Abstract
Background
Bone strength is impaired in patients with type 2 diabetes mellitus despite an increase in bone mineral density (BMD). Thiazolidinedione (TZD), a peroxisome proliferator activated receptor γ agonist, promotes adipogenesis, and suppresses osteoblastogenesis. Therefore, its use is associated with an increased risk of fracture. The aim of this study was to examine the in vitro and in vivo effects of lobeglitazone, a new TZD, on bone.
Methods
MC3T3E1 and C3H10T1/2 cells were cultured in osteogenic medium and exposed to lobeglitazone (0.1 or 1 µM), rosiglitazone (0.4 µM), or pioglitazone (1 µM) for 10 to 14 days. Alkaline phosphatase (ALP) activity, Alizarin red staining, and osteoblast marker gene expression were analyzed. For in vivo experiments, 6-month-old C57BL/6 mice were treated with vehicle, one of two doses of lobeglitazone, rosiglitazone, or pioglitazone. BMD was assessed using a PIXImus2 instrument at the baseline and after 12 weeks of treatment.
Results
As expected, in vitro experiments showed that ALP activity was suppressed and the mRNA expression of osteoblast marker genes RUNX2 (runt-related transcription factor 2) and osteocalcin was significantly attenuated after rosiglitazone treatment. By contrast, lobeglitazone at either dose did not inhibit these variables. Rosiglitazone-treated mice showed significantly accelerated bone loss for the whole bone and femur, but BMD did not differ significantly between the lobeglitazone-treated and vehicle-treated mice.
Diabetes mellitus is a chronic disease, and people with type 2 diabetes mellitus (T2DM) require oral antidiabetic drugs (OADs) for the rest of their life [1]. Therefore, it is important to identify efficacious ways to lower the blood glucose level in patients using these drugs and to understand the side effects of OADs and their effects on other organs [2]. The pathophysiology and causes of T2DM are complicated and multifactorial, and a variety of drugs with unique mechanisms of action are used to treat this disease [3]. Thiazolidinedione (TZD) is a class of OAD that acts by increasing insulin sensitivity by activating peroxisome proliferator-activated receptor γ (PPARγ), especially in muscle and adipose tissue [4]. These drugs have been shown to be effective in controlling hyperglycemia in people with T2DM. Rosiglitazone and pioglitazone are two representative TZDs [56].
Both adipocytes and osteoblasts differentiate from a common progenitor cell—the mesenchymal stem cell [7]. PPARγ is a transcription factor that regulates mesenchymal stem cell fate by favoring differentiation into adipocytes and by inhibiting differentiation into osteoblasts [8]. The TZDs exert their antidiabetic effects by activating PPARγ, and it is thought that long-term administration may adversely affect bone tissue [8910]. Consistent with this biological mechanism, the use of rosiglitazone, a representative TZD, is associated with decreased bone mineral density (BMD), impaired bone quality, and increased risk of fracture [1112]. However, each TZD has a slightly different profile, and several new TZDs have been reported to have no detrimental effects on bone [131415].
Patients with T2DM have impaired bone quality and strength, which increases their risk of fracture [16]. Therefore, it is important to consider the potential harm and benefits of each OAD on bone when selecting one for patients at high risk of fracture [17]. Lobeglitazone, a new PPARγ agonist, has been shown to lower blood glucose level and is used as an OAD in several Asian countries [18]. We recently reported that a 52-week treatment with lobeglitazone had no detrimental effects on BMD (unpublished data). In the present study, we investigated the effects of lobeglitazone on osteoblast differentiation in vitro and on aging-related changes in BMD in mice.
MC3T3-E1 and C3H10T1/2 cells were maintained at 37℃ in a humidified incubator supplemented with 5% CO2 in Dulbecco's modified Eagle medium (high glucose; Gibco, Waltham, MA, USA) with 10% fetal bovine serum, 100 U penicillin, and 100 µg/mL streptomycin. MC3T3-E1 preosteoblasts and C3H10T1/2 mesenchymal stem cells were treated with 10 mM β-glycerophosphate and 50 µg/mL ascorbic acid for 7 days to induce osteoblastogenesis. The cells were then treated with one of several TZDs, including lobeglitazone, rosiglitazone, or pioglitazone, for 10 to 14 days. Each treatment dose was selected based on the biological equivalence: 0.1 µM for lobeglitazone, 0.4 µM for rosiglitazone, and 1 µM for pioglitazone; a higher dose of lobeglitazone (1.0 µM) was also used.
The total RNA was isolated with an RNeasy Mini Kit (Qiagen, Valencia, CA, USA). Equal amounts of RNA were then subjected to cDNA synthesis using an iScript III cDNA synthesis kit (Bio-Rad, Hercules, CA, USA). The relative amount of mRNA was evaluated using a Vii real-time polymerase chain reaction system (Applied Biosystems, Waltham, MA, USA) and was calculated following normalization for β-actin and glyceraldehyde 3-phosphate dehydrogenase mRNA.
Cells were lysed with 0.9% NaCl and 0.2% Triton X-100 and centrifuged at 2,500 µg for 10 minutes at 4℃. The supernatant was used for measurement of protein content and alkaline phosphatase (ALP) activity. ALP enzyme activity was determined by measuring the cleavage of 10 mM p-nitrophenyl phosphate (pNPP) at 405 nM using a commercially available kit (AnaSpec, San Jose, CA, USA). Total ALP activity is expressed as pNPP ng/µg protein. The protein content of the cells was measured using Pierce BCA protein assay reagent (Thermo Scientific, Rockford, IL, USA) and bovine serum albumin was used as the standard.
Calcium deposition was evaluated by staining the cell matrix monolayer with Alizarin red. Cells were washed twice with phosphate-buffered saline, fixed in 4% paraformaldehyde for 15 minutes at room temperature, stained with 2% Alizarin red (pH 4.2) for 20 minutes at room temperature and rinsed three times with distilled water.
Nondiabetic male 6-month-old C57BL/6 mice were obtained from SLC Company (Tokyo, Japan). The animals were housed with free access to water and were maintained at a constant temperature and on a 12-hour light, 12-hour dark cycle. All animal experiments were approved by the Animal Study Committee of Seoul National University Bundang Hospital. To evaluate the effects of lobeglitazone on BMD and compare those effects with other TZDs', biologically equivalent doses of rosiglitazone (5 µg/g/day), pioglitazone (19 µg/g/day), and lobeglitazone (0.6 µg/g/day) were tested and we also evaluated the effects of higher dose of lobeglitazone (1.2 µg/g/day). Finally, the animals were grouped into five groups: (1) control (normal saline); (2) rosiglitazone (5 µg/g/day); (3) pioglitazone (19 µg/g/day); (4) low-dose lobeglitazone (0.6 µg/g/day); and (5) high-dose lobeglitazone (1.2 µg/g/day) (n=8 per group). Each experimental drug was given for 12 weeks.
BMD was determined using the PIXImus2 instrument and software version 2.10 (GE Lunar, Madison, WI, USA). Mice were anesthetized and scanned at the beginning of the experiment before the onset of rosiglitazone treatment, an intermediate time point (4 weeks), and at the time of sacrifice (12 weeks). Total body BMD (g/cm2), excluding the head and tail regions, was obtained from each scan. Femoral BMD (g/cm2) was also evaluated. The internal variation of the repeated measures of total body BMD in these mice was determined as 1.7% to 2.0%.
The results are expressed as mean±standard error of the mean (SEM). The data were analyzed using one-way analysis of variance (ANOVA) with Bonferroni's correction for multiple comparisons. In all analyses, P<0.05 was considered to be significant. The statistical analyses were performed using SPSS for Windows version 19.0 (IBM Corp., Armonk, NY, USA).
ALP activity was measured to evaluate early osteoblast differentiation. Rosiglitazone is known to suppress osteoblastogenesis and was used as a positive control [11]. As expected, rosiglitazone significantly suppressed ALP activity. However, pioglitazone and both the lower and higher doses of lobeglitazone did not (Fig. 1).
Next, the effects of rosiglitazone, pioglitazone, and the two doses of lobeglitazone on the expression of osteoblast-specific gene markers, runt-related transcription factor 2 (RUNX2) and osteocalcin (OCN), were examined in MC3T3E1 preosteoblasts and C3H10T1/2 mesenchymal stem cells. As shown in Fig. 2, rosiglitazone attenuated the mRNA expression of RUNX2 and OCN in both MC3TE1 and C3H10T1/2 cells, and pioglitazone slightly inhibited the mRNA expression of these two genes only in differentiated MC3T3E1 cells. By contrast, neither dose of lobeglitazone had significant effects on the mRNA expression levels of RUNX2 and OCN in both MC3T31E1 cells and C3H10T1/2 cells (Fig. 2).
Mineralization was evaluated using Alizarin red staining (Fig. 3). Rosiglitazone strongly inhibited and pioglitazone suppressed to a lesser degree the staining of MC3T3E1 cells. By contrast, consistent with the effects of lobeglitazone on the osteoblasts-specific gene markers mentioned above, neither dose of lobeglitazone altered the mineralization level, as shown by Alizarin red staining, in MC3T3E1 cells.
Each experimental drug (rosiglitazone [5 µg/g/day], pioglitazone [19 µg/g/day], low-dose lobeglitazone [0.6 µg/g/day], and high-dose lobeglitazone [1.2 µg/g/day]) was fed to adult male mice for 12 weeks. The BMD changes were calculated as the percentage changes in BMD from the baseline to the end of the 12-week treatment. Compared with the vehicle-treated control group, mice in the rosiglitazone-treated group provided accelerated bone loss at whole-body and femoral BMD (Fig. 4). By contrast, mice in the pioglitazone and low- and high-dose lobeglitazone groups showed no significant differences in BMD loss compared to vehicle-treated group.
We investigated the effects of lobeglitazone on osteoblastogenesis and BMD in mice. Neither dose of lobeglitazone had any significant inhibitory effects on osteoblast differentiation. The results of the in vivo experiments also showed no significant antiosteoblastic activity. Thus, that lobeglitazone administration did not accelerate the aging-related changes of BMD in mice.
TZDs are antidiabetic agents used to treat insulin resistance in patients with T2DM [4]. These drugs have proven clinical benefits for both controlling hyperglycemia and preventing several complications related to hyperglycemia [192021]. Despite the clinical benefits and efficacy, the use of the TZDs is limited because of the side effects including edema, weight gain, and increased risk of fracture [6].
PPARγ is most abundantly expressed in adipose tissue and plays a crucial role in adipocyte biology [22]. PPARγ stimulates adipocyte differentiation and modulates insulin sensitivity in tissues by altering adipokine release or fatty acid metabolism [422]. TZDs are PPARγ agonists that promote adipocyte differentiation and proliferation [9]. The two main cell types in bone are osteoblasts and osteoclasts [2324]. The active dynamics of both cells is critical to normal bone physiology, and maintaining the ideal balance between these two cells is fundamental when treating osteoporosis [25].
Osteoclasts differentiate from hematopoietic stem cells, and osteoblasts and adipocytes share the same progenitor cell type by differentiating from mesenchymal stem cells [23]. For osteoblasts and adipocytes, differentiation into one lineage occurs at the expense of the precedent cells and limits the differentiation into other lineage [9]. This relationship between osteoblast and adipocyte differentiation may explain how TZD activation of PPARγ might favor adipogenesis and suppresses osteoblast differentiation [26]. Therefore, the potentially harmful effects of TZDs on the skeleton is not surprising in some individuals, and preclinical and clinical studies have reported deleterious effects of TZDs on bone health [1012].
Rosiglitazone, the most representative TZD and which is not currently widely used because of the associated cardiovascular risk issue, is associated with decreased BMD and increased risk of fracture [1227]. However, there are some differences between TZDs. Pioglitazone, another representative TZD, shows slightly negative effects on bone but to a lesser degree compared with those of rosiglitazone [52829].
Because of the clinical benefits of TZDs, much attention has focused on developing new TZDs that retain the antihyperglycemic actions but have fewer side effects [15]. Several new TZDs with properties different from those of rosiglitazone have been introduced. The harmful skeletal effects of these other TZDs are not obvious from in vitro and in vivo experiments [1314]. Lobeglitazone is another new TZD that is synthesized by modification of rosiglitazone [3031]. Previous clinical studies using lobeglitazone have shown its potency as an antidiabetic agent and it is now used in several Asian countries [323334]. In the present study, a biologically equivalent dose of lobeglitazone did not cause significant inhibitory effects on osteoblastogenesis and BMD in mice. The higher potency compared with that of rosiglitazone or pioglitazone should allow the use of a very low-dose of lobeglitazone for controlling hyperglycemia [30]. Moreover, previous microarray analysis of lobeglitazone, rosiglitazone, and lobeglitazone also showed that lobeglitazone has some different effects on adipocyte biology [34]. Therefore, the lower dose and distinguishable properties of lobeglitazone may contribute to the bone-sparing effect of lobeglitazone.
There are several limitations in this study. We only measured BMD as bone parameters. Other parameters that can show the bone strength properties such as bone turnover markers, or histomorphometric analysis were not assessed. Moreover, 12 weeks of treatment duration might not be enough to evaluate the long term effects of lobeglitazone on BMD changes. Finally, the animal model that was used in the present experiment was not diabetic model. Therefore, further studies are needed to elucidate whether lobeglitazone impact neutrally on the skeleton of diabetes.
In conclusion, the evidence presented here from in vitro and in vivo studies indicate that lobeglitazone has no detrimental effects on osteoblast biology compared with those of rosiglitazone. These data suggest that lobeglitazone may have few or no side effects on the skeletal system.
Figures and Tables
Fig. 1
Alkaline phosphatase (ALP) activity in MC3T3E1 cells. Cells were incubated with osteogenic media for 7 days and treated with each drug for 10 to 14 days and ALP activity was measured. Values are the mean±SEM from three independent experiments performed in duplicate. Pio, pioglitazone; Rosi, rosiglitazone; Lobe, lobeglitazone. aP<0.001 vs. non-differentation; bP<0.001 compared to osteogenesis.
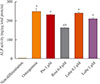
Fig. 2
mRNA expression of osteoblasts marker genes in MC3T3E1 cells (A, B) and C3H10T1/2 cells (C, D). Gene expression for osteocalcin (OCN) and runt-related transcription factor 2 (RUNX2) were evaluated by quantitative polymerase chain reaction. The expression level of each gene was normalized to that of β-actin. All data were shown as mean±SEM. Pio, pioglitazone; Rosi, rosiglitazone; Lobe, lobeglitazone. aP<0.001 vs. non-differentation; bP<0.05; and cP<0.001 compared to osteogenesis.
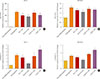
References
1. Overbeek JA, Heintjes EM, Prieto-Alhambra D, Blin P, Lassalle R, Hall GC, et al. Type 2 diabetes mellitus treatment patterns across Europe: a population-based multi-database study. Clin Ther. 2017; 39:759–770.
2. Stolar MW, Hoogwerf BJ, Gorshow SM, Boyle PJ, Wales DO. Managing type 2 diabetes: going beyond glycemic control. J Manag Care Pharm. 2008; 14:5 Suppl B. S2–S19.
3. Tran L, Zielinski A, Roach AH, Jende JA, Householder AM, Cole EE, et al. Pharmacologic treatment of type 2 diabetes: oral medications. Ann Pharmacother. 2015; 49:540–556.
4. Yki-Jarvinen H. Thiazolidinediones. N Engl J Med. 2004; 351:1106–1118.
5. Grey A, Bolland M, Fenwick S, Horne A, Gamble G, Drury PL, et al. The skeletal effects of pioglitazone in type 2 diabetes or impaired glucose tolerance: a randomized controlled trial. Eur J Endocrinol. 2013; 170:255–262.
6. Kahn BB, McGraw TE. Rosiglitazone, PPARγ, and type 2 diabetes. N Engl J Med. 2010; 363:2667–2669.
7. Ksiazek K. A comprehensive review on mesenchymal stem cell growth and senescence. Rejuvenation Res. 2009; 12:105–116.
8. Wan Y. PPARγ in bone homeostasis. Trends Endocrinol Metab. 2010; 21:722–728.
9. Patel JJ, Butters OR, Arnett TR. PPAR agonists stimulate adipogenesis at the expense of osteoblast differentiation while inhibiting osteoclast formation and activity. Cell Biochem Funct. 2014; 32:368–377.
10. Billington EO, Grey A, Bolland MJ. The effect of thiazolidinediones on bone mineral density and bone turnover: systematic review and meta-analysis. Diabetologia. 2015; 58:2238–2246.
11. Ali AA, Weinstein RS, Stewart SA, Parfitt AM, Manolagas SC, Jilka RL. Rosiglitazone causes bone loss in mice by suppressing osteoblast differentiation and bone formation. Endocrinology. 2005; 146:1226–1235.
12. Bilezikian JP, Josse RG, Eastell R, Lewiecki EM, Miller CG, Wooddell M, et al. Rosiglitazone decreases bone mineral density and increases bone turnover in postmenopausal women with type 2 diabetes mellitus. J Clin Endocrinol Metab. 2013; 98:1519–1528.
13. Fukunaga T, Zou W, Rohatgi N, Colca JR, Teitelbaum SL. An insulin-sensitizing thiazolidinedione, which minimally activates PPARγ, does not cause bone loss. J Bone Miner Res. 2015; 30:481–488.
14. Lazarenko OP, Rzonca SO, Suva LJ, Lecka-Czernik B. Netoglitazone is a PPAR-gamma ligand with selective effects on bone and fat. Bone. 2006; 38:74–84.
15. Liu C, Feng T, Zhu N, Liu P, Han X, Chen M, et al. Identification of a novel selective agonist of PPARγ with no promotion of adipogenesis and less inhibition of osteoblastogenesis. Sci Rep. 2015; 5:9530.
16. Sellmeyer DE, Civitelli R, Hofbauer LC, Khosla S, Lecka-Czernik B, Schwartz AV. Skeletal metabolism, fracture risk, and fracture outcomes in type 1 and type 2 diabetes. Diabetes. 2016; 65:1757–1766.
17. Schwartz AV. Diabetes, bone and glucose-lowering agents: clinical outcomes. Diabetologia. 2017; 60:1170–1179.
18. Bae KH, Seo JB, Jung YA, Seo HY, Kang SH, Jeon HJ, et al. Lobeglitazone, a novel peroxisome proliferator-activated receptor γ agonist, attenuates renal fibrosis caused by unilateral ureteral obstruction in mice. Endocrinol Metab (Seoul). 2017; 32:115–123.
19. Osman I, Segar L. Pioglitazone, a PPARγ agonist, attenuates PDGF-induced vascular smooth muscle cell proliferation through AMPK-dependent and AMPK-independent inhibition of mTOR/p70S6K and ERK signaling. Biochem Pharmacol. 2016; 101:54–70.
20. Kernan WN, Viscoli CM, Furie KL, Young LH, Inzucchi SE, Gorman M, et al. Pioglitazone after ischemic stroke or transient ischemic attack. N Engl J Med. 2016; 374:1321–1331.
21. Dormandy JA, Charbonnel B, Eckland DJ, Erdmann E, Massi-Benedetti M, Moules IK, et al. Secondary prevention of macrovascular events in patients with type 2 diabetes in the PROactive Study (PROspective pioglitAzone Clinical Trial In macroVascular Events): a randomized controlled trial. Lancet. 2005; 366:1279–1289.
22. Cariou B, Charbonnel B, Staels B. Thiazolidinediones and PPARγ agonists: time for a reassessment. Trends Endocrinol Metab. 2012; 23:205–215.
23. Harada S, Rodan GA. Control of osteoblast function and regulation of bone mass. Nature. 2003; 423:349–355.
24. Matsuo K, Irie N. Osteoclast-osteoblast communication. Arch Biochem Biophys. 2008; 473:201–209.
25. Henriksen K, Neutzsky-Wulff AV, Bonewald LF, Karsdal MA. Local communication on and within bone controls bone remodeling. Bone. 2009; 44:1026–1033.
26. Crossno JT Jr, Majka SM, Grazia T, Gill RG, Klemm DJ. Rosiglitazone promotes development of a novel adipocyte population from bone marrow-derived circulating progenitor cells. J Clin Invest. 2006; 116:3220–3228.
27. Berberoglu Z, Yazici AC, Demirag NG. Effects of rosiglitazone on bone mineral density and remodelling parameters in postmenopausal diabetic women: a 2-year follow-up study. Clin Endocrinol (Oxf). 2010; 73:305–312.
28. Bone HG, Lindsay R, McClung MR, Perez AT, Raanan MG, Spanheimer RG. Effects of pioglitazone on bone in postmenopausal women with impaired fasting glucose or impaired glucose tolerance: a randomized, double-blind, placebo-controlled study. J Clin Endocrinol Metab. 2013; 98:4691–4701.
29. Li R, Xu W, Luo S, Xu H, Tong G, Zeng L, et al. Effect of exenatide, insulin and pioglitazone on bone metabolism in patients with newly diagnosed type 2 diabetes. Acta Diabetol. 2015; 52:1083–1091.
30. Lee HW, Kim BY, Ahn JB, Kang SK, Lee JH, Shin JS, et al. Molecular design, synthesis, and hypoglycemic and hypolipidemic activities of novel pyrimidine derivatives having thiazolidinedione. Eur J Med Chem. 2005; 40:862–874.
31. Kim BY, Ahn JB, Lee HW, Kang SK, Lee JH, Shin JS, et al. Synthesis and biological activity of novel substituted pyridines and purines containing 2,4-thiazolidinedione. Eur J Med Chem. 2004; 39:433–447.
32. Kim SG, Kim DM, Woo JT, Jang HC, Chung CH, Ko KS, et al. Efficacy and safety of lobeglitazone monotherapy in patients with type 2 diabetes mellitus over 24-weeks: a multicenter, randomized, double-blind, parallel-group, placebo controlled trial. PLoS One. 2014; 9:e92843.
33. Kim SH, Kim SG, Kim DM, Woo JT, Jang HC, Chung CH, et al. Safety and efficacy of lobeglitazone monotherapy in patients with type 2 diabetes mellitus over 52 weeks: an open-label extension study. Diabetes Res Clin Pract. 2015; 110:e27–e30.
34. Lim S, Lee KS, Lee JE, Park HS, Kim KM, Moon JH, et al. Effect of a new PPAR-gamma agonist, lobeglitazone, on neointimal formation after balloon injury in rats and the development of atherosclerosis. Atherosclerosis. 2015; 243:107–119.