant dose reduction (22.6%) in the supine position than in the standing position. This finding agrees with that of a previous study with respect to the effects of the patient's position on the effective dose (18). The previous study indicated that if the distribution of subcutaneous fat changes when a patient is placed in the supine position, only the thickness of the fat remaining within the X-ray field affects the patient's effective dose. In addition, as some of the adipose tissue may move to a lateral position if the patient's position is supine, lateral fat has little or no influence on the effective dose. Therefore, the authors claimed that the standing position during an abdominal radiographic examination results in a higher effective dose than the supine position. Although abdominal radiography was previously commonly performed in both the supine and standing positions, some radiologists suggest that this practice should perhaps be discontinued because of the excessive radiation dose (19). They also proposed that when a patient has an acute abdomen and pneumoperitoneum, only a single, supine abdominal radiograph should be performed (1920). However, to the best of our knowledge, there are no specific indications for selecting the position for abdominal digital radiography in patients with a high BMI and ascites. Thus, considering our results and the findings from previous studies, a single abdominal radiograph with the patient placed in the supine position should be considered in order to reduce the effective dose in patients with a high BMI and ascites. Some studies have suggested that the use of extra copper filtration and increasing the beam energy can also reduce the patient's dose (2122). However, it should be noted that increased energy may affect image quality because a higher proportion of Compton interactions and more forward scatter reach the image receptor.
This study has several limitations. First, as the number of patients who had received stents, tubes or spinal metallic instruments was relatively small, the effect of these materials on the radiation dose could not be demonstrated well. Although the presence of metallic instruments was not an independent factor for an increased effective dose in multivariate logistic regression analysis, the univariate analysis showed statistical significance. Thus, future studies with large number of patients or prospective studies would be beneficial to prove the exact influence of these materials on abdominal radiography. Second, we determined that the upper third quartile value (0.311 mSv) of the effective dose is the cut-off value of a high effective dose as there are no previously defined highly effective doses for abdominal digital radiography. Moreover, the upper third quartile value of the effective dose assessed in our study was lower than the mean effective dose reported in other studies (0.44–1.5 mSv) (1923242526). One possible reason why the effective dose in our study showed a low value compared with that in other published studies was the relatively low BMI unlike in the other studies. However, the fact that various types of equipment, techniques, parameter settings, and patient positions were used during abdominal examinations makes it difficult to explain the differences in the amount of the effective dose noted in previous studies. Third, the dose calculations in PCXMC are based on a standard phantom that can be scaled in accordance with patient weight. However, the presence of ascites could possibly change the relative position and the contents of the abdominal cavity, thus adding uncertainty to dose estimation for these patients. Nevertheless, our study suggests the possible factors influencing the increased effective dose in abdominal radiography, and it also suggests that future studies are required to reduce the radiation dose in patients undergoing radiographic examinations.
In conclusion, we showed that a high BMI and the presence of ascites were independent factors associated with an increased effective dose in abdominal radiography. For patients with a high BMI and ascites, the radiation dose was significantly lower for examination in the supine position than in the standing position. Therefore, significant dose reduction in patients with a high BMI or ascites may be achieved by placing the patient in the supine position during abdominal digital radiography.
Figures and Tables
Fig. 1
Scatterplot and quadratic curve fitting showing the distribution of the effective dose for both the supine and standing positions according to the patient's body mass index (BMI). The effective dose is significantly decreased in the supine position than in the standing position, especially in patients with BMI > 23 kg/m2 (red dotted line).
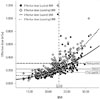
Fig. 2
Box-plot showing the distribution of the effective doses according to patient position in groups A and B.
*Outliers indicate values more than 1.5 times of upper quartile.
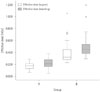
Table 1
Patient Characteristics, Effective Dose and DAP Value According to Patient Position
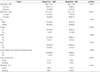
Table 2
Univariate and Multiple Logistic Regression Analysis to Identify the Independent Factors Associated with Increased Effective Dose of Abdominal Digital Radiography
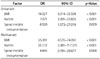
Table 3
Effective Dose in Abdominal Digital Radiography According to Patient Position, Presence of Ascites, and BMI

References
1. Mettler FA Jr, Thomadsen BR, Bhargavan M, Gilley DB, Gray JE, Lipoti JA, et al. Medical radiation exposure in the U.S. in 2006: preliminary results. Health Phys. 2008; 95:502–507.
2. Bacher K, Smeets P, Bonnarens K, De Hauwere A, Verstraete K, Thierens H. Dose reduction in patients undergoing chest imaging: digital amorphous silicon flat-panel detector radiography versus conventional film-screen radiography and phosphor-based computed radiography. AJR Am J Roentgenol. 2003; 181:923–929.
3. Bacher K, Smeets P, Vereecken L, De Hauwere A, Duyck P, De Man R, et al. Image quality and radiation dose on digital chest imaging: comparison of amorphous silicon and amorphous selenium flat-panel systems. AJR Am J Roentgenol. 2006; 187:630–637.
4. Grewal RK, Young N, Colins L, Karunnaratne N, Sabharwal N. Digital chest radiography image quality assessment with dose reduction. Australas Phys Eng Sci Med. 2012; 35:71–80.
5. Schaefer-Prokop C, Neitzel U, Venema HW, Uffmann M, Prokop M. Digital chest radiography: an update on modern technology, dose containment and control of image quality. Eur Radiol. 2008; 18:1818–1830.
6. Doyle P, Martin CJ. Calibrating automatic exposure control devices for digital radiography. Phys Med Biol. 2006; 51:5475–5485.
7. Kim H, Park M, Park S, Jeong H, Kim J, Kim Y. Estimation of absorbed organ doses and effective dose based on body mass index in digital radiography. Radiat Prot Dosimetry. 2013; 153:92–99.
8. Schindera ST, Nelson RC, Toth TL, Nguyen GT, Toncheva GI, DeLong DM, et al. Effect of patient size on radiation dose for abdominal MDCT with automatic tube current modulation: phantom study. AJR Am J Roentgenol. 2008; 190:W100–W105.
9. Chan VO, McDermott S, Buckley O, Allen S, Casey M, O'Laoide R, et al. The relationship of body mass index and abdominal fat on the radiation dose received during routine computed tomographic imaging of the abdomen and pelvis. Can Assoc Radiol J. 2012; 63:260–266.
10. Tung CJ, Lee CJ, Tsai HY, Tsai SF, Chen IJ. Body size-dependent patient effective dose for diagnostic radiography. Radiat Meas. 2008; 43:1008–1011.
11. Ector J, Dragusin O, Adriaenssens B, Huybrechts W, Willems R, Ector H, et al. Obesity is a major determinant of radiation dose in patients undergoing pulmonary vein isolation for atrial fibrillation. J Am Coll Cardiol. 2007; 50:234–242.
12. Hsi RS, Zamora DA, Kanal KM, Harper JD. Severe obesity is associated with 3-fold higher radiation dose rate during ureteroscopy. Urology. 2013; 82:780–785.
13. Guo H, Liu WY, He XY, Zhou XS, Zeng QL, Li BY. Optimizing imaging quality and radiation dose by the age-dependent setting of tube voltage in pediatric chest digital radiography. Korean J Radiol. 2013; 14:126–131.
14. Nickoloff EL, Lu ZF, Dutta AK, So JC. Radiation dose descriptors: BERT, COD, DAP, and other strange creatures. Radiographics. 2008; 28:1439–1450.
15. Khelassi-Toutaoui N, Berkani Y, Tsapaki V, Toutaoui AE, Merad A, Frahi-Amroun A, et al. Experimental evaluation of PCXMC and prepare codes used in conventional radiology. Radiat Prot Dosimetry. 2008; 131:374–378.
16. Kiljunen T, Tietäväinen A, Parviainen T, Viitala A, Kortesniemi M. Organ doses and effective doses in pediatric radiography: patient-dose survey in Finland. Acta Radiol. 2009; 50:114–124.
17. The 2007 Recommendations of the International Commission on Radiological Protection. ICRP publication 103. Ann ICRP. 2007; 37:1–332.
18. Yanch JC, Behrman RH, Hendricks MJ, McCall JH. Increased radiation dose to overweight and obese patients from radiographic examinations. Radiology. 2009; 252:128–139.
19. James B, Kelly B. The abdominal radiograph. Ulster Med J. 2013; 82:179–187.
20. Levine MS, Scheiner JD, Rubesin SE, Laufer I, Herlinger H. Diagnosis of pneumoperitoneum on supine abdominal radiographs. AJR Am J Roentgenol. 1991; 156:731–735.
21. Smans K, Struelens L, Hoornaert MT, Bleeser F, Buls N, Berus D, et al. A study of the correlation between dose area product and effective dose in vascular radiology. Radiat Prot Dosimetry. 2008; 130:300–308.
22. Doherty P, O'Leary D, Brennan PC. Do CEC guidelines under-utilise the full potential of increasing kVp as a dose-reducing tool? Eur Radiol. 2003; 13:1992–1999.
23. Teeuwisse W, Geleijns J, Veldkamp W. An inter-hospital comparison of patient dose based on clinical indications. Eur Radiol. 2007; 17:1795–1805.
24. Wall BF, Hart D. Revised radiation doses for typical X-ray examinations. Report on a recent review of doses to patients from medical X-ray examinations in the UK by NRPB. National Radiological Protection Board. Br J Radiol. 1997; 70:437–439.
25. Chen TR, Tyan YS, Teng PS, Chou JH, Yeh CY, T WE, et al. Population dose from medical exposure in Taiwan for 2008. Med Phys. 2011; 38:3139–3148.
26. Mettler FA Jr, Huda W, Yoshizumi TT, Mahesh M. Effective doses in radiology and diagnostic nuclear medicine: a catalog. Radiology. 2008; 248:254–263.