Abstract
Purpose
To compare coronary computed tomography angiography (CTA) with single photon emission computed tomography-myocardial perfusion imaging (SPECT-MPI) for the detection of physiologically significant coronary artery disease (CAD).
Materials and Methods
We evaluated 202 patients undergoing 64-slice coronary CTA and 201-Tl SPECT-MPI within a 3-month time interval. In addition, 68 patients underwent invasive coronary angiography (ICA). Coronary artery stenoses with luminal narrowing ≥50% were defined as "significant" on CTA and ICA. All myocardial segments were classified as reversible or fixed perfusion defects and normal segments on 201-Tl SPECT-MPI, and were allocated to the corresponding coronary vessels. Agreement and diagnostic performance between each imaging modality for physiologically significant CAD was calculated using the kappa (κ) statistic and receiver operating characteristic analysis, respectively.
Results
The sensitivity and specificity of CTA for the detection of physiologically significant CAD were 88% and 86% by patient-based analysis, and 84% and 91% by vessel-based analysis as compared to 201-Tl SPECT-MPI, respectively. The agreement between CTA and SPECT-MPI was good (κ = 0.647) and moderate (κ = 0.558) by patient- and vessel-based analyses, respectively. The accuracy of CTA for predicting perfusion defects on SPECT-MPI was comparable (area under the curve; 0.814 vs. 0.819, p=0.902 on patient-based analysis, and 0.808 vs. 0.749, p=0.197 on vessel-based analysis) to ICA.
Myocardial perfusion imaging (MPI) with single photon emission computed tomography (SPECT) is a well accepted and widely used method to evaluate the physiological importance of coronary artery stenosis (1). Although invasive coronary angiography (ICA) is the generally accepted gold standard for the detection of significant coronary artery stenosis, morphological information depicted with ICA offers limited physiological and prognostic information (23). More recently, multidetector computed tomography (MDCT) has been proposed as an alternative imaging modality for the evaluation of patients with suspected CAD, and shows high sensitivity and specificity for the detection of significant (≥50% luminal narrowing) stenoses (45). Additionally, CT angiography (CTA) provides information regarding the presence of atherosclerosis, unlike ICA (6). However, recent reports of studies in small groups of patients (789) indicate that CTA has only limited positive predictive value (PPV) for the detection of lesions that induce perfusion defects on MPI. Furthermore, these previous studies have not addressed the relationship among the total calcium score, stenosis severity, and perfusion defects for SPECT-MPI. Thus, the purpose of our study was to compare coronary CTA with SPECT-MPI in the detection of physiologically significant CAD, and to explore the relative merits of CTA over ICA or SPECT-MPI in patients who underwent ICA.
We studied 280 consecutive patients with suspected CAD who underwent 64-slice coronary CTA and 201-Tl SPECT-MPI at our hospital from January 2005 to March 2010. We excluded 41 patients with known CAD defined by a history of coronary artery revascularization such as a coronary artery bypass graft (CABG, n=19), intracoronary stent (n=17) and history of myocardial infarction (n=5). We also excluded 36 patients who underwent ICA between CTA and SPECT, and 1 patient with an aberrant right coronary artery (RCA) from the pulmonary trunk. The remaining 202 patients represent the subjects of this study. The pretest likelihood of CAD was determined according to the Morise method using cut-offs of < 9, > 18, and in between for low, high, and intermediate pretest likelihood, respectively (10). Our institutional review board approved the study design and waived the requirement for informed patient consent for this retrospective analysis.
The mean interval between CTA and SPECT-MPI was 35 ± 28 days (range, 0 - 90 days). CTA was performed after SPECT-MPI in 135 patients (67%), prior to SPECT-MPI in 63 patients (31%), and the same day in 4 patients (2%). These patients did not experience changes in symptoms between the coronary CTA and SPECT. Sixty-eight patients (34%) were additionally investigated by ICA for the further diagnosis and intervention of CAD. The circumstances involving the need to perform an ICA were as follows; abnormal coronary CTA (n=14) resulting in stenosis ≥ 50%, abnormal myocardial perfusion scan (n=1), abnormalities on both examinations (n=25), or persistent symptoms (n=28) after normal examinations. The mean time interval between the last non-invasive study (either CTA or SPECT-MPI) and ICA was 13 ± 4 days (range, 1 - 19 days).
Multidetector CT data were acquired using Brilliance 64-slice CT (Philips Medical Systems, Eindhoven, The Netherlands). All patients underwent non-enhanced imaging to calculate the total calcium score before CTA. The imaging parameters were 120 kV and 55 mAs with prospective electrocardiogram (ECG)-gating. For the purpose of obtaining the best image quality, CTA was performed using a retrospective ECG-gating technique without dose modulation. The image acquisition parameters were as follows: 400 msec gantry rotation time, detector collimation of 64 × 0.625 mm, apitch of 0.2, effective tube current of 600 - 900 mAs, and a tube voltage of 120 kV. The exclusion criteria were as follows: 1) known intolerance of iodinated contrast agents; 2) impaired renal function (serum creatinine >120 mmol/l); 3) cardiac arrhythmia or atrial fibrillation; and 4) pregnancy. If the patient had an elevated heart rate (≥ 70 bpm), without contraindications to beta-blockers, an oral beta-blocker (50 - 100 mg of metoprolol) was administered 1 hour before the examination according to our practice in daily clinical routine. Sublingual nitroglycerin (0.4 mg) was administered 1 min before image acquisition to dilate the coronary arteries if there were no contraindications. An 80 mL volume of a contrast medium (iomeprol, Iomeron 400; Bracco, Milano, Italy) was injected intravenously at a rate of 4.5 mL/s, and followed by a 40-mL saline flush at 4 mL/sec. Bolus tracking in the descending thoracic aorta with a threshold of 150 HU was used for timing, and a delay of 6 s was added to the arrival time.
Reconstructions were performed routinely at the 0%, 35%, 45%, and 75% phases of the R-R interval period. The 75% phases of the R-R interval period were first used for coronary analysis. If indicated, additional cardiac cycles were explored. Trans-axial images (slice thickness, 0.9 mm; increment, 0.45 mm) were reconstructed using an ECG-gated half-rotation reconstruction algorithm and a sharp kernel (filter of XCC). The dose-length product was recorded for each CT examination, and the effective radiation dose equivalent was calculated according to the following formula: effective radiation dose equivalent (in millisieverts) = dose-length product × a conversion factor of 0.017 (11).
MDCT data were transferred to a workstation (Extended Brilliance workspace; Philips Medical Systems) for post-processing. Oblique multi-planar reformations (MPRs), maximum intensity projections (MIPs), curved MPRs along the axis of each vessel, 2-dimensional (2-D) map images, and volume-rendered techniques (VRTs) were reconstructed on the dedicated workstation. Two experienced readers (D.K. Kang and J.H. Ma), who were both blinded to the clinical history and to the findings from MPI and ICA, performed image analyses by consensus using a picture archiving and a communication system (PACS, PiviewSTAR 5051; INFINITT, Seoul, Korea).
CTAs were evaluated by patient- and vessel-based analyses. Each coronary artery was visually classified as "evaluable" or "unevaluable" (presence of severe motion artifacts, stair-step artifacts, image noise, or heavy calcifications). The degree of stenosis was classified as completely normal, non-significant stenosis, and significant stenosis. Significant stenosis was defined as the narrowing of the coronary diameter ≥ 50% with visual assessment, while non-significant stenosis was defined as narrowing < 50%. For the purpose of a vessel-based analysis, the maximum stenosis from each of the three major coronary arteries was used. If the coronary artery was unevaluable, it was censored as a positive finding in patient- and vessel-based analyses, reflecting the intentionto-diagnose the nature of the study (9). The image display settings were adjusted according to the individual contrast and noise. Thus, the window width varied from 400 - 800 HU and the level was from 150 - 300 HU. In the case of severe calcifications, the bone window setting was used to prevent the overestimation of luminal narrowing (12).
For all evaluable and diseased coronary arteries ≥ 2 mm in diameter, a quantitative analysis was also performed on a commercially available workstation (Vitrea 2, version 3.8.1; Vital Image, Minnetonka, MN, USA). Coronary artery segmentation was performed with a semi-automatic vessel detection tool on curved MPRs and cross-sectional images orthogonal to the vessel (13). Then, computerised quantitative analysis on the basis of the density-based full width half maximum method was performed (14). After two observers detected the stenotic segment by consensus, the degree of coronary stenosis was quantified by measuring vessel diameters on images orthogonal to the vessel (cross-sectional images) within the stenosis and in the reference vessels immediately proximal and distal to the stenosis.
All subjects were prepared for myocardial perfusion studies by overnight fasting, withholding of antianginal medication for 24 hr, and abstinence from caffeine products and smoking for 24 hr. Pharmacologic stress was induced by 0.14 mg/kg/min of adenosine over 6 min. Tl-201 (111 MBq) was injected at 4 min, and 10 min stress and 4 hr redistribution images were acquired using a triple-head gamma camera (MS-3; Simens, Illinois, USA), with low-energy parallel-hole collimators. Tomographs displayed in standard views were visually assessed for perfusion defects by consensus of 2 independent observers (S.J. Lee and Y.S. An) blinded to the CTA findings. Persistent mild defects, defined as areas of 65 - 80% activity on polar map displays, were disregarded in order to exclude attenuation artifacts, while all other defects were considered positive. Positive scans were divided into reversible (including partially reversible) or fixed perfusion defects. A 17-segment model was used such that myocardial segments were allocated to the territories of the different coronary arteries, as previously described (15).
All angiograms were interpreted by a single experienced reader (H.S. Lim) who was not aware of the coronary CTA and SPECT-MPI results. Each segment was quantitatively evaluated using the QCA software (Xcelera; Philips Medical Systems). Coronary artery stenoses were registered and located using the modified American Heart Association classification mentioned above (16). Analyses were performed in all vessels without excluding distal coronary segments or side branches. Significant stenosis was defined as a vessel diameter reduction ≥ 50%.
After independent analysis for each imaging modality, perfusion defects were allocated to the determining lesion for coronary CTA, and then the data from the coronary CTA and SPECT-MPI images were compared. We also added a patient-based analysis, as this provides the most clinically meaningful information for patient management. If the patient had at least 1 significant coronary artery stenosis ≥ 50%, the patient was classified as obstructive CAD, while the presence of non-significant stenosis was defined as non-obstructive CAD. In a subgroup of patients who underwent ICA, CTA was compared and correlated to ICA in allocating perfusion defects on SPECT-MPI to the determining coronary lesions (Fig. 1).
The Microsoft Excel 2007 (Microsoft Corporation, Redmond, WA, USA) software package was used for data collection. Continuous variables were described as mean values ± SD, and categorical data were expressed as percentages. Quantitative values were compared using the two-tailed Student's t-test or one-way ANOVA. Qualitative data are given in proportions and were compared using the Chi-square test. To evaluate the impact of the total calcium score for the detection of CAD, subjects were divided into 3 groups (mild [0 - 100], moderate [101 - 400], and heavy [> 400]) based on the Agatston score (17). The kappa value for quantifying agreement was interpreted as follows: poor, < 0.20; fair, 0.21 - 0.40; moderate, 0.41 - 0.60; good, 0.61 - 0.80; and very good, 0.81 - 1.00 (18). A receiver operating characteristic (ROC) analysis was applied to compare the diagnostic performance of CTA and ICA in the detection of perfusion defects, by comparing the respective areas under the ROC curve (AUCs). MedCalc (version 10.4.8; MedCalc Software, Mariakerke, Belgium) was used for all statistical analyses and a p-value < 0.05 was considered to indicate statistical significance.
The clinical characteristics of the study population are described in Table 1. The pretest likelihood of CAD was based on the Morise score (19), which was low in 43 (21%), intermediate in 136 (67%), and high in 23 (11%) patients. The mean heart rate of all subjects was 62 ±10 bpm (range, 43 - 84 bpm). The mean Agatston calcium score of the subjects was 182 ± 434 (range, 0 - 2537).
All coronary CTAs were successfully performed without failure or complications. The estimated total radiation exposure was 21 ± 2 mSv (range, 14-27 mSv) for CTA. Nineteen coronary arteries (3%) in 10 patients (5%) were unevaluable; of these, 13 coronary arteries in 8 patients were confirmed to be significant stenoses on ICA. For the patient-based analysis, a total of 59 patients (29%) had obstructive CAD with at least 1 significant ≥ 50% luminal narrowing, whereas non-obstructive CAD was observed in 70 patients (35%). On vessel-based analysis, CTA detected 91 (15%) significant stenoses, including 3 total occlusions and 133 non-significant stenoses (22%) (Table 2).
For the patient-based analysis, 42 patients (21%) had abnormal SPECT-MPI (reversible perfusion defects in 37 patients, including 8 partially reversible, fixed perfusion defects in 3 patients, and fixed and reversible defects in 2 patients). Seven patients (2%) had reversible defects in 2 coronary artery territories. For the vessel-based analysis, 51 coronary artery territories (8%) revealed reversible (n=46) or fixed perfusion (n=5) (Table 2).
Sensitivity, specificity, PPV, and negative predictive value (NPV) of CTA for the detection of physiologically significant coronary artery lesions were 88%, 86%, 63%, and 97% on patient-based analysis, and 84%, 91%, 47%, and 98% for vessel-based analysis, respectively (Fig. 1). The agreement between CTA and SPECT-MPI was good (κ=0.647; 95% CI, 0.527 - 0.766) for the patient-based analysis and moderate (κ=0.558, 95% CI, 0.457 - 0.659) for the vessel-based analysis (Fig. 2). When applying cut-off criteria ≥70% and ≥90% for significant stenoses measured by quantitative analysis, the agreement between CTA and SPECT-MPI was moderate [κ= 0.451; 95% confidence interval (CI): 0.315 - 0.586] and fair (κ = 0.326; 95% CI: 0.180 - 0.476), respectively, for the vessel-based analysis (Table 3). After applying a higher cut-off criterion, the specificity and PPV increased, while the sensitivity decreased. The negative predictive value (NPV) was maintained at a high level. The area under the curve (AUC) was highest (p = 0.002 to < 0.001) for the cut-off level ≥50% (0.878; 95% CI: 0.850 - 0.903), followed by cut-off levels of ≥70% (0.693; 95% CI: 0.655 - 0.730) and ≥90% (0.607; 95% CI: 0.567 - 0.646) (Fig. 3).
In the 160 patients with normal SPECT-MPI, CAD was observed in 88 patients (55%), including 66 patients (41%) with non-obstructive CAD. Of 555 coronary artery territories with normal perfusion on SPECT-MPI, 175 coronary arteries (32%) were narrowed, including 48 with significant stenosis ≥ 50% (Fig. 4). The higher the calcium score, the higher the prevalence of obstructive CAD on CTA (p < 0.0001) and perfusion defect on SPECT-MPI (p < 0.0001). There was poor agreement (κ = 0.077) between CTA and SPECT-MPI in patients with a high calcium score (> 400), compared to good agreement (κ= 0.633 - 0.652) in other groups (Table 4).
Among the 68 patients undergoing ICA, 36 (53%) had at least 1 significant stenosis. The remaining 32 patients (47%) were confirmed to be patients with non-obstructive CAD (n=22) or normal (n=10). Obstructive CAD consisted of 18 one-vessel diseases, 11 two-vessel diseases, and 7 three-vessel diseases. For the vessel-based analysis, 61 coronary arteries (10%) revealed significant stenosis ≥ 50%, including 5 total occlusions in RCA (n=3) and LAD (n=2). Conversely, the remaining 143 coronary arteries (90%) were non-significant stenoses (n=70) and normal patients (n=73).
The kappa values between CTA and SPECT-MPI were 0.574 and 0.571 on patient- and vessel-based analyses, respectively (Table 5). The kappa values between ICA and SPECT-MPI were 0.594 and 0.488 on patient- and vessel-based analyses, respectively (Table 5). Two of 3 patients with 3-vessel disease confirmed at ICA appeared normal on SPECT-MPI.
With ROC analyses, the optimal lesion severity cut-off for predicting ischemia was > 48% at CTA and > 49% at ICA. With a cut-off ≥ 50%, the ROC analysis showed no difference in the diagnostic performance between CTA and ICA for the detection of perfusion defects. The AUC was 0.814 (95% CI, 0.701 - 0.898) and 0.819 (95% CI, 0.706 - 0.902) for CTA and ICA, respectively, for the patient-based analysis (p=0.902). The AUC was 0.808 (95% CI, 0.719 - 0.879) and 0.749 (95% CI, 0.654 - 0.829) for CTA and ICA, respectively, for the vesselbased analysis (p = 0.197).
In the present study, 64-slice CTA was highly predictive for the exclusion of perfusion defects on SPECT-MPI with excellent NPV (97% and 98% for patient- and vessel-based analysis). The results are in agreement with those of previous studies [72% and 94% from Hacker et al. (8), as well as 93% and 99% for Gaemperli et al. (20)]. In contrast, 64-slice CTA alone is a poor predictor of the physiologic relevance of coronary artery stenoses. The PPV of CTA for the detection of any perfusion defects on SPECT-MPI in patient- and vessel-based analyses was 63% and 47%, respectively. The results are also in agreement with those of previous studies [60% and 32% of Hacker et al. (8), and 68% and 50% of Gaemperli et al (20)]. Similar results were obtained in the subgroup of patients undergoing ICA (Table 5). With increasing stenosis severity, the specificity and PPV increased, while the NPV was relatively unchanged. This result was identical to previous reports (192021), which emphasized that a higher cut-off level prevented unnecessary ICA with improved PPV. However, the sensitivity was much lower, at approximately 32% (21). Moreover, the AUC in the present study decreased significantly (p = 0.002 to < 0.001) with a higher cut-off level, unlike previous reports (1921). These observations confirmed that the severity of focal stenosis severity in itself was not sufficient to predict the physiologic significance of the coronary plaque burden. In addition, additional perfusion imaging may be required to identify patients who might benefit from revascularization therapies (9). Many factors that are beyond the simple quantification of diameter narrowing and therefore cannot be fully assessed by ICA, will eventually determine whether or not a given lesion produces stress-induced ischemia (9). Therefore, the two pieces of information obtained with myocardial perfusion imaging and morphology are difficult to directly compare (1).
On the other hand, the combined use of coronary CTA and SPECT provide incremental value for the evaluation of patients with suspicious CAD. One of the main benefits proposes the accurate allocation between morphologic stenosis severity and physiologic status of coronary artery lesions (2223). SPECT-MPI has a limited potential to specify abnormal results, thus the allocation of perfusion defects to the determining coronary lesion is impossible using MPI without morphologic correlation (24). However, our result showed only moderate agreement (κ= 0.558) for 64-slice CTA to allocate perfusion defects in SPECT-MPI to the determining coronary artery lesions. To some extent, these discrepancies may be attributable to the imperfect allocation of perfusion defects to corresponding coronary arteries, owing to individual variations in the coronary anatomy. However, the differences were also caused by the heavy calcium score, which revealed poor agreement (κ= 0.077) compared to the good agreement (κ= 0.633 - 0.652) seen in patients with normal-to-moderate calcium scores (Table 4). The result indicated that extensive calcification interferes with the accurate allocation between the severity of morphologic stenosis detected on CTA and the physiologic status of coronary artery lesions.
In the present study, 55% of patients with normal SPECT-MPI had abnormal CTA. Of 555 normal perfused coronary artery territories on SPECT-MPI, 32% had coronary artery lesions on CTA. Thus, a normal SPECT-MPI does not exclude non-obstructive CAD. On the contrary, CTA allows for the detection of CAD at a much earlier stage than does SPECT-MPI. With the introduction of CTA and comparison to MPI, a paradigm shift occurs in the definition of CAD, displacing the emphasis from inducible ischemia to atherosclerosis (7). In patients with an abnormal CTA, but normal MPI, aggressive medical therapy and risk factor modification should be considered. Although ICA has been used as the gold standard for the diagnosis of coronary narrowing and clinical decision-making for coronary intervention, atherosclerotic lesions cannot be detected in the absence of coronary artery stenoses (6). Conversely, CTA provides additional information on plaque morphology that may be useful in guiding therapeutic decisions and affect the long-term prognosis (25).
The present study had several limitations. First, there are shortcomings to using SPECT-MPI for physiologically significant coronary stenoses. SPECT-MPI has some technical limitations associated with thallium imaging, which is susceptible to a variety of artifacts, particularly attenuation artifacts due to the breast or diaphragm (1). In the present study, one attenuation artifact deemed to be a true fixed defect was allocated to the obstruction of LAD on CTA. However, considering the extensive experience accumulated over two decades, SPECT-MPI can be regarded as the best evaluated and best established non-invasive imaging tool for the functional assessment of suspected CAD. Further study using 99Tc-tetrofosmin or MIBI should be performed because of the use of X-ray-based attenuation correction and information from gated SPECT, which is known to improve the specificity of SPECT-MPI studies without affecting the sensitivity (1). Moreover magnetic resonance (MR) perfusion imaging may have advantages over nuclear methods because of its higher spatial resolution, which enables this modality to depict subendocardial hypoperfusion or three-vessel disease more accurately than nuclear methods (26). In the present study, two patients with normal SPECT-MPI and attenuation artifacts were confirmed to have three-vessel disease at ICA. Second, ICA was available to only 34% of the study population in the present study, although ICA has remained the gold standard for coronary anatomy. However, ICA was performed as considered clinically indicated and according to standard practice of our hospital. Moreover, considerable radiation exposure due to the combination of CTA and SPECT-MPI must be considered.
In summary, coronary CTA revealed statistically significant agreement with allocating perfusion defects using SPECT-MPI. Additionally, CTA may allow detection of CAD at a much earlier stage than SPECT-MPI. However, an abnormal CTA examination provided limited predictive power for physiologically significant CAD. Thus, further MPI testing is warranted in these patients.
Figures and Tables
Fig. 1
A. CTA of a 49-year-old female showing tubular significant stenosis at the mid-LAD due to soft plaque.
B. A large-sized, moderate-to-severe perfusion defect in the LAD territory on SPECT.
C. ICA revealing significant luminal narrowing of the LAD.
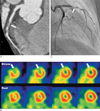
Fig. 2
A, B. CTA of an 80-year-old male with intermediate CAD risk showing multifocal calcified plaques, but no significant stenoses.
C. SPECT suggesting possible myocardial ischemia in the apical inferior wall.
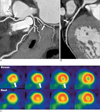
Fig. 3
Receiver operating curve of each diameter stenosis cut-off for predicting perfusion defect on SPECT-MPI shows the highest AUC for cut-off ≥ 50% (0.878) compared to cut-off of ≥ 70% (0.693) and ≥ 90% (0.607).
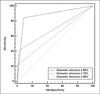
Fig. 4
A. A discrete significant stenosis at the proximal LAD with soft plaque on CTA in a 61-year-old male patient.
B. SPECT-MPI showing no significant perfusion defects.
C. This patient underwent ICA for further evaluation and diagnosed with significant stenosis at the LAD.
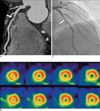
Table 2
Coronary CTA and Myocardial Tl-201 SPECT-MPI Results in a Patient- and Vessel-based Analysis
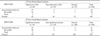
Table 3
Diagnostic Performance of Each Cut-off value for Significant Stenosis in a Vessel-based Analysis

References
1. Klocke FJ, Baird MG, Lorell BH, Bateman TM, Messer JV, Berman DS, et al. ACC/AHA/ASNC guidelines for the clinical use of cardiac radionuclide imaging: executive summary - a report of the American College of Cardiology/American Heart Association Task Force on Practice Guidelines (ACC/AHA/ASNC Committee to Revise the 1995 Guidelines for the Clinical Use of Cardiac Radionuclide Imaging). J Am Coll Cardiol. 2003; 42:1318–1333.
2. Topol EJ, Nissen SE. Our preoccupation with coronary luminology. The dissociation between clinical and angiographic findings in ischemic heart disease. Circulation. 1995; 92:2333–2242.
3. White CW, Wright CB, Doty DB, Hiratza LF, Eastham CL, Harrison DG, et al. Does visual interpretation of the coronary arteriogram predict the physiologic importance of a coronary stenosis? N Engl J Med. 1984; 310:819–824.
4. Leber AW, Knez A, von Ziegler F, Becker A, NikolaouK , Paul S, et al. Quantification of obstructive and nonobstructive coronary lesions by 64-slice computed tomography: a comparative study with quantitative coronary angiography and intravascular ultrasound. J Am Coll Cardiol. 2005; 46:147–154.
5. Ropers D, Rixe J, Anders K, K¨ttener A, Baum U, Bautz W, et al. Usefulness of multidetector row spiral computed tomography with 64- × 0.6-mm collimation and 330-ms rotation for the noninvasive detection of significant coronary artery stenoses. Am J Cardiol. 2006; 97:343–348.
6. Leber AW, Knez A, Becker A, Becker C, Ziegler F, Nikolaou K, et al. Accuracy of multidetector spiral computed tomography in identifying and differentiating the composition of coronary atherosclerotic plaques: a comparative study with intracoronary ultrasound. J Am Coll Cardiol. 2004; 43:1241–1247.
7. Schuijf JD, Wijns W, Jukema JW, Atsma DE, Roos A, Lamb HJ, et al. Relationship between noninvasive coronary angiography with multi-slice computed tomography and myocardial perfusion imaging. J Am Coll Cardiol. 2006; 48:2508–2514.
8. Hacker M, Jakobs T, Hack N, Nikolaou K, Becker C, Ziegler F, et al. Sixty-four slice spiral CT angiography does not predict the functional relevance of coronary artery stenoses in patients with stable angina. Eur J Nucl Med Mol Imaging. 2007; 34:4–10.
9. Gaemperli O, Schepis T, Valenta I, Koepfli P, Husmann L, Scheffel H, Kaufmann PA, et al. Functionally relevant coronary artery disease: comparison of 64-section CT angiography with myocardial perfusion SPECT. Radiology. 2008; 248:414–423.
10. Morise A, Evans M, Jalisi F, Shetty R, Stauffer M. A pretest prognostic score to assess patients undergoing exercise or pharmacological stress testing. Heart. 2007; 93:200–204.
11. McCollough CH. Patient dose in cardiac computed tomography. Herz. 2003; 28:1–6.
12. Dewey M, Rutsch W, Schnapauff D, Teige F, Hamm B. Coronary artery stenosis quantification using multislice computed tomography. Invest Radiol. 2007; 42:78–84.
13. Cordeiro MA, Lardo AC, Brito MS, Neto MA, Siqueira MH, Parga JR, et al. CT angiography in highly calcified arteries: 2D manual vs. modified automated 3D approach to identify coronary stenoses. Int J Cardiovasc Imaging. 2006; 22:507–516.
14. Leber AW, Johnson T, Becker A, Ziegler F, Tittus J, Nikolaou K, et al. Diagnostic accuracy of dual-source multi-slice CT-coronary angiography in patients with an intermediate pretest likelihood for coronary artery disease. Eur Heart J. 2007; 28:2354–2360.
15. Cerqueira MD, Weissman NJ, Dilsizian V, Jacobs AK, Kaul S, Laskey WK, et al. Standardized myocardial segmentation and nomenclature for tomographic imaging of the heart: a statement for healthcare professionals from the Cardiac Imaging Committee of the Council on Clinical Cardiology of the American Heart Association. Circulation. 2002; 105:539–542.
16. Austen WG, Edwards JE, Frye RL, Gensini GG, Gott VL, Griffith LS, et al. A reporting system on patients evaluated for coronary artery disease. Report of the Ad Hoc Committee for Grading of Coronary Artery Disease, Council on Cardiovascular Surgery, American Heart Association. Circulation. 1975; 51:5–40.
17. Meng L, Cui L, Cheng Y, Wu X, Tang Y, Wang Y, et al. Effect of heart rate and coronary calcification on the diagnostic accuracy of the dual-source CT coronary angiography in patients with suspected coronary artery disease. Korean J Radiol. 2009; 10:347–354.
18. Altman DG, Bland JM. Diagnostic tests 2: predictive values. BMJ. 1994; 309:102.
19. Tamarappoo BK, Gutstein A, Cheng VY, Nakazato R, Gransar H, Dey D, et al. Assessment of the relationship between stenosis severity and distribution of coronary artery stenoses on multislice computed tomographic angiography and myocardial ischemia detected by single photon emission computed tomography. J Nucl Cardiol. 2010; 17:791–802.
20. Gaemperli O, Schepis T, Koepfli P, Valenta I, Soyka J, Leschka S, et al. Accuracy of 64-slice CT angiography for the detection of functionally relevant coronary stenoses as assessed with myocardial perfusion SPECT. Eur J Nucl Med Mol Imaging. 2007; 34:1162–1171.
21. Nicol ED, Schultz C, Stirrup J, Roughton M, Padley SP, Rubens MB, et al. Defining the appropriate CTA stenosis threshold for gatekeeping to invasive angiography: 50% or 70%? Int J Cardiol. 2010; 144:297–298.
22. Gaemperli O, Husmann L, Schepis T, Koepfli P, Valenta I, Jenni W, et al. Coronary CT angiography and myocardial perfusion imaging to detect flow-limiting stenoses: a potential gatekeeper for coronary revascularization? Eur Heart J. 2009; 30:2921–2929.
23. Rocha-Filho JA, Blankstein R, Shturman LD, Bezerra HG, Okada DR, Rogers IS, et al. Incremental value of adenosine-induced stress myocardial perfusion imaging with dual-source CT at cardiac CT angiography. Radiology. 2010; 254:410–419.
24. Hacker M, Jakobs T, Matthiesen F, Nikolaou K, Becker C, Knez A, et al. Combined functional and morphological imaging consisting of gated myocardial perfusion SPECT and 16-detector multislice spiral CT angiography in the noninvasive evaluation of coronary artery disease: first experiences. Clin Imaging. 2007; 31:313–320.
25. Hausleiter J, Meyer T, Hadamitzky M, Kastrati A, Martinoff S, Schomig A. Prevalence of noncalcified coronary plaques by 64-slice computed tomography in patients with an intermediate risk for significant coronary artery disease. J Am Coll Cardiol. 2006; 48:312–318.
26. Chung SY, Lee KY, Chun EJ, Lee WW, Park EK, Chang HJ, et al. Comparison of stress perfusion MRI and SPECT for detection of myocardial ischemia in patients with angiographically proven three-vessel coronary artery disease. AJR Am J Roentgenol. 2010; 195:356–362.