Abstract
Purpose
To evaluate the diagnostic performance of dual-energy computed tomography (DECT) in detecting traumatic bone marrow lesions in patients with acute knee injury.
Materials and Methods
Between August 2011 and June 2012, 22 patients presenting with an acute knee injury, including 4 patients who were referred for bilateral knee trauma, underwent DECT (80 kVp and 140 kVp) and MR imaging. DECT data were postprocessed using a three-dimensional, color-coded, virtual non-calcium technique (VNC). DECT data were graded by 2 blinded independent readers using a four-point system (1 = distinct bone marrow lesion, 2 = less distinct bone marrow lesion, 3 = equivocal, 4 = none) for 6 femoral and tibial regions and 2 patellar regions. Routine MR knee imaging served as the reference standard.
Results
MR images showed bone bruises in 81 of 364 regions. The overall sensitivity, specificity, positive predictive value, and negative predictive value of DECT for bone bruises were 65.4%, 98.2%, 91.4%, and 90.8%, respectively, for Reader 1 and 70.3%, 93.6%, 76.0%, and 91.7%, respectively, for Reader 2. In particular, tibial bone bruises could be found more easily with better sensitivity (80.2%).
Bone bruises are frequently identified on magnetic resonance imaging (MRI) after an injury to the musculoskeletal system. The terms "bone contusion", "bone bruise", and "bone marrow edema" have appeared in published reports for at least a decade and are used synonymously (1).
It is presumed that bone bruises are the result of trabecular microfractures. They can cause pain in the absence of substantial soft-tissue injuries (2). Two-year follow-up studies suggest that 10-15% of patients have persistent marrow edema and up to one-third have some evidence of subchondral osteonecrosis or articular cartilage degeneration (3). Early diagnosis with appropriate therapy including restriction or cessation of activity is important to prevent complications.
In evaluating the musculoskeletal system for soft-tissue and bone abnormalities after trauma, MRI is an excellent imaging modality (4). A bone bruise is defined as a traumatically involved poorly-marginated area of signal loss on T1-weighted images (T1WIs) involving the subcortical bone with increased signal intensity on T2-weighted images (T2WIs) (5). This appearance is thought to represent areas of hemorrhage, edema, or infarction secondary to trabecular microfracture (6). MRI sensitivity for bone bruises is reported to be 91-96% and the specificity 86-96% (1). However, MRI is not routinely performed in all patients with knee trauma in the clinical setting. Multidetector computed tomography is able to depict the bony structure and even small fractures with high accuracy, however, it does not allow for the evaluation of the bone marrow.
By subtracting calcium from cancellous bone, dual-energy computed tomography (DECT) with the virtual non-calcium (VNC) technique enables assessment of the bone marrow and may detect bone bruises around the knee (7). The purpose of this randomized, controlled study was to evaluate traumatic bone marrow lesions with DECT in comparison with MR imaging in patients with acute knee trauma.
This study was approved by our institutional review board for the retrospective data analysis of DECT scans.
Between August 2011 and June 2012, 73 patients with acute knee trauma were eligible for this study. All patients had been referred for CT to rule out fracture based on plain radiography or clinical suspicion, and had undergone DECT. The 23 patients who also underwent MRI within 1 week were included. Exclusion criteria were age less than 18 years, previous knee trauma or surgery within 1 year, metal implant, and pregnancy. One patient who had a benign osteolytic lesion involving the lateral femoral condyle was excluded. Bilateral knee DECT was performed in 4 patients. The final data for analysis were 26 knee joints in 22 patients (11 men, 11 women; mean age, 48.9 years ± 14; age range, 18-72 years).
To compare radiation dosimetry, 25 patients (14 men, 11 women; mean age, 57.4 years ± 14.6; age range, 28-83 years) who had visited the emergency room for knee trauma from January 2011 to July 2011 were randomly recruited into the control group. Single-energy CT scans were done for 26 knee joints in this group and the radiation dose for each scan was recorded.
All CT scans were obtained using a dual-source CT scanner (Somatom Definition Flash; Siemens Healthcare, Forchheim, Germany). This CT scanner is equipped with 2 X-ray tubes and 2 detectors rotating with an angular offset of 90°. We performed noncontrast DECT scans of the knee in the craniocaudal direction with the following scanning parameters: collimation, 20 × 0.6 mm; pitch, 0.7; rotation time, 0.5 s; tube voltages, 80 kVp (tube A) and 140 kVp (tube B); tube current ratio, approximately 1 : 4 (tube A, 50 reference mAs; tube B, 213 reference mAs); and auto-mA (Care dose, CAREDOSE 4D program, Siemens Medical Solutions, Forchheim, Germany). Routine single-energy CT examinations were acquired in the control group with the same scanner (tube voltage, 120 kVp; 172 reference mAs).
A weighted average image acquired with the DECT was computed from the 80 kVp and 140 kVp data with a ratio of 0.7 : 0.3 to resemble the contrast characteristics of a single-energy 120-kVp image. Axial images were reconstructed with a section thickness of 1 mm, with 0.7 mm increments, using a soft-tissue convolution kernel (D30f) and bone kernel (B70f). Coronal and sagittal images were reconstructed with the bone kernel (B70f). Postprocessing was performed using a dedicated workstation equipped with commercial DE postprocessing software (Syngo Dual Energy, version VA34A; Siemens Healthcare, Forchheim, Germany). A dual-energy 3-material decomposition algorithm was used to differentiate yellow marrow and red marrow for imaging analysis (8). Using the knee VNC application (Syngo Dual Energy, version VA34A; Siemens Healthcare, Forchheim, Germany), three-dimensional color-coded VNC images were obtained for further analysis (knee VNC setting of Syngo Dual Energy, Siemens Healthcare, Forchheim, Germany).
The effective radiation dose of DECT was calculated as the product of the CT dose index and compared with that of the routine protocol.
All MRIs were performed using a 1.5-T unit (Intera; Philips Medical Systems, Best, the Netherlands) with a dedicated knee surface four-element phased-array coil. MR imaging was performed with the patient in the conventional supine position. The standard MR imaging protocol used at the authors' hospital for all patients with knee trauma was as follows: sagittal spin-echo proton-density images (repetition time ms/echo time ms, 2401/20; field of view, 170 × 170; matrix, 320 × 273; section thickness, 3.3 mm; gap, 0.3 mm), sagittal fat-suppressed fast spin-echo T2WIs (2975/50; field of view, 170 × 170; matrix, 292 × 235; section thickness, 3.3 mm; gap, 0.3 mm), coronal fat-suppressed spin-echo proton-density images (2715/20; field of view, 170 × 170; matrix, 268 × 266; section thickness, 3.5 mm; gap, 0.35 mm), coronal spin-echo T1WIs (600/13; field of view, 170 × 170; matrix, 324 × 259; section thickness, 4 mm; gap, 0.35 mm), axial spin-echo T1WIs (551/15; field of view, 160 × 160; matrix, 256 × 204; section thickness, 4.5 mm; gap, 0.45 mm), and axial fat-suppressed fast spin-echo T2WIs (1454/60; field of view, 160 × 160; matrix, 300 × 277; and section thickness, 4.5 mm; gap, 0.45 mm).
For image analysis, the knee joint was divided into 14 anatomic regions: the anterior, middle and posterior aspects of the medial and lateral femoral condyles; the anterior, middle and posterior aspects of the medial and lateral tibial plateau; and the medial and lateral facets of the patella.
Two radiologists (J.S.K. and S.S.H.), who had 25 and 3 years of experience in radiology, respectively, independently reviewed the DECT images in random order. Both readers were blinded to each other and to the clinical information. A bone bruise on a color-coded VNC image was defined as a geographic dark color area on the bone marrow. The readers independently assessed the image using a four-point classification system (Fig. 1): grade 1, distinct bone marrow lesion; grade 2, less distinct bone marrow lesion; grade 3, equivocal lesion; and grade 4, normal bone marrow. Lesions that were within 2 mm of the adjacent cortical bone were excluded to avoid a beam hardening artifact (7). The presence or absence of fractures was recorded for all patients.
Four weeks later, to avoid any potential recall bias, MRI examinations were reviewed by Reader 1 as the standard of reference. On the MR images, the definition of a bone bruise was an area of poorly marginated signal intensity alteration (decreased signal intensity on T1WI, increased signal intensity on T2WI, or both) (4).
For calculation of sensitivity, specificity, positive predictive value (PPV), negative predictive value (NPV), and accuracy, we considered grades 1 and 2 as positive and grades 3 and 4 as negative. MR imaging served as the standard of reference. Weighted Kappa (κ) statistics were used to assess the interobserver agreement for semiquantitative image-quality grades. All statistical analysis was performed using 2 software packages (MedCalc; MedCalcSoftware, Mariakerke, Belgium) and SPSS 11.5 (SPSS Inc., Chicago, IL, USA).
We analyzed 364 anatomic regions in 26 knee joints of 22 patients. Fractures were noted on DECT images in 18 of 26 (69.2%) knee joints. In 11 patients, they were noted in the tibial plateau; in 2, the femoral condyle; in 4, the patella; and in one, both the femoral condyle and patella. The mean interval time between the DECT and MRI was 3.4 days (range, 0-12 days).
On DECT images, Reader 1 assigned 52 regions as grade 1, 6 regions grade 2, and 306 regions grade 4. Reader 2 assigned 54 regions as grade 1, 21 regions grade 2, 43 regions grade 3, and 246 regions grade 4 (Table 1).
MR imaging showed a total of 81 regions with bone bruises; 28 were confined to femoral regions, 43 were in tibial regions, and 10 were in patellar regions (Fig. 2).
Reader 1 achieved an overall sensitivity of 65.4%, a specificity of 98.2%, a PPV of 91.4%, a NPV of 90.8%, and an accuracy of 90.9%; Reader 2 achieved values of 70.3% sensitivity, 93.6% specificity, 76.0% PPV, 91.7% NPV, and 88.5% accuracy. Twenty-eight false-negative and 5 false-positive findings were made by Reader 1. Twenty-four false-negatives and 18 false-positive findings were made by Reader 2 (Figs. 3, 4). In the comparison among the 3 compartments of the knee, interpretations in the tibial regions showed high sensitivity and specificity, but in the patellar region, they showed low sensitivity and high specificity (Table 2).
Among 6 lesions that were diagnosed as less distinct lesions by Reader 1, 4 (66.7%) were diagnosed as positive on the MRI. Nine lesions (42.7%) out of 21 lesions that were diagnosed as less distinct lesions by Reader 2 were diagnosed as positive on the MRI. There were no equivocal lesions diagnosed by Reader 1, and 43 equivocal lesions were diagnosed by Reader 2. Among them, 39 (90.7%) were diagnosed as negative.
The CT dose index value was 12.1 mGy ± 1.5, and the mean dose-length product was 231 mGy·cm ± 38. This is comparable to our current conventional CT protocol of the knee joint, in which the volume CT dose index was 10.5 mGy ± 1.2 and the mean dose-length product was 242 mGy·cm ± 91. The mean percentage of increased radiation exposure for DECT was 12.0%.
The overall interobserver agreement for the qualitative analysis of color-coded VNC images between the readers was good (k = 0.707).
"Bone bruise" describes a bony lesion caused by edema and hemorrhage of bone marrow. Its underlying process is believed to be fat cell destruction and fibrovascular regeneration. It is a generally established term in MRI. A bone bruise appears as an ill-defined hyperintensity on STIR images with a hypointense signal on T1WIs (9, 10).
Conventional CT has its limitations in its ability to detect bone bruises. In a previous study, bone bruise was visualized as an increased density in the trabecular bone in the injured hip when compared to the contralateral hip. Since this was a result of a comparison with the contralateral side, it may not be applicable in practice (11).
Recently, DECT using the VNC technique has been exploited for better visualization of attenuation changes in the bone marrow, therefore, increasing detectability of marrow abnormalities. Subtraction of calcium from cancellous bone allows us to use the different attenuations at different X-ray energies to evaluate the marrow (7, 12). In this study, we assessed the clinical usefulness of the VNC technique for bone marrow edema around the knee joint. There have only been a few reports on the detectability of bone bruises with DECT. Pache et al. (7) described a DECT VNC technique that can detect bone bruises around the knee with high sensitivity (86.4%, 86.4%) and specificity (94.4%, 95.5%). CT numbers between normal and edematous bone marrow showed close correlation with the MRI. Guggenberger et al. (12) noted that when strict image interpretation on MR imaging (distinct bone marrow lesion was positive) was done in ankle trauma patients, DECT showed high sensitivity (90.0%) and excellent NPV (99.1%). But a when distinct bone marrow lesion and less distinct bone marrow lesion were included in the diagnosis on MR imaging, DECT showed less sensitivity (72.1%), with a lower NPV (93.6%). These studies evaluated bone bruises using various methods such as gray level-coded and color-coded images and quantitative analysis. We used color-coded images only, which are readily available and relatively simple for bone bruise evaluation. However, our study showed lower sensitivity compared to the previous studies.
In this study, the three-dimensional color-coded VNC images reconstructed from the DECT showed moderate sensitivity (65.4%, 70.3%) and very good specificity (98.2%, 93.6%) for the detection of traumatic bone marrow lesions, using MRI as a standard of reference. We included less distinct bone marrow lesions as a positive finding. Although overall interobserver agreement was good, there were differences between the readers for less distinct lesions and equivocal lesions. This might be due to the readers' different approaches to the grading system.
We found a higher sensitivity and specificity for the bone marrow lesions in the tibial plateau than in the femoral condyles. This may be because more fractures were present in the tibial plateau in our patient group. Patellar bone marrow lesions were difficult to detect, with low sensitivity. There are several hypotheses for this result. First, the patella has a small volume of trabecular bone. Additionally, Hoffa"s fat pad can resemble patellar bone marrow in color-coded images with similar attenuation.
Bone bruises around the knee joints have often been used as secondary signs of other associated abnormalities (13). Bone bruises most commonly occur in association with a tear of the anterior cruciate ligament (ACL). They are noted in more than 80% of patients after acute rupture of the ACL and there is a similar incidence with ruptures of the posterior cruciate ligament (14). The pattern of bone marrow edema after trauma is like a fingerprint; the type of injury can be deduced by studying the distribution of bone marrow edema on the MRI (4).
Bone bruises areas associated with pain. The time to resolution of bone bruising varies from 3 weeks to 2 years, according to the severity of injury, extent of bone bruising, associated internal knee derangement, and post-injury rehabilitation (15, 16).
There were several limitations to our study. First, the study group was too small to prove the effect of various factors affecting image quality. Only 26 knee joints in 22 patients with acute knee trauma were included. Second, we did not quantitatively evaluate CT attenuation of bone marrow on the VNC images. We only focused on the clinical performance of DECT in detecting traumatic bone bruises. Third, we used MRI as the reference standard for the presence of bone bruises, without clinical follow-up. Fifth, the fact that MRI was not performed in all patients with acute knee trauma that underwent DECT might have been due to selection bias for the type of injury. Finally, both readers did not have enough experience in using the DECT VNC technique, and they had some difficulty in defining less distinct bone marrow lesions.
In conclusion, our study shows that traumatic bone bruises of the knee joint can be diagnosed on color-coded VNC images with DECT, by using a subjective grading system, with good sensitivity and excellent specificity compared with MR images. This DECT protocol causes only 12% additional radiation compared to our routine single-energy CT protocol. The color-coded VNC technique of DECT may be useful to detect traumatic bone bruises and may be used as a screening tool to predict possible associated soft tissue injuries.
Figures and Tables
Fig. 1
Four-point grading system for axial color-coded VNC image (left) and corresponding T2-weighted STIR MR images (right).
A. Grade 1: distinct bone marrow lesion, color-coded VNC images depict bone marrow abnormalities (arrow) in the same area as seen on the MR image (arrow).
B. Grade 2: less distinct bone marrow lesion, "probable" bone marrow abnormality on color-coded VNC image (arrows). Corresponding MR image shows bone bruise in the same area (arrows).
C. Grade 3: equivocal lesion, probably no bone marrow lesion on color-coded VNC image (arrow). MR image shows no bone bruise in medial femoral condyle.
D. Grade 4: normal bone marrow, no abnormality on either modality.
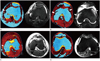
Fig. 2
A 23-year-old man with acute knee trauma.
A. Sagittal weighted-average image simulating single-energy CT shows no fracture and normal trabecular structure.
B. Color-coded VNC image shows bone bruise in the medial condyle of the femur (arrow).
C. T2-weighted STIR MR image shows diffuse bone marrow lesion in the medial femoral condyle (arrow).
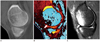
Fig. 3
A 72-year-old woman with acute knee trauma.
A. Axial weighted-average image simulating single-energy CT shows no fracture.
B. Color-coded VNC image shows no traumatic lesion in the medial femoral condyle.
C. T2-weighted STIR MR image shows bone marrow edema in the medial femoral condyle (arrow).
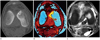
Fig. 4
A 61-year-old man with acute knee trauma.
A. Sagittal weighted-average image simulating single-energy CT shows normal trabecular bone structure without fracture.
B. Color-coded VNC image shows dark colored area in medial tibialplateau of knee joint (arrow).
C. T2-weighted STIR MR image shows no bone bruise in medial tibial plateau (arrow).
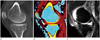
References
1. Terzidis IP, Christodoulou AG, Ploumis AL, Metsovitis SR, Koimtzis M, Givissis P. The appearance of kissing contusion in the acutely injured knee in the athletes. Br J Sports Med. 2004; 38:592–596.
2. Boks SS, Vroegindeweij D, Koes BW, Hunink MG, Bierma-Zeinstra SM. Follow-up of occult bone lesions detected at MR imaging: systematic review. Radiology. 2006; 238:853–862.
3. Costa-Paz M, Muscolo DL, Ayerza M, Makino A, Aponte-Tinao L. Magnetic resonance imaging follow-up study of bone bruises associated with anterior cruciate ligament ruptures. Arthroscopy. 2001; 17:445–449.
4. Sanders TG, Medynski MA, Feller JF, Lawhorn KW. Bone contusion patterns of the knee at MR imaging: footprint of the mechanism of injury. Radiographics. 2000; 20 Spec No:S135–S151.
5. Vincken PW, Ter Braak BP, van Erkel AR, Coerkamp EG, Mallens WM, Bloem JL. Clinical consequences of bone bruise around the knee. Eur Radiol. 2006; 16:97–107.
6. Nakamae A, Engebretsen L, Bahr R, Krosshaug T, Ochi M. Natural history of bone bruises after acute knee injury: clinical outcome and histopathological findings. Knee Surg Sports Traumatol Arthrosc. 2006; 14:1252–1258.
7. Pache G, Krauss B, Strohm P, Saueressig U, Blanke P, Bulla S, et al. Dual-energy CT virtual noncalcium technique: detecting posttraumatic bone marrow lesions--feasibility study. Radiology. 2010; 256:617–624.
8. Fischer MA, Gnannt R, Raptis D, Reiner CS, Clavien PA, Schmidt B, et al. Quantification of liver fat in the presence of iron and iodine: an ex-vivo dual-energy CT study. Invest Radiol. 2011; 46:351–358.
9. Ryu KN, Jin W, Ko YT, Yoon Y, Oh JH, Park YK, et al. Bone bruises: MR characteristics and histological correlation in the young pig. Clin Imaging. 2000; 24:371–380.
10. Zanetti M, Bruder E, Romero J, Hodler J. Bone marrow edema pattern in osteoarthritic knees: correlation between MR imaging and histologic findings. Radiology. 2000; 215:835–840.
11. Geijer M, Dunker D, Collin D, Göthlin JH. Bone bruise, lipohemarthrosis, and joint effusion in CT of non-displaced hip fracture. Acta Radiol. 2012; 53:197–202.
12. Guggenberger R, Gnannt R, Hodler J, Krauss B, Wanner GA, Csuka E, et al. Diagnostic performance of dual-energy CT for the detection of traumatic bone marrow lesions in the ankle: comparison with MR imaging. Radiology. 2012; 264:164–173.
13. Mandalia V, Fogg AJ, Chari R, Murray J, Beale A, Henson JH. Bone bruising of the knee. Clin Radiol. 2005; 60:627–636.
14. Mair SD, Schlegel TF, Gill TJ, Hawkins RJ, Steadman JR. Incidence and location of bone bruises after acute posterior cruciate ligament injury. Am J Sports Med. 2004; 32:1681–1687.
15. Mandalia V, Henson JH. Traumatic bone bruising--a review article. Eur J Radiol. 2008; 67:54–61.
16. Boks SS, Vroegindeweij D, Koes BW, Bernsen RM, Hunink MG, Bierma-Zeinstra SM. MRI follow-up of posttraumatic bone bruises of the knee in general practice. AJR Am J Roentgenol. 2007; 189:556–562.