Abstract
Purpose
To evaluate the effects of proteoglycan and type II collagen within articular cartilage on T1ρ relaxation time of articular cartilage.
Materials and Methods
This study was exempted by the institutional and animal review boards, and informed consent was not required. Twelve porcine patellae were assigned to three groups of control, trypsin-treated (proteoglycan-degraded), or collagenase-treated (collagen-degraded). The T1ρ images were obtained with a 3 tesla magnetic resonance imaging scanner with a single loop coil. Statistical differences were detected by analysis of variance to evaluate the effects of the enzyme on T1ρ relaxation time. Safranin-O was used to stain proteoglycan in the articular cartilage and immunohistochemical staining was performed for type II collagen.
Osteoarthritis, also known as degenerative arthritis, is a very common and significant disease characterized by decreases in proteoglycan (PG) and collagen fibers in the articular cartilage (1, 2). Although many cartilage regeneration treatment methods have been developed due to advances in molecular biology and stem cell research (2, 3), cartilage damage is irreversible, and the only treatment method for severely damaged articular cartilage is arthroplasty with an artificial joint. Thus, early detection of degeneration is important to enable early therapeutic intervention, reduced disability, and improve patient quality of life (1,2,3). Recent advances in magnetic resonance imaging (MRI) have made it possible to detect degenerative changes in articular cartilage, which are characterized by changes only in the biochemical component with minimal or no structural changes (4,5,6,7,8,9,10). Delayed gadolinium-enhanced magnetic resonance imaging of cartilage (dGEMRIC) is a method to quantify loss of PGs in articular cartilage (5). However, a double dose of intravenous contrast media is required for this type of imaging, and patients must wait 2 hours after the injection before analysis. T2 mapping is a MRI method used to measure T2 relaxation time, which is influenced by the content and orientation of collagen fibers in articular cartilage. Thus, T2 mapping visualizes changes in articular cartilage collagen fibers but has low sensitivity and lacks specificity to quantify PG loss (7, 9, 11, 12). T1ρ relaxation time is a parameter related to the energy exchange between water molecules and the surrounding environment by spin-lattice relaxation in a rotating frame. Unlike dGEMRIC and T2 mapping, T1ρ imaging does not require contrast media or a long scan time and has better sensitivity than that of T2 mapping. Thus, T1ρ imaging has been proposed as an attractive alternative method to probe biochemical changes in cartilage (7, 13). Several studies have demonstrated that T1ρ relaxation time reflects macromolecules, such as PGs and collagen, in the articular cartilage extracellular matrix (8, 9). Nishioka et al. (8) showed that T1ρ relaxation time varies with degenerative changes in human articular cartilage. Akella et al. (14) showed that T1ρ relaxation time is directly proportional with loss of PGs in articular cartilage. However, it remains unclear whether in vivo T1ρ relaxation time reflects the changes in PGs, collagen fibers, or both that result from cartilage degeneration (15, 16).
Thus, the purpose of this study was to evaluate the effects of PGs and type II collagen on T1ρ values of articular cartilage.
This study was exempted by the institutional and animal review boards, and informed consent was not required.
Twelve fresh porcine patellae from 1-2-year-old pigs were obtained from a local slaughter house within 6 hours after sacrifice. After dissecting the surrounding soft tissue, the patellae were sagittally hemisected with a mechanical cutter and stored frozen. The cartilage was thawed at room temperature for the investigation. We assigned the specimens to three groups as follows: 1) trypsin-treated (n = 4), 2) collagenase-treated (n = 4), and 3) control (n = 4).
The patellae were placed in a vial containing 1 mg trypsin (Sigma, St. Louis, MO, USA) in 1 mL phosphate buffered saline (PBS) for the trypsin-treated group, in a vial containing 135 units collagenase (Sigma) in 1 mL PBS for the collagenase-treated group, and in PBS without enzymes for the control group. We expected the trypsin and collagenase to degrade the PGs and collagen, respectively. All three groups were incubated for 6 hours at 37℃ with gentle agitation. Each patella was placed in a container half-filled with agarose gel. The flat surface of the hemisected patella was fixed to an agar plate using adhesive. Perfluorocarbon was used as the buffer, which filled the empty space in the container.
T1ρ imaging was obtained with a 3 tesla MRI scanner (Trio; Siemens Medical Solutions, Erlangen, Germany) with a single loop coil. The specimen was loaded into the coil ensuring that one of the articular surfaces was perpendicular to the B0 direction of the magnetic field. Coronal two-dimensional T1ρ-weighted images were obtained using the spin-lock technique and spiral image acquisition. The acquisition parameters were: field of view = 6 × 6 cm, matrix = 128 × 128, effective in-plane spatial resolution = 0.47 × 0.47 mm, slice thickness = 3 mm, repetition time/echo time = 3500/3.08 ms, number of excitation = 2, spin-lock time (TSL) = 0/10/30/50/80 ms, flip angle = 30°, and spin-lock frequency = 500 Hz. Acquisition time was 1 min 30 sec for each TSL.
T1ρ maps were reconstructed by fitting image intensity pixel-by-pixel to the equation below using the mono-exponential fitting algorithm: S (TSL) = S0 × exp (-TSL / T1ρ), where TSL is spin-lock time, and S is the signal intensity of the T1ρ-weighted image with a given TSL. The articular cartilage T1ρ map was constructed with a homemade mapping program using Mat Lab R2013 (MathWorks, Natick, MA, USA). After obtaining the T1ρ map result as a DICOM file, the T1ρ value of each patellar cartilage was measured using a Picture Archiving Communication System (M-view, Infinitt, Seoul, Korea). The mean T1ρ value of the patellar cartilage was evaluated by placing the region of interest in the patellar cartilage using a freehand drawing technique (Fig. 1B). The program also provided a T1ρ map as a color mapping file (Fig. 1A).
All patellae were sagittally sawed with a 3-4 mm thickness thin plate using a mechanical cutter immediately after MRI scanning. The thin plates of cartilage were fixed in neutral buffered formalin at room temperature. After decalcification and paraffin embedding, the cartilage plates were cut into tiny fragments. The plates were stained with Safranin-O for PGs, and immunohistochemical staining was used to detect type II collagen. Safranin-O staining was performed with a 0.1% Safranin-O solution, and with a 0.2% aqueous solution of Fast Green used as a counter-stain. The tissue sections for type II collagen immunohistochemistry were deparaffinized in xylene and rehydrated in a reverse-graded ethanol series. The sections were immunohistochemically stained with rabbit polyclonal antibodies against rabbit type II collagen (Abcam, Cambridge, UK) and goat anti-rabbit IgG/biotin (Abcam) using the manufacturer's standard protocol (17).
Mean T1ρ values of the control, trypsin-treated, and collagenase-treated groups were 37.72 ± 5.82, 57.53 ± 8.24, and 45.08 ± 5.31 msec, respectively (p < 0.001). The mean T1ρ value of the trypsin-treated group was significantly higher than that of the control and collagenase-treated groups (p < 0.001). The difference in the T1ρ values between the control and the collagenase-treated groups was small, but significant (p = 0.008). Table 1 summarizes the T1ρ values for each group.
Loss of PGs was observed in the trypsin-degraded group and was confirmed by microscopy in the Safranin-O stained specimens. The histological samples of normal articular cartilage showed a dense and homogeneous distribution of PGs (Fig. 2A), whereas those of Safranin-O stained trypsin-degraded articular cartilage showed observable decreases in PGs (Fig. 2B). The color-mapped T1ρ data, wherein a color scale represents the T1ρ value, illustrated high T1ρ values in trypsin-degraded articular cartilage compared with those of control articular cartilage. Similarly, loss of type II collagen was confirmed by microscopy and immunohistochemical staining with a rabbit polyclonal antibody against type II collagen. The histological samples of normal articular cartilage showed a dense and homogeneous distribution of collagen II fibers (Fig. 3A), whereas those of the collagenase-degraded articular cartilage showed observable decreases in collagen II fibers on the immunohistochemically stained specimens (Fig. 3B).
Our results show that 3-T MRI and T1ρ mapping can be used to quantify loss of PGs and collagen II fibers. Because cartilage damage is irreversible, quantification and early detection of osteoarthritis is important. Our results agree with those of previous studies. Duvvuri et al. (18) suggested that T1ρ measurements are selectively sensitive to PG content and can potentially distinguish early degenerative changes in cartilage associated with osteoarthritis. Li et al. (19) demonstrated that the T1ρ value is significantly but moderately correlated with PG content and reflects the degree of histological cartilage degeneration. Nishioka et al. (8) revealed that the T1ρ value reflects cartilage glycosaminoglycan content and indicates in vivo cartilage degeneration. They also insisted that a noninvasive diagnosis and evaluation of cartilage degeneration can be facilitated using the T1ρ value. Thus, similar with a previous study, our results suggest that T1ρ MRI has the potential to provide noninvasive imaging biomarkers for cartilage degeneration by measuring articular cartilage T1ρ values, which are affected by PG content. This process could enable early detection and treatment of degenerative arthritis, which could prevent invasive treatments, such as arthroplasty with an artificial joint.
A few controversial studies have reported a relationship between the articular cartilage T1ρ value and collagen fiber content. Menezes et al. (20) reported a relationship between T1ρ value and collagen concentration. However, Duvvuri et al. (18) revealed that the T1ρ value does not increase in collagen-degraded bovine patellae. Li et al. (19) also reported no correlation between the T1ρ value and collagen content in degenerated human cartilage. In our study, the T1ρ value increased in the collagen-degraded specimens but less than that in the PG-degraded specimens. We postulate that changes in the T1ρ value based on collagen content are small in articular cartilage; thus, the controversial results reported by previous studies may have been dependent on the study population or design. Our results suggest that both PG and collagen contents should be considered at the time of application in clinical practice.
Our study had several limitations. First, the number of specimens was small. However, we showed significant differences in T1ρ values among the three different enzyme groups. Second, quantitative biochemical assays were not performed to assess loss of PG and type II collagen in articular cartilage. The histological staining only showed the qualitative loss of macromolecules. We revealed the feasibility of T1ρ mapping using a clinical MRI scanner to quantify and evaluate cartilage degeneration; therefore, further studies, including an evaluation of in vivo animal joints, should be conducted to apply this process in clinical practice.
In conclusion, degradation of articular cartilage PG and collagen fiber content increased the T1ρ value of articular cartilage.
Figures and Tables
Fig. 1
Reconstruction of T1ρ map and measurement of T1ρ value.
A. Image shows result of T1ρ map using color scale.
B. Image shows positioning of region of interest to calculate the T1ρ value of full-thickness cartilage. Region of interest was placed on the patellar cartilage with a freehand drawing technique.
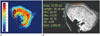
Fig. 2
Safranin-O staining for proteoglycans.
A. In Safranin-O staining, control cartilage showed a dense and homogeneous distribution of proteoglycans.
B. In Safranin-O staining, trypsin degraded articular cartilage showed observable decreases of proteoglycans.
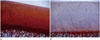
Fig. 3
Immunohistochemical staining for type II collagen.
A. In immunohistochemical staining with antibodies against type II collagen, control cartilage showed a dense and homogeneous distribution of collagen II fibers.
B. In immunohistochemical staining with antibodies against type II collagen, collagenase degraded articular cartilage showed observable decreases of collagen II fibers.
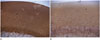
Table 1
Results of T1ρ Value of Articular Cartilage for Each Group
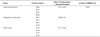
References
1. Shapiro LM, McWalter EJ, Son MS, Levenston M, Hargreaves BA, Gold GE. Mechanisms of osteoarthritis in the knee: MR imaging appearance. J Magn Reson Imaging. 2014; 39:1346–1356.
2. Buckwalter JA, Mankin HJ. Articular cartilage: degeneration and osteoarthritis, repair, regeneration, and transplantation. Instr Course Lect. 1998; 47:487–504.
3. Buckwalter JA, Mankin HJ. Articular cartilage repair and transplantation. Arthritis Rheum. 1998; 41:1331–1342.
4. Burstein D, Gray M, Mosher T, Dardzinski B. Measures of molecular composition and structure in osteoarthritis. Radiol Clin North Am. 2009; 47:675–686.
5. Bashir A, Gray ML, Boutin RD, Burstein D. Glycosaminoglycan in articular cartilage: in vivo assessment with delayed Gd(DTPA)(2-)-enhanced MR imaging. Radiology. 1997; 205:551–558.
6. Taylor C, Carballido-Gamio J, Majumdar S, Li X. Comparison of quantitative imaging of cartilage for osteoarthritis: T2, T1rho, dGEMRIC and contrast-enhanced computed tomography. Magn Reson Imaging. 2009; 27:779–784.
7. Li X, Benjamin Ma C, Link TM, Castillo DD, Blumenkrantz G, Lozano J, et al. In vivo T(1rho) and T(2) mapping of articular cartilage in osteoarthritis of the knee using 3 T MRI. Osteoarthritis Cartilage. 2007; 15:789–797.
8. Nishioka H, Hirose J, Nakamura E, Oniki Y, Takada K, Yamashita Y, et al. T1ρ and T2 mapping reveal the in vivo extracellular matrix of articular cartilage. J Magn Reson Imaging. 2012; 35:147–155.
9. Stahl R, Luke A, Li X, Carballido-Gamio J, Ma CB, Majumdar S, et al. T1rho, T2 and focal knee cartilage abnormalities in physically active and sedentary healthy subjects versus early OA patients--a 3.0-Tesla MRI study. Eur Radiol. 2009; 19:132–143.
10. Trattnig S, Mlynárik V, Breitenseher M, Huber M, Zembsch A, Rand T, et al. MRI visualization of proteoglycan depletion in articular cartilage via intravenous administration of Gd-DTPA. Magn Reson Imaging. 1999; 17:577–583.
11. Mosher TJ, Dardzinski BJ, Smith MB. Human articular cartilage: influence of aging and early symptomatic degeneration on the spatial variation of T2--preliminary findings at 3 T. Radiology. 2000; 214:259–266.
12. Dunn TC, Lu Y, Jin H, Ries MD, Majumdar S. T2 relaxation time of cartilage at MR imaging: comparison with severity of knee osteoarthritis. Radiology. 2004; 232:592–598.
13. Prasad AP, Nardo L, Schooler J, Joseph GB, Link TM. T1ρ and T2 relaxation times predict progression of knee osteoarthritis. Osteoarthritis Cartilage. 2013; 21:69–76.
14. Akella SV, Regatte RR, Gougoutas AJ, Borthakur A, Shapiro EM, Kneeland JB, et al. Proteoglycan-induced changes in T1rho-relaxation of articular cartilage at 4T. Magn Reson Med. 2001; 46:419–423.
15. Mlynárik V, Trattnig S, Huber M, Zembsch A, Imhof H. The role of relaxation times in monitoring proteoglycan depletion in articular cartilage. J Magn Reson Imaging. 1999; 10:497–502.
16. Borthakur A, Shapiro EM, Beers J, Kudchodkar S, Kneeland JB, Reddy R. Sensitivity of MRI to proteoglycan depletion in cartilage: comparison of sodium and proton MRI. Osteoarthritis Cartilage. 2000; 8:288–293.
17. Shibata S, Fukada K, Suzuki S, Yamashita Y. Immunohistochemistry of collagen types II and X, and enzyme-histochemistry of alkaline phosphatase in the developing condylar cartilage of the fetal mouse mandible. J Anat. 1997; 191(Pt 4):561–570.
18. Duvvuri U, Reddy R, Patel SD, Kaufman JH, Kneeland JB, Leigh JS. T1rho-relaxation in articular cartilage: effects of enzymatic degradation. Magn Reson Med. 1997; 38:863–867.
19. Li X, Cheng J, Lin K, Saadat E, Bolbos RI, Jobke B, et al. Quantitative MRI using T1ρ and T2 in human osteoarthritic cartilage specimens: correlation with biochemical measurements and histology. Magn Reson Imaging. 2011; 29:324–334.
20. Menezes NM, Gray ML, Hartke JR, Burstein D. T2 and T1rho MRI in articular cartilage systems. Magn Reson Med. 2004; 51:503–509.