This article has been corrected. See "Erratum: Coronary Stent on Coronary CT Angiography: Assessment with Model-Based Iterative Reconstruction Technique" in Volume 74 on page 417.
Abstract
Purpose
To assess the performance of model-based iterative reconstruction (MBIR) technique for evaluation of coronary artery stents on coronary CT angiography (CCTA).
Materials and Methods
Twenty-two patients with coronary stent implantation who underwent CCTA were retrospectively enrolled for comparison of image quality between filtered back projection (FBP), adaptive statistical iterative reconstruction (ASIR) and MBIR. In each data set, image noise was measured as the standard deviation of the measured attenuation units within circular regions of interest in the ascending aorta (AA) and left main coronary artery (LM). To objectively assess the noise and blooming artifacts in coronary stent, we additionally measured the standard deviation of the measured attenuation and intra-luminal stent diameters of total 35 stents with dedicated software.
Results
All image noise measured in the AA (all p < 0.001), LM (p < 0.001, p = 0.001) and coronary stent (all p < 0.001) were significantly lower with MBIR in comparison to those with FBP or ASIR. Intraluminal stent diameter was significantly higher with MBIR, as compared with ASIR or FBP (p < 0.001, p = 0.001).
A sizable segment of the population will undergo coronary artery stent implantation in their lifetime. They require frequent follow-up for stent patency; hence, noninvasive, low radiation dose imaging technique to rule out in-stent re-stenosis is desirable (1). Coronary CT angiography (CCTA) has been used for this purpose, and the evaluation of larger (≥ 3 mm) coronary artery stents is considered appropriate according to current guidelines (1234567). Two recent meta-analyses demonstrate the high diagnostic value of CCTA with sensitivities and specificities > 90% when non-evaluable stents are excluded (189). However, these studies also confirm CCTA's long recognized limitations of bl-ooming artifact, which can cause a decrease in diagnostic image quality or even render studies non-diagnostic (14). Therefore, it is important to reduce both noise and blooming artifact for a better quality CCTA image, in the evaluation of coronary artery stents.
Improved computing power has led to the introduction of iterative reconstruction into the clinical arena, and its beneficial effects throughout the body are reported (1). Improved image quality, reduction in image noise, and the potential for radiation dose savings are demonstrated, as compared with traditional filtered back projection (FBP) (1101112). Several studies showed that iterative reconstruction techniques could reduce image noise and blooming artifacts when heavy calcifications or stent are present in coronary arteries (113). Adaptive statistical iterative reconstruction (ASIR) techniques are reported to significantly improve the imaging evaluation of coronary artery stents and have the potential to substantially reduce the radiation dose (114).
Recently developed model-based iterative reconstruction (MBIR) technique is a raw data based 3-dimensional image reconstruction method (15). It does not involve blending with FBP images, and is mathematically more complex and accurate than ASIR techniques (16). Several studies showed that MBIR images, as compared with ASIR and FBP images, have equivalent or better image quality with a substantial decrease in image noise and potential to reduce radiation dose on CT images of cardiac, liver, lung and cervicothoracic region (15161718).
However, to the best of our knowledge, there is no data regarding the potential usefulness of MBIR for evaluation of coronary artery stent. Therefore, the purpose of this study was to assess the performance of MBIR technique for evaluation of coronary artery stent using CCTA in comparison to ASIR and the traditional FBP algorithm.
The study was approved by our Institutional Review Board that waived the need for individual informed patient consent. The study population consisted of consecutive 33 patients of post-percutaneous coronary intervention status with stent implantation who underwent CCTA within 12 months for risk assessment due to prior positive ischemic equivalents (1, 2, 3). Exclusion criteria were images with poor quality due to motion artifact, small caliber of vessel diameter less than 2.5 mm, in-stent restenosis on previous follow-up conventional angiography, and insufficient medical records. After exclusion of 11 patients, CT images of 22 patients (13 men, 9 women; mean age 66.27 ± 10.16 years, 35 coronary stents) were analyzed (Tables 1, 2).
CCTA were performed using a 256-slice MDCT scanner (Brilliance iCT; Philips Medical Systems, Best, the Netherlands). The parameters were detector configurations: 256 overlapping slices of 0.625-mm thickness and a dynamic z-focal spot; gantry rotation time, 0.27 seconds; tube voltage, 120 kVp; and 800–1050 mAs (depending on patient habitus). Prior to CCTA, all patients with a baseline heart rate over 70 beats per minute (bpm) received intravenous esmolol (10–30 mg; Jeil Pharm, Seoul, Korea). Nitroglycerine (0.6 mg) was immediately administered sublingually to all patients before contrast injection, with the exception of patients with a contraindication to nitroglycerin (19, 20). A bolus of 70 mL of iomeprol (Iomeron 400; Bracco, Milan, Italy) was injected intravenously (4 mL/s), followed by a 50-mL saline chaser. A region of interest (ROI) was placed in the descending thoracic aorta, and image acquisition was automatically initiated 7 seconds after a selected threshold [150 Hounsfield units (HU)] had been reached with bolus tracking. Details of data acquisition are described previously (19). Patients with heart rates < 70 bpm underwent CCTA using a prospectively-triggered axial technique, and patients with heart rates > 70 bpm underwent a retrospectively-gated helical technique. Details of MBIR reconstruction are described previously (17). After a pilot study with 15 cases, soft-tissue kernel was selected as the optimal type to reconstruct the CT images.
All images were reviewed and interpreted on dedicated workstation by two radiologists. In each data set, one observer measured image noise, which was defined as the standard deviation (SD) of the measured attenuation (in HU) within circular ROIs in the ascending aorta and left main coronary artery (LM). The size of the ROIs was adapted to account for anatomic differences of our patients, representing an area that was not too small as to be affected by pixel variability and not so large as to approach the edges of the vessel. However, between the different reconstruction approaches, the ROI size was kept constant within each patient. We compared these parameters between the three reconstruction settings.
We also measured the stent lumen diameter in portions free of stenosis on curved multi-planar images acquired with the FBP, ASIR, and MBIR algorithm, in order to objectively assess the reduction of blooming artifact in coronary stent by each reconstruction method. Using dedicated software, in-stent lumen maximal diameter was calculated automatically. The window width and level was set at 1500 and 300 HU, respectively.
Statistical analysis was performed using dedicated statistical software (SPSS 12.0, SPSS Inc., Chicago, IL, USA). Noise and in-stent diameters were expressed as mean ± SD and compared using paired t-test and repeated measure ANOVA. A p-value of < 0.05 was considered statistically significant. Interobserver agreement was determined by intra-class correlation coefficient.
Patient demographics and scan parameters on CCTA were provided in Table 1. Image noise with MBIR in the ascending aorta was significantly lower, as compared to those with FBP and ASIR (all p < 0.001; 34.07 ± 8.67 HU with FBP, 21.15 ± 4.16 HU with ASIR, and 10.59 ± 10.59 HU with MBIR) (Fig. 1). Interobserver agreements were between 0.616 and 0.896.
Image noise with MBIR in the LM was significantly lower, as compared to those with FBP and ASIR (p < 0.001, p = 0.001). The mean values of image noise in each data set were 55.32 ± 30.60 HU with FBP, 41.71 ± 24.04 HU with ASIR, and 34.80 ± 23.93 HU with MBIR (Fig. 2). Interobserver agreements were between 0.958 and 0.977.
Image noise with MBIR within coronary stent was significantly lower, as compared to those with FBP and ASIR (all p < 0.001) (Fig. 3). The mean values of image noise were 39.39 ± 17.46 HU with FBP, 29.53 ± 13.82 HU with ASIR, and 19.96 ± 12.19 HU with MBIR (Fig. 4). Interobserver agreements were between 0.720 and 0.907.
In-stent diameters with MBIR showed significantly higher value, as compared to those with ASIR and FBP (p < 0.001, p = 0.001), which indicated that the reduction of blooming artifact was best on images with MBIR than those with ASIR and FBP (Fig. 3). The mean values of intraluminal stent diameter were 1.67 ± 0.45 with FBP, 1.75 ± 0.43 with ASIR, and 1.80 ± 0.43 with MBIR (Fig. 5). Interobserver agreements were between 0.990 and 0.994.
The main findings of the present study were as follows: 1) All image noise was significantly lower with MBIR in comparison to those with FBP or ASIR. 2) Intraluminal stent diameter was significantly higher with MBIR, as compared with FBP or ASIR. Our study showed that the application of MBIR in patients with coronary stent could improve the image quality, as compared to FBP or ASIR, in terms of reduced noise and blooming artifact.
Results reported from previous studies likewise indicated that MBIR could provide improved image quality in cardiovascular imaging. Scheffel et al. (15) reported that MBIR can provide reduced image noise and increased contrast-to-noise ratio compared to those using ASIR and FBP in CCTA of ex vivo human heart (15). Oda et al. (21) also reported improved image quality in low-dose cardiac CT with MBIR (20% of the standard tube current) than those using full dose or low dose FBP. Besides, other studies showed similar or improved image quality and equivalent lesion detection in abdominal (17, 22) and chest (18) CT using MBIR, as compared with FBP or ASIR.
Interestingly, although Scheffel et al. (15) were unable to show the effective suppression of blooming artifact in the evaluation of calcified plaque with MBIR than other reconstruction methods, our study showed significant reduction of blooming artifact in the evaluation of coronary stent. This discrepancy is possibly due to different methods of analysis; while Scheffel et al. (15) only used qualitative method with four-point scale to qualify blooming artifact, we adopted quantitative method by measuring the intraluminal stent diameters using dedicated software. Moreover, other studies reported that ASIR technique provides improved in-stent lumen visualization by reducing noise or blooming artifact in the evaluation of coronary stent using CCTA at a lower radiation dose. However, they are either studies with phantom only (14) or those that do not include MBIR (1, 14).This study is meaningful, since it is the first to evaluate in-stent image quality in CCTA with MBIR.
From a clinical viewpoint, the expected increased accuracy and reliability in diagnosing in-stent stenosis could be extended to clinical applicability in follow-up-patients with implanted coronary stent. Also, improvement of image quality with MBIR suggests the potential to reduce radiation dose while preserving similar image quality level, as compared to those of FBP or other iterative reconstructions.
There are several limitations to be considered in our study. First, we did not provide the diagnostic accuracy of MBIR in the evaluation of in-stent restenosis using CCTA; hence, further study will be needed. Second, due to the limited size of the study population, we did not conduct subgroup analysis according to the diameter of coronary stent, which could possibly have biased the results. Third, despite improved image quality and in-stent lumen visualization, we did not assess the diagnostic accuracy of disease such as in-stent restenosis in coronary stent on CCTA using MBIR. Fourth, potential radiation dose reduction using MBIR can only be assumed and is without definite evidence, because the image quality of MBIR was compared with other reconstruction methods at the same radiation dose settings. Further studies are needed to compare the image quality of MBIR in reduced radiation dose setting, with those of other reconstruction techniques in conventional radiation dose settings. Fifth, our study did not provide intraobserver agreement. We considered that calculation of intraobserver agreement would be unhelpful, because the observers measured noise and diameter using dedicated software. Sixth, we did not include signal to noise ratio and contrast to noise ratio in this study.
In summary, the results of our study suggested that MBIR could provide significant improvement of image quality, as compared to FPB or ASIR by reducing noise and blooming artifact in the evaluation of coronary stent on CCTA. MBIR has the potential to enhance diagnostic accuracy of in-stent stenosis or reduce the radiation dose in the evaluation of coronary stent.
Figures and Tables
Fig. 1
Image noise measured in the ascending aorta is significantly lower by MBIR, as compared to ASIR and FBP (all p < 0.001).
AA = ascending aorta, ASIR = adaptive statistical iterative reconstruction, FBP = filtered back projection, HU = Hounsfield units, MBIR = model-based iterative reconstruction
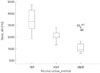
Fig. 2
Image noise measured in the left main coronary artery is significantly lower by MBIR, as compared to ASIR and FBP (p < 0.001, p = 0.001).
ASIR = adaptive statistical iterative reconstruction, FBP = filtered back projection, HU = Hounsfield units, LM = left main coronary artery, MBIR = model-based iterative reconstruction
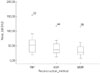
Fig. 3
Straight view of curved MPR images with FBP (A), ASIR (B), and MBIR (C) technique. Curved MPR images with FBP (D), ASIR (E), and MBIR (F) technique. Note the reduction of noise and blooming artifact in coronary stent is better on images with MBIR (C, F) than those with ASIR (B, E) and FBP (A, D).
ASIR = adaptive statistical iterative reconstruction, FBP = filtered back projection, MBIR = model-based iterative reconstruction, MPR = multi-planar reconstruction
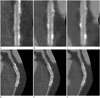
Fig. 4
Image noise measured in the stent is significantly lower by MBIR, as compared to ASIR and FBP (all p < 0.001).
ASIR = adaptive statistical iterative reconstruction, FBP = filtered back projection, HU = Hounsfield units, MBIR = model-based iterative reconstruction
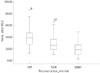
Fig. 5
In-stent diameters are significantly higher by MBIR, as compared to ASIR and FBP, which means that the reduction of blooming artifact is better by MBIR (p < 0.001, p = 0.001) than ASIR and FBP.
ASIR = adaptive statistical iterative reconstruction, FBP = filtered back projection, HU = Hounsfield units, MBIR = model-based iterative reconstruction
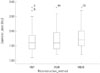
Table 1
Patient Demographics and CCTA Scan Parameters
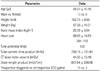
Table 2
Types of Stents in the Study Population
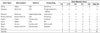
Acknowledgments
This work was supported by the National Research Foundation of Korea (NRF) grant funded by the Korea government (MEST) (No. 2011-0023624).
References
1. Ebersberger U, Tricarico F, Schoepf UJ, Blanke P, Spears JR, Rowe GW, et al. CT evaluation of coronary artery stents with iterative image reconstruction: improvements in image quality and potential for radiation dose reduction. Eur Radiol. 2013; 23:125–132.
2. Kim YJ, Yong HS, Kim SM, Kim JA, Yang DH, Hong YJ. Guideline for Appropriate Use of Cardiac CT in Heart Disease. J Korean Soc Radiol. 2014; 70:93–109.
3. Taylor AJ, Cerqueira M, Hodgson JM, Mark D, Min J, O'Gara P, et al. ACCF/SCCT/ACR/AHA/ASE/ASNC/NASCI/SCAI/SCMR 2010 appropriate use criteria for cardiac computed tomography. A report of the American College of Cardiology Foundation Appropriate Use Criteria Task Force, the Society of Cardiovascular Computed Tomography, the American College of Radiology, the American Heart Association, the American Society of Echocardiography, the American Society of Nuclear Cardiology, the North American Society for Cardiovascular Imaging, the Society for Cardiovascular Angiography and Interventions, and the Society for Cardiovascular Magnetic Resonance. J Am Coll Cardiol. 2010; 56:1864–1894.
4. Andreini D, Pontone G, Bartorelli AL, Mushtaq S, Trabattoni D, Bertella E, et al. High diagnostic accuracy of prospective ECG-gating 64-slice computed tomography coronary angiography for the detection of in-stent restenosis: in-stent restenosis assessment by low-dose MDCT. Eur Radiol. 2011; 21:1430–1438.
5. Kerl JM, Schoepf UJ, Vogl TJ, Ackermann H, Vogt S, Costello P, et al. In vitro evaluation of metallic coronary artery stents with 64-MDCT using an ECG-gated cardiac phantom: relationship between in-stent visualization, stent type, and heart rate. AJR Am J Roentgenol. 2010; 194:W256–W262.
6. Sheth T, Dodd JD, Hoffmann U, Abbara S, Finn A, Gold HK, et al. Coronary stent assessability by 64 slice multi-detector computed tomography. Catheter Cardiovasc Interv. 2007; 69:933–938.
7. Zhang X, Yang L, Ju H, Zhang F, Wu J, He B, et al. Prevalence and prognosis of coronary stent gap detected by multi-detector CT: a follow-up study. Eur Radiol. 2012; 22:1896–1903.
8. Sun Z, Almutairi AM. Diagnostic accuracy of 64 multislice CT angiography in the assessment of coronary in-stent restenosis: a meta-analysis. Eur J Radiol. 2010; 73:266–273.
9. Kumbhani DJ, Ingelmo CP, Schoenhagen P, Curtin RJ, Flamm SD, Desai MY. Meta-analysis of diagnostic efficacy of 64-slice computed tomography in the evaluation of coronary in-stent restenosis. Am J Cardiol. 2009; 103:1675–1681.
10. Gosling O, Loader R, Venables P, Roobottom C, Rowles N, Bellenger N, et al. A comparison of radiation doses between state-of-the-art multislice CT coronary angiography with iterative reconstruction, multislice CT coronary angiography with standard filtered back-projection and invasive diagnostic coronary angiography. Heart. 2010; 96:922–926.
11. Prakash P, Kalra MK, Ackman JB, Digumarthy SR, Hsieh J, Do S, et al. Diffuse lung disease: CT of the chest with adaptive statistical iterative reconstruction technique. Radiology. 2010; 256:261–269.
12. Sagara Y, Hara AK, Pavlicek W, Silva AC, Paden RG, Wu Q. Abdominal CT: comparison of low-dose CT with adaptive statistical iterative reconstruction and routine-dose CT with filtered back projection in 53 patients. AJR Am J Roentgenol. 2010; 195:713–719.
13. Renker M, Nance JW Jr, Schoepf UJ, O'Brien TX, Zwerner PL, Meyer M, et al. Evaluation of heavily calcified vessels with coronary CT angiography: comparison of iterative and filtered back projection image reconstruction. Radiology. 2011; 260:390–399.
14. Funama Y, Oda S, Utsunomiya D, Taguchi K, Shimonobo T, Yamashita Y, et al. Coronary artery stent evaluation by combining iterative reconstruction and high-resolution kernel at coronary CT angiography. Acad Radiol. 2012; 19:1324–1331.
15. Scheffel H, Stolzmann P, Schlett CL, Engel LC, Major GP, Károlyi M, et al. Coronary artery plaques: cardiac CT with model-based and adaptive-statistical iterative reconstruction technique. Eur J Radiol. 2012; 81:e363–e369.
16. Katsura M, Sato J, Akahane M, Matsuda I, Ishida M, Yasaka K, et al. Comparison of pure and hybrid iterative reconstruction techniques with conventional filtered back projection: image quality assessment in the cervicothoracic region. Eur J Radiol. 2013; 82:356–360.
17. Chang W, Lee JM, Lee K, Yoon JH, Yu MH, Han JK, et al. Assessment of a model-based, iterative reconstruction algorithm (MBIR) regarding image quality and dose reduction in liver computed tomography. Invest Radiol. 2013; 48:598–606.
18. Kim H, Park CM, Song YS, Lee SM, Goo JM. Influence of radiation dose and iterative reconstruction algorithms for measurement accuracy and reproducibility of pulmonary nodule volumetry: a phantom study. Eur J Radiol. 2014; 83:848–857.
19. Lee MS, Chun EJ, Kim KJ, Kim JA, Vembar M, Choi SI. Reproducibility in the assessment of noncalcified coronary plaque with 256-slice multi-detector CT and automated plaque analysis software. Int J Cardiovasc Imaging. 2010; 26:Suppl 2. 237–244.
20. Chun EJ, Lee W, Choi YH, Koo BK, Choi SI, Jae HJ, et al. Effects of nitroglycerin on the diagnostic accuracy of electrocardiogram-gated coronary computed tomography angiography. J Comput Assist Tomogr. 2008; 32:86–92.
21. Oda S, Utsunomiya D, Funama Y, Katahira K, Honda K, Tokuyasu S, et al. A knowledge-based iterative model reconstruction algorithm: can super-low-dose cardiac CT be applicable in clinical settings? Acad Radiol. 2014; 21:104–110.
22. Shuman WP, Green DE, Busey JM, Kolokythas O, Mitsumori LM, Koprowicz KM, et al. Model-based iterative reconstruction versus adaptive statistical iterative reconstruction and filtered back projection in liver 64-MDCT: focal lesion detection, lesion conspicuity, and image noise. AJR Am J Roentgenol. 2013; 200:1071–1076.