Abstract
Purpose
The purpose of this study was to evaluate the diagnostic performance of acoustic radiation force impulse (ARFI) elasticity imaging.
Materials and Methods
One hundred and twenty-seven thyroid nodules were examined
by both ARFI elastography and B-mode sonography. Virtual Touch tissue quantification (VTQ) values of the thyroid nodules were measured. Scoring of B-mode sonographic findings of each thyroid nodules was performed. The sums of these VTQ and the B-mode scores were determined. The comparative diagnostic performances of the VTQ value, the B-mode score, and the combined score were analyzed.
Results
The sensitivity, specificity, positive predictive value, negative predictive value, and accuracy of each scoring mode were: B-mode score, 84%, 85%, 66%, 94%, and 85%; VTQ, 75%, 91%, 73%, 92%, and 86%; and combined score, 88%, 87%, 70%, 95%, and 88%. The areas under the curves for B-mode, VTQ, and combined score were 0.895, 0.837, and 0.912, respectively. Pairwise comparisons of receiver-operating characteristic curves showed no statistical differences between B-mode and VTQ, and B-mode and combined score. Combined score showed better diagnostic performance than VTQ value (p = 0.0023).
It is widely accepted that malignant thyroid nodules are harder than benign nodules. This concept serves as the basis for a number of diagnostic examinations, including palpation. The principle underlying elastography is that tissue compression produces strain within the tissue that is less in hard tissue, as compared with soft tissue (1). Sonographic elastography is an imaging technique for visualizing tissue stiffness in color or grayscale. The application of sonographic elastography for thyroid nodule scoring reportedly has good diagnostic performance to predict the malignant thyroid nodule (234). According to previous studies, sonographic elastography is approved as a promising adjunctive tool for evaluation of thyroid nodules (567). Most initial studies used elasticity scoring systems (on 5-point or 6-point scales) to assign different scores according to the proportion of colored tissue on the map. However, elastographic evaluations with these elasticity scoring systems are qualitative in nature, highly operator- dependent, and have poor interobserver agreement and low reproducibility (58).
Acoustic radiation force impulse (ARFI) imaging is a new elastography method for quantifying tissue elasticity (9). Without any need for external compression, this elastometric technique evaluates tissue stiffness while performing real time B-mode examination. The tissue within a region of interest (ROI) is mechanically excited by short-duration acoustic pulses, which are generated by a probe. These pulses induce localized tissue displacement within the ROI and lateral shear-wave propagation. Multiple laterally positioned ultrasound tracking beams detect the shear wave and its velocity can be calculated (10111213). Shear wave propagation velocity is proportional to the square root of the tissue elasticity; thus, faster shear wave propagation is observed in stiffer tissue (91415). ARFI imaging can provide qualitative (grayscale map) or quantitative (wave velocity values, measured in m/s) information by Virtual Touch tissue imaging (VTI) and Virtual Touch tissue quantification (VTQ), respectively (915). Of these 2 methods, only VTQ can quantitatively describe thyroid nodule stiffness in terms of measured shear wave velocity (101112).
A few previous studies on ARFI for thyroid nodules reported consistently good performance of ARFI for differentiating malignant thyroid nodule from the benign (101112131617). However, the reported results varied from excellent value to just acceptable performance as an adjuvant tool.
The goal of this study was to evaluate the overall diagnostic performance of VTQ value for predicting malignant thyroid nodule, as compared with B-mode sonographic evaluation.
From November 2011 to April 2012, the ultrasonography (US) and clinical data of 146 thyroid nodules from 140 patients who were referred for US and fine needle aspiration biopsy (FNAB) were retrospectively reviewed. Seven nodules from 5 patients with unsatisfactory cytology results were excluded. Five additional nodules from 5 patients with cytologic results showing atypical cells of undetermined significance were also excluded; these patients were also lost to follow-up. Seven more patients with benign cytologic results and follow-up loss were also excluded. In total, 127 thyroid nodules (mean size: 16.4 mm, range: 5 mm to 49.6 mm) from 123 consecutive patients (105 females, 18 males) were included in the study. The exclusion criteria for this study were purely cystic or predominantly cystic thyroid nodules that did not require FNAB. This study was approved by the local Human Research Ethics Committee.
Thyroid nodules were considered as benign based on 2 consecutive cytologic FNAB biopsy results; moreover, nodules were required to show no interval change in size for at least 12 months after the US exam. Patients were diagnosed with malignant thyroid nodules based on pathologic diagnosis after surgery, following a suspicious malignant cytologic result in the fine-needle biopsy.
One radiologist who had 12 years of experience with conventional sonography and 3 years of experience in elastography, performed the US and the FNAB. Patients underwent both B-mode and ARFI imaging in the supine position with a sonography scanner (Acuson S2000, Siemens Medical Solutions, Mountain View, CA, USA). A linear array transducer (9L4, Siemens Medical Solutions) with a center frequency of 7.5 MHz (range, 5.0–14.0 MHz) was used for US examination. Patients were positioned on their backs with their necks slightly extended.
Conventional B-mode images of each thyroid nodule were initially obtained. Next, the ARFI imaging mode was turned on and the ROI was placed within the thyroid nodule to measure its VTQ value. While the VTQ value was being measured, patients were asked to hold their breath for a few seconds to minimize any potential artifacts from patient motion.
Next, a FNAB was performed on each nodule that had been evaluated by B-mode scanning and ARFI imaging. The FNAB was performed using a 23-gauge needle attached to a 10 mL disposable plastic syringe, and the aspirated material was expelled onto glass slides and smeared. For Papanicolaou staining, all smeared glass slides were fixed with 95% alcohol.
The B-mode nodule images were retrospectively reviewed for shape, margin, echogenicity, and calcification. Nodule vascularity was not evaluated, because increased vascularity did not have good diagnostic performance for predicting malignancy in previous studies (1819). Additionally, the sonographic features of all thyroid nodules that underwent US-guided FNAB were recorded at the same time that FNAB was performed. In our study, only solid and predominantly solid nodules were included. Nodule shapes were classified as ovoid or round, irregular, and taller-than-wide (defined as a ratio of the transverse diameter to the anteroposterior diameter > 1). Nodule echogenicities were classified as hyperechoic (defined as more echogenic than the normal thyroid parenchyma), isoechoic (isoechoic compared to the normal thyroid gland), hypoechoic (hypoechoic compared with thyroid parenchymae), or marked hypoechoic (hypoechoic compared with the surrounding strap muscle). Nodule margins were classified as smooth, spiculated/microlobulated or ill-defined, whereas calcifications were classified as microcalcification (< 1 mm in size), macrocalcification (> 1 mm), or rim calcification. Among these findings, a taller-than-wide shape, marked hypoechogenicity, a spiculated margin, and micro/ macrocalcification were considered as suspicious malignant findings, based on the Korean Society of Thyroid Radiology guidelines (20). Each nodule was assigned a B-mode score, according to its sonographic findings, as follows: shape (ovoid or round, 1; taller-than-wide, 2), echogenicity (hyperechoic or isoechoic, 1; hypoechoic or marked hypoechoic, 2), margin (smooth or ill-defined, 1; speculated/microlobulated, 2), and calcification (absent or rim calcification, 1; macro/microcalcification, 2). The total score for each thyroid nodule was determined by calculating the sum of all the criteria (range, 4 to 8).
After B-mode evaluation, the ARFI imaging mode was activated and the ROI (fixed size, 5 × 6 mm) was defined in the nodule, taking care to avoid the cystic area and calcification of the nodule. Also, ROI was placed at the central portion of nodule at a depth of 1.0 to 2.0 cm. The VTQ value of each thyroid nodule was measured 5 times continuously and the average value of these 5 measurements was calculated. An additional 1 to 2 minutes were needed to measure the VTQ value. The VTQ value expresses the shear wave speed in solid materials as numeric values in meters per second (m/s). Previous studies have shown that the VTQ value can be expressed as X.XX m/s in ARFI systems in some cases. This can be explained by a variety of possibilities including operator movement, patient respiration, erroneous ROI positioning (i.e., at the cystic portion of the nodule), or calcification (1012). If the VTQ value exceeds the upper detection limit (8.4 m/s) of the ARFI system, it is also shown as X.XX m/s (10). Among the cases in this study, only 1 solid and hypoechoic nodule without calcification in a patient with medullary carcinoma yielded a VTQ value of X.XX m/s. In this case, the X.XX value was considered as the upper limit (8.4 m/s).
The combined score for each thyroid nodule was defined as the sum of the B-mode evaluation score and the VTQ value. For example, a thyroid nodule with a round shape, isoechogenicity, and a smooth margin without calcification was given a B-mode score of 4. If the ARFI VTQ value of this nodule was 2 m/s, the combined score of this nodule was 6.
All statistical analyses were carried out with MedCalc, version 13 (MedCalc Software, Mariakerke, Belgium). p values < 0.05 were considered as statistical significance. Qualitative data were compared with the chi-squared test. Receiver-operating characteristic (ROC) curve analyses were performed to evaluate the diagnostic performances of the B-mode score, the VTQ value, and the combined score in differentiating malignant from benign thyroid nodules. Cut-off values for each method of analysis were determined using Youden's index. The areas under the ROC curves (Az) were calculated and compared using the z test.
Thirty-two of the 127 total thyroid nodules were malignant. Among the malignant thyroid nodules, 30 nodules were diagnosed as papillary carcinoma, 1 was follicular carcinoma, and the other was medullary carcinoma. The 2 consecutive cytologic results of the other 95 thyroid nodules were benign, nodular hyperplasia.
The mean size of the examined nodules was 17.9 mm (longest diameter of nodule) for benign and 11.8 mm for malignant nodules. The mean depth of both benign and malignant nodules was 13 mm without difference in the 2 groups. All nodules were evaluated according to the presence of suspicious malignant findings. The B-mode scores exhibited the following accuracies: 87%, shape; 76%, margin; 59%, echogenicity; and 65%, calcification. The Az for B-mode scoring was 0.895 [95% confidence interval (CI): 0.829–0.943] (p < 0.0001) with a cut-off value of 6.
The VTQ values of the nodules were distributed from 0.97 m/s to 8.4 m/s and the mean value of 2.78 m/s. The Az for ARFI evaluation and VTQ value was 0.837 (95% CI: 0.761–0.897) (p < 0.0001) with a cut-off value of 3.28 m/s. Twenty-three of the 32 malignant nodules showed a high VTQ value (> 3.28 m/s) (Fig. 1), whereas 83 of the 95 benign nodules exhibited a low VTQ value (≤ 3.28 m/s) (Fig. 2).
The mean combined score for the nodules was 8.53 (range, 4.97 to 16.4). The Az for the combined score was 0.912 (95% CI: 0.849–0.955) (p < 0.0001) with a cut-off value of 9.37. Twenty-seven nodules (84%) of all the malignant nodules showed a high combined score (> 9.37) and 85 (89%) of the benign nodules exhibited a low (< 9.37) combination score.
The diagnostic performances of the B-mode scoring, VTQ, and combination scoring approaches were shown in Table 1. B-mode scoring showed a sensitivity of 84%, a specificity of 85%, a positive predictive value (PPV) of 66%, a negative predictive value (NPV) of 94%, and an accuracy of 85%. Among the assessed categories, shape, echogenicity, and margin were significantly able to differentiate benign from malignant thyroid nodules. However, calcification did not show statistically meaningful results (p = 0.1911). ARFI evaluation/VTQ assessment showed a sensitivity of 75%, a specificity of 91%, a PPV of 73%, an NPV of 92%, and an accuracy of 86% (p < 0.0001). The combination scoring approach showed a sensitivity of 88%, a specificity of 87%, a PPV of 70%, an NPV of 95%, and an accuracy of 88% (p <0.0001). The ROC curves for the B-mode scoring, VTQ assessment, and combined scoring approaches for the differentiation of benign from malignant thyroid nodules were shown in Fig. 3.The areas under the curves for B-mode scoring, VTQ scoring, and combined scoring were 0.895, 0.837, and 0.912, respectively. ROC curve pairwise comparison analyses did not reveal any significant differences between the B-mode scoring and VTQ assessment approaches. However, the combined scoring method showed significantly improved performance, as compared with VTQ evaluation alone (p value = 0.0023). These results, including the areas under the curves, the 95% CIs, and the cut-off values were shown in Table 2.
US is the first choice imaging modality for evaluating thyroid nodules. According to B-mode findings, thyroid nodules are typically classified as probably benign, indeterminate, or suspicious. B-mode US findings and nodule classification are extremely important, because they are often used to determine whether a FNAB is necessary. Thyroid nodules that show findings typical of suspicious malignant and indeterminate nodules usually undergo further cytological evaluation according to nodule size (21). Recently, elastography was introduced as a new US diagnostic method that is useful for differentiating between malignant and benign thyroid nodules. Early studies on sonographic elastography used an elasticity scoring system to evaluate the thyroid nodules. US elastography coupled with an elasticity scoring system is reported to have good diagnostic value for the differential diagnosis of thyroid cancer (342223). Moreover, a real-time elasticity scoring system based on US elastography was reported to have exceptionally high diagnostic performance, with a sensitivity of 97%, a specificity of 100%, a PPV of 100%, and a NPV of 98% (4). However, recent studies of real-time elastography have shown somewhat lower diagnostic performance, as compared with previous studies. For instance, one study of the diagnostic performance of real-time elastography reported a sensitivity of 65%, a specificity of 72%, and an accuracy of 61% (5). Moreover, no statistically reliable interobserver agreement is reported in the application of a real-time elasticity scoring system to the diagnosis of malignant thyroid nodules (24). These contradictory results may be due to different operator technical skills and the qualitative nature of the elasticity scoring system, which can lead to low reproducibility.
ARFI is a recently developed US-based elastography modality that can provide quantitative information regarding tissue stiffness (925). Compared with conventional elastography, ARFI imaging is expected to produce operator-independent, highly reproducible, and quantitative results (1317). Various studies have investigated the diagnostic potential of ARFI imaging to differentiate malignant thyroid nodules (101213161726). Some studies showed that ARFI imaging exhibits excellent diagnostic performance in the differentiation of thyroid nodules. In one study, ARFI imaging was reported to have a robust diagnostic performance in predicting malignancy, with a sensitivity of 96.8%, a specificity of 95.7%, a PPV of 93.75%, an NPV 97.8%, and an Az of 0.989 (17). Another study used a 2.85 m/s cutoff value and found that the Az of the ROC curve was 0.9453 in the prediction of malignancy (sensitivity: 94.4%, specificity: 85.3%, PPV: 77.2%, NPV: 96.6%) (16). A following study that used ARFI imaging to evaluate 98 thyroid nodules also found good diagnostic values; the sensitivity, specificity, PPV, and NPV in this study were 86.36%, 93.42%, 79.17%, and 95.95%, respectively (12). Recently, some studies showed somewhat lower diagnostic performances of ARFI imaging for thyroid nodules. One study of ARFI imaging reported a sensitivity, specificity, NPV, and PPV of 57%, 85%, 93%, and 38%, respectively, with a 2.57 m/s cut-off value (10). Another study on the diagnostic value of ARFI imaging reported a sensitivity, specificity, PPV, and NPV of 75%, 82.2%, 58.9%, and 90.5%, respectively; the Az in this study was 0.861 (95% CI: 0.804–0.918; p < 0.001) (13).
The results of our study (sensitivity, 75%; specificity, 91%; PPV, 73%; and NPV, 92%) were similar to the diagnostic values in more recent studies that reported lower diagnostic performances for ARFI imaging of thyroid nodules. We compared ARFI imaging with B-mode evaluation and a combined method because this comparison most accurately reflects the clinical situation. The B-mode scoring approach showed a higher sensitivity (84%), specificity (85%), and NPV (94%), as compared with the ARFI evaluation. The ARFI evaluation of VTQ values showed a lower sensitivity (75%) and NPV (91.5%), as compared with the B-mode evaluation; however, the ARFI approach showed a higher specificity (90.5%) and PPV (72.7%). The B-mode scoring approach showed a higher Az value (0.895, p < 0.0001), as compared with ARFI evaluation (0.837, p < 0.0001); however, pairwise ROC curve comparison of both methods did not reveal any significant differences between them. These results indicated that both B-mode and ARFI evaluations have good diagnostic performance, and that the current ARFI evaluation technique is not a superior substitute for conventional B-mode imaging of thyroid nodules. Interestingly, the combined evaluation approach showed the highest sensitivity (88%), specificity (87%), PPV (70%), and NPV (95%). Moreover, the combined scoring approach showed the highest Az value (0.912), which was significantly greater than that of ARFI evaluation alone (p = 0.0023). These results indicated that a combined evaluation that incorporates both B-mode findings and ARFI evaluation could be useful.
Our study had a few limitations. First, nodules identified as benign by FNAB that had not been surgically removed were included in the study. This could have increased the false negative results of benign nodules. We only included cases that were confirmed as benign with > 2 consecutive FNAB results, to minimize this effect; moreover, the nodules were required to have no interval change in size or sonographic configuration. Second, the fixed size and shape of the ROI of ARFI measurement could not represent the entire area of the nodule. Therefore, we performed multiple ARFI measurements by changing the location of ROI within possible range. Another limitation of our study was that only a few types of malignant nodules were included. Among the 32 malignant nodules, 30 were papillary carcinoma nodules, with only 1 follicular carcinoma and 1 medullary carcinoma nodule included. Additional studies of thyroid nodules with more variable malignant pathologies are needed to fully validate our findings. The sample size of this study was small and further prospective studies with large case series are required.
In a few cases, we found that the VTQ results conflicted with the pathologic results. For example, one case of follicular carcinoma showed a low VTQ value (average VTQ value, 1.91) in the ARFI evaluation indicative of a false negative (Fig. 4). Thus, this follicular carcinoma case could not be discriminated from a benign nodule in our study. The differentiation of follicular neoplasms from nodular hyperplasia remains a diagnostic challenge. In contrast, another benign nodule showed a high VTQ value (average VTQ value, 3.86) indicative of a false positive (Fig. 5). This benign nodule showed calcific degeneration, which may have led to a high VTQ value similar to that of a malignant nodule. From these results, we concluded that the ARFI VTQ value alone is not a reliable alternative diagnostic method to B-mode US findings. However, a high VTQ value could be considered as a suspicious malignant finding that further requires FNAB.
In conclusion, ARFI evaluation of the VTQ value is a good diagnostic modality for differentiating malignant thyroid nodules from benign nodules. However, ARFI evaluation is not superior to B-mode sonographic evaluation, but has a better diagnostic performance when combined with B-mode sonographic finding scores.
Figures and Tables
Fig. 1
Solid, marked hypoechoic nodule with calcification shows high VTQ score (4.22 m/s). This nodule was confirmed as papillary thyroid carcinoma.
VTQ = Virtual Touch tissue quantification
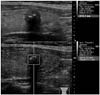
Fig. 2
Predominantly solid, isoechoic nodule shows low VTQ score (1.20 m/s) in ARFI evaluation. This nodule was confirmed as benign nodular hyperplasia.
ARFI = acoustic radiation force impulse, VTQ = Virtual Touch tissue quantification
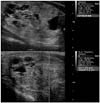
Fig. 3
Receiver operating characteristic curves for B-mode evaluation, VTQ value and combined evaluation. Area under the curve for combined evaluation (0.912) is higher than that for B-mode (0.895) or VTQ value (0.837).
VTQ = Virtual Touch tissue quantification
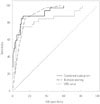
Fig. 4
Solid, heterogeneous isoechoic nodule shows relatively low VTQ value (2.03 m/s). This nodule was confirmed as follicular carcinoma.
VTQ = Virtual Touch tissue quantification
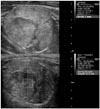
Fig. 5
Hypoechoic solid nodule with macrocalcification shows high VTQ value (4.71 m/s) in ARFI evaluation. This nodule was confirmed as benign nodule with calcific degeneration.
ARFI = acoustic radiation force impulse, VTQ = Virtual Touch tissue quantification
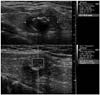
Table 1
Results of Sonography Criteria for Benign and Malignant Thyroid Nodules
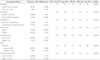
Table 2
Comparison of Receiver-Operating Characteristic (ROC) Curves

References
1. Itoh A, Ueno E, Tohno E, Kamma H, Takahashi H, Shiina T, et al. Breast disease: clinical application of US elastography for diagnosis. Radiology. 2006; 239:341–350.
2. Dighe M, Bae U, Richardson ML, Dubinsky TJ, Minoshima S, Kim Y. Differential diagnosis of thyroid nodules with US elastography using carotid artery pulsation. Radiology. 2008; 248:662–669.
3. Hong Y, Liu X, Li Z, Zhang X, Chen M, Luo Z. Real-time ultrasound elastography in the differential diagnosis of benign and malignant thyroid nodules. J Ultrasound Med. 2009; 28:861–867.
4. Rago T, Santini F, Scutari M, Pinchera A, Vitti P. Elastography: new developments in ultrasound for predicting malignancy in thyroid nodules. J Clin Endocrinol Metab. 2007; 92:2917–2922.
5. Moon HJ, Sung JM, Kim EK, Yoon JH, Youk JH, Kwak JY. Diagnostic performance of gray-scale US and elastography in solid thyroid nodules. Radiology. 2012; 262:1002–1013.
6. Kwak JY, Kim EK. Ultrasound elastography for thyroid nodules: recent advances. Ultrasonography. 2014; 33:75–82.
7. Sun J, Cai J, Wang X. Real-time ultrasound elastography for differentiation of benign and malignant thyroid nodules: a meta-analysis. J Ultrasound Med. 2014; 33:495–502.
8. Bojunga J, Herrmann E, Meyer G, Weber S, Zeuzem S, Friedrich-Rust M. Real-time elastography for the differentiation of benign and malignant thyroid nodules: a meta-analysis. Thyroid. 2010; 20:1145–1150.
9. Zhai L, Palmeri ML, Bouchard RR, Nightingale RW, Nightingale KR. An integrated indenter-ARFI imaging system for tissue stiffness quantification. Ultrason Imaging. 2008; 30:95–111.
10. Bojunga J, Dauth N, Berner C, Meyer G, Holzer K, Voelkl L, et al. Acoustic radiation force impulse imaging for differentiation of thyroid nodules. PLoS One. 2012; 7:e42735.
11. Friedrich-Rust M, Romenski O, Meyer G, Dauth N, Holzer K, Grünwald F, et al. Acoustic Radiation Force Impulse-Imaging for the evaluation of the thyroid gland: a limited patient feasibility study. Ultrasonics. 2012; 52:69–74.
12. Gu J, Du L, Bai M, Chen H, Jia X, Zhao J, et al. Preliminary study on the diagnostic value of acoustic radiation force impulse technology for differentiating between benign and malignant thyroid nodules. J Ultrasound Med. 2012; 31:763–771.
13. Zhang YF, Xu HX, He Y, Liu C, Guo LH, Liu LN, et al. Virtual touch tissue quantification of acoustic radiation force impulse: a new ultrasound elastic imaging in the diagnosis of thyroid nodules. PLoS One. 2012; 7:e49094.
14. Nightingale K, Soo MS, Nightingale R, Trahey G. Acoustic radiation force impulse imaging: in vivo demonstration of clinical feasibility. Ultrasound Med Biol. 2002; 28:227–235.
15. Palmeri ML, Wang MH, Dahl JJ, Frinkley KD, Nightingale KR. Quantifying hepatic shear modulus in vivo using acoustic radiation force. Ultrasound Med Biol. 2008; 34:546–558.
16. Zhan J, Diao XH, Chai QL, Chen Y. Comparative study of acoustic radiation force impulse imaging with real-time elastography in differential diagnosis of thyroid nodules. Ultrasound Med Biol. 2013; 39:2217–2225.
17. Zhang FJ, Han RL. The value of acoustic radiation force impulse (ARFI) in the differential diagnosis of thyroid nodules. Eur J Radiol. 2013; 82:e686–e690.
18. Kim HG, Moon HJ, Kwak JY, Kim EK. Diagnostic accuracy of the ultrasonographic features for subcentimeter thyroid nodules suggested by the revised American Thyroid Association guidelines. Thyroid. 2013; 23:1583–1589.
19. Moon HJ, Kwak JY, Kim MJ, Son EJ, Kim EK. Can vascularity at power Doppler US help predict thyroid malignancy. Radiology. 2010; 255:260–269.
20. Moon WJ, Baek JH, Jung SL, Kim DW, Kim EK, Kim JY, et al. Ultrasonography and the ultrasound-based management of thyroid nodules: consensus statement and recommendations. Korean J Radiol. 2011; 12:1–14.
21. American Thyroid Association (ATA) Guidelines Taskforce on Thyroid Nodules and Differentiated Thyroid Cancer. Cooper DS, Doherty GM, Haugen BR, Kloos RT, Lee SL. Revised American Thyroid Association management guidelines for patients with thyroid nodules and differentiated thyroid cancer. Thyroid. 2009; 19:1167–1214.
22. Asteria C, Giovanardi A, Pizzocaro A, Cozzaglio L, MorabitoA , Somalvico F, et al. US-elastography in the differential diagnosis of benign and malignant thyroid nodules. Thyroid. 2008; 18:523–531.
23. Lyshchik A, Higashi T, Asato R, Tanaka S, Ito J, Mai JJ, et al. Thyroid gland tumor diagnosis at US elastography. Radiology. 2005; 237:202–211.
24. Park SH, Kim SJ, Kim EK, Kim MJ, Son EJ, Kwak JY. Interobserver agreement in assessing the sonographic and elastographic features of malignant thyroid nodules. AJR Am J Roentgenol. 2009; 193:W416–W423.
25. Nightingale K, Bentley R, Trahey G. Observations of tissue response to acoustic radiation force: opportunities for imaging. Ultrason Imaging. 2002; 24:129–138.
26. Hou XJ, Sun AX, Zhou XL, Ji Q, Wang HB, Wei H, et al. The application of Virtual Touch tissue quantification (VTQ) in diagnosis of thyroid lesions: a preliminary study. Eur J Radiol. 2013; 82:797–801.