Abstract
The morbidity and mortality associated with diabetic complications impose a huge socioeconomic burden worldwide. Therefore, the ultimate goal of managing diabetes mellitus (DM) is to lower the risk of macrovascular complications and highly morbid microvascular complications such as diabetic nephropathy (DN) and diabetic retinopathy (DR). Potential benefits of incretin-based therapies such as glucagon-like peptide 1 receptor agonists (GLP-1 RAs) and dipeptidyl peptidase-4 (DPP-4) inhibitors on the diabetic macrovascular complications have been recently suggested, owing to their pleiotropic effects on multiple organ systems. However, studies primarily investigating the role of these therapies in diabetic microvascular complications are rare. Nevertheless, preclinical and limited clinical data suggest the potential protective effect of incretin-based agents against DN and DR via their anti-inflammatory, antioxidative, and antiapoptotic properties. Evidence also suggests that these incretin-dependent and independent beneficial effects are not necessarily associated with the glucose-lowering properties of GLP-1 RAs and DPP-4 inhibitors. Hence, in this review, we revisit the preclinical and clinical evidence of incretin-based therapy for DR and DN, the two most common, morbid complications in individuals with DM. In addition, the review discusses a few recent studies raising concerns of aggravating DR with the use of incretin-based therapies.
The ultimate goal of maintaining euglycemia in individuals with diabetes mellitus (DM) is to lower the risk of morbidity and mortality associated with diabetic complications. Accordingly, the UK Prospective Diabetes Study (UKPDS) reported that compared with the conventional group, the intensive group showed a significant risk reduction by 12% in the any diabetes-related aggregate endpoint, which was mainly due to a 25% risk reduction in microvascular endpoints [1]. Moreover, this intensive glycemic control led to the lower rates of cardiovascular events and diabetes-related mortality 10 years later [2]. However, in the Korean diabetic population, the prevalence of diabetic complications remains high; the prevalence of diabetic nephropathy (DN) was 30.3% in 2016, and that of diabetic retinopathy (DR) was 15.9% in 2015 [3]. Unfortunately, these numbers are not significantly decreasing despite a huge improvement in antidiabetic therapies over the last few decades. Thus, an antidiabetic medication that exerts additional protective effects against diabetic complications beyond glycemic control [4] would certainly be ideal to further reduce diabetes-related morbidity and mortality.
Incretins are a family of gut hormones that include glucose-dependent insulinotropic polypeptide and glucagon-like peptide 1 (GLP-1) [5]. Recognition of the glucoregulatory role exerted by these intestinal hormones eventually led to the recent development of novel, targeted therapies for the treatment of type 2 diabetes mellitus (T2DM) [4]. Incretin-based therapies, such as GLP-1 receptor agonists (GLP-1 RAs) and dipeptidyl peptidase-4 (DPP-4) inhibitors, which inhibit the degradation of GLP-1 by DPP-4, have been introduced into the market in the past decade to overcome the short half-life of the endogenous incretin effect [6]. A body of evidence suggests that the pleiotropic effects that these incretin-based therapies possess result in a better glycemic profile, weight loss, and favorable macrovascular and microvascular outcomes [7]. Moreover, the low risk of hypoglycemia with incretin-based drugs can reduce the concern of hypoglycemia-induced aggravation of vascular outcomes [8].
Recently, there have been multiple clinical trials to determine the efficacy and cardiovascular safety of GLP-1 RAs and DPP-4 inhibitors [91011121314]. In these trials, the impact of these agents on the microvascular complications is not well elaborated and the interpretation can be limited because the microvascular outcomes were reported as the secondary endpoint. Considering the morbidity and economic burden due to microvascular complications, more evidence is needed to evaluate the efficacy and safety of incretin-based drugs using microvascular complications as the primary endpoint. Hence, by reviewing the available, up-to-date evidence in the literature, we evaluate the effects of incretin-based therapies on DN and DR and the mechanisms through which these therapies exerted protective effects against these diabetic microvascular complications. In addition, we here address the recent issue of potential adverse effect of incretin-based therapies in DR [1015].
Fig. 1 illustrates various pathways through which incretin-based therapies can potentially exert beneficial effects on diabetic microvascular complications. Incretins induce insulin secretion and suppress glucagon secretion, which improve hyperglycemia and insulin resistance [16]. As a result, reduced oxidative stress and advanced glycation end products (AGEs) lead to vasculoprotective effects [1718]. We have previously reviewed the pleiotropic and protective effects of native GLP-1 and GLP-1 RAs on the vascular endothelium and inflammatory cells in addition to their effects on glycemic control [6]. Both the direct effect of GLP-1 on the microvasculature and the improvements in glycemic control and insulin resistance via the “incretin-dependent pathway” can contribute to the beneficial effects on microvascular complications.
In addition, a body of evidence suggests that incretin-based therapies exert anti-inflammatory, antioxidant, and antiapoptotic effects in the vasculature via incretin-independent pathways [4]. This phenomenon has been well demonstrated in studies involving DPP-4 inhibitors, since DPP-4 has actions on other substrates, in addition to its actions on incretin hormones. For example, DPP-4 exhibits protease activities on substrates such as stromal cell-derived factor 1α (SDF-1α), brain natriuretic peptide, and neuropeptide Y-1 (NPY-1); these substrates have been suggested to have regulatory effects on vascular function, such as vascular tone regulation, inflammation, cell migration, and cell differentiation [19]. Taken together, these findings suggest that these incretin-dependent and independent mechanisms of GLP-1 RAs and DPP-4 inhibitors can exert potentially beneficial effects on diabetic microangiopathies (Fig. 1).
Obesity and T2DM are associated with progressive loss of renal function that could eventually result in chronic kidney disease (CKD) and end-stage renal disease [2021]. Expression of GLP-1 receptors in both animal and human kidneys has been identified, especially in the glomeruli and proximal tubules [222324]. Moreover, the increased renal DPP-4 activity in the overnutrition/obese state [25] and the downregulated GLP-1 receptor expression in the glomeruli in a diabetic rat model [26] indicate the potential benefits of incretin-based therapies against DN.
In fact, accumulated evidence from preclinical studies suggests that GLP-1 RAs exert renoprotective effects via numerous mechanisms. Mima et al. [27] demonstrated that exendin-4, a GLP-1 RA, mediates its renoprotective action in the glomerular endothelial cells by inhibiting angiopoietin-2 signaling and suppressing downstream inflammatory reactions, as supported by decreased mRNA levels of inflammatory markers such as plasminogen activator inhibitor 1 (PAI-1) in the renal cortex of diabetic rodents. Similarly, Ishibashi et al. [28] observed that GLP-1 inhibited receptor for AGE (RAGE) gene expression in mesangial cells, further reducing reactive oxygen species generation, and the gene and protein expression of monocyte chemoattractant protein-1 (MCP-1) in AGE-exposed mesangial cells. In addition, in a study using a streptozotocin (STZ)-induced rat model of type 1 diabetes mellitus (T1DM), a 4-week administration of another GLP-1 RA, liraglutide, reversed the abnormal elevation of the oxidative stress markers, NAD(P)H (nicotinamide adenine dinucleotide phosphate) oxidase, transforming growth factor β (TGF-β), fibronectin, and urinary albumin excretion, all of which were induced by STZ treatment [29]. Interestingly, these phenomena were observed with unchanged plasma glucose levels and body weights [29]. Taken together, these studies suggest that GLP-1 directly exerts renoprotective effects in both glomerular endothelial cells and mesangial cells in addition to glycemic control, by minimizing oxidative stress and inflammation.
Clinical evidence of renoprotective effects of GLP-1 RAs, albeit limited, exists. In a study of 31 T2DM patients, subjects were randomized to a GLP-1 RA arm (exenatide) or a sulfonylurea arm (glimepiride) [30]. After 16 weeks of treatment, 24-hour urinary albumin, urinary TGF-β1, and type IV collagen were significantly lower in the exenatide group than in the glimepiride group, with no significant difference in the glycemic control between the groups [30]. These findings suggest a direct benefit of exenatide in individuals with DN via anti-inflammatory and possibly antifibrotic mechanisms, independent of its glucose-lowering effect. In addition, GLP-1 injection to obese individuals with insulin resistance, who were overtly healthy, induced natriuresis without altering potassium excretion [31]. von Scholten et al. [32] observed antihypertensive effects as well as reversible reductions in albuminuria with a short regimen of liraglutide treatment (i.e., 7 weeks) in 31 participants with T2DM. In the latter study, similar results were reported when the investigators performed a 1-year extension study [33], indicating persistent hemodynamic improvement induced by GLP-1 RAs by diminishing volume expansion and glomerular hyperfiltration.
In the Liraglutide Effect and Action in Diabetes: Evaluation of Cardiovascular Outcome Results (LEADER) trial, which observed favorable cardiovascular outcomes with the use of liraglutide, the risk of nephropathy was investigated as the secondary endpoint [11]. In this study, a long-term (i.e., 42 to 60 months) treatment with liraglutide also resulted in a reduced risk of DN (hazard ratio [HR], 0.78; 95% confidence interval [CI], 0.67 to 0.92; P=0.003), defined as the development of new onset macroalbuminuria, a doubling of the serum creatinine level and an estimated glomerular filtration rate (eGFR) of ≤45 mL/min/1.73 m2, the need for continuous renal-replacement therapy, or death from renal disease [11]. In the Trial to Evaluate Cardiovascular and Other Long-term Outcomes with Semaglutide in Subjects with Type 2 Diabetes (SUSTAIN-6), the risk of new or worsening nephropathy was also observed as the secondary outcome, which included persistent macroalbuminuria, persistent doubling of the serum creatinine level and an eGFR of ≤45 mL/min/1.73 m2, or the need for continuous renal-replacement therapy [10]. In this study, the HR of new or worsening DN was 0.64 (95% CI, 0.46 to 0.88; P=0.005), further emphasizing the renoprotective roles of GLP-1 RAs in DN. Results from upcoming large-sized studies, such as the Exenatide Study of Cardiovascular Event Lowering (EXSCEL) trial of exenatide or the Researching CV Events with a Weekly Incretin in Diabetes (REWIND) trial of dulaglutide, could clarify whether these renoprotective results are a class effect.
In a rat model of T1DM, administration of DPP-4 inhibitors for 8 weeks resulted in an increased serum concentration of active GLP-1, a decreased urinary albumin excretion, and an ameliorated DN histology [34]. DPP-4 inhibitors prevented macrophage infiltration, downregulated inflammatory molecules, and suppressed nuclear factor-κB (NF-κB) activity in the kidney [34]. All of these effects were observed without significant changes in glycemic profiles, suggesting that DPP-4 inhibitors exert anti-inflammatory and antifibrotic renoprotective effects by increasing the serum concentration of active GLP-1. Such renoprotective effects of commercially available DPP-4 inhibitors, including sitagliptin, linagliptin, and vildagliptin, have also been demonstrated in numerous animal studies [35363738].
However, the increased activity of NPY owing to the inhibition of DPP-4 (i.e., an incretin-independent effect) has been suggested in an animal model, which enhances the renovascular effects of angiotensin II [3940]. Therefore, it is possible that the enhanced NPY activity owing to the inhibition of the protease activity of DPP-4 ameliorate the effect of antihypertensives such as angiotensin-converting enzyme inhibitors (ACEi). This finding raises a concern, because ACEi are commonly prescribed as first-line antihypertensive agents to control blood pressure in patients with DM, who might also be using DPP-4 inhibitors. However, in the aforementioned studies, there was no evidence that the enhanced NPY activity led to aggravated DN. Moreover, inhibition of diverse substrate-specific actions following administration of DPP-4 inhibitors can lead to various downstream results (Fig. 1). Therefore, more study results will be needed to assess the overall risk-benefit of administering DPP-4 inhibitors in humans.
A few clinical studies also demonstrated the effects of DPP-4 inhibitors on the renal function of patients with DM. Sitagliptin [41], alogliptin [42], vildagliptin [43], and linagliptin [44] decreased albuminuria in patients with T2DM. Interestingly, the effect of sitagliptin in reducing albuminuria was observed 6 months after the initiation of therapy, for which the authors suggested that the lowering effect of eGFR by sitagliptin was dependent on improved dysglycemia and blood pressure [4145]. However, because the serum levels of high-sensitivity C-reactive protein and soluble vascular cell adhesion protein 1 were also reduced after 6 months of sitagliptin treatment in this study [41], it is plausible that some anti-inflammatory properties of the DPP-4 inhibitor had contributed to this effect. Fujita et al. [42] conducted a crossover study of T2DM patients who were treated with sitagliptin for 4 weeks, switched to alogliptin for 4 weeks, and then returned to sitagliptin treatment for an additional 4 weeks. Interestingly, urinary albumin and oxidative stress marker (i.e., 8-hydroxy 2'-deoxyguanosine [8-OHdG]) levels significantly decreased and the plasma SDF-1α level significantly increased during the alogliptin treatment compared to the levels during the sitagliptin treatment [42], suggesting that alogliptin exerts a stronger renoprotective effect via an incretin-independent, antioxidant mechanism. In addition, administration of linagliptin for 24 weeks reduced the albumin-creatinine ratio by 32% compared to 6% in the placebo group, without significant changes in hemoglobin A1c (HbA1c) and blood pressure [44]. Hence, it is still unclear whether DPP-4 inhibitors exert their renoprotection via direct effects, better glycemic control, or both.
Some large clinical trials included renal outcome as their secondary endpoint. For example, the Saxagliptin Assessment of Vascular Outcomes Recorded in Patients with Diabetes Mellitus-Thrombolysis in Myocardial Infarction (SAVOR-TIMI 53) trial reported a significant reduction in albuminuria, when examining the treatment difference in the number and proportions of patients with worsening, no change, or improvement in albumin/creatinine ratio, pre-specified as a shift from baseline category at 1, 2 year, and end of treatment [13].
The Efficacy, Safety and Modification of Albuminuria in Type 2 Diabetes Subjects with Renal Disease with LINAgliptin (MARLINA-T2D) study [46] was a 24-week, prospective, randomized, placebo-controlled, phase 3b clinical trial. It evaluated the renal outcomes (i.e., albuminuria and progression of CKD) as the primary endpoint in T2DM patients who had pre-study DN and were receiving current standard of care, including ACEi or angiotensin receptor blockers. In this study, linagliptin reduced the urinary albumin/creatinine ratio, but not to a statistically significant extent when adjusted for the results of the placebo group (i.e., mean change, −6.0%; 95% CI, −15.0 to 3.0; P=0.1954) [46]. There was no new case of CKD, but linagliptin did not significantly affect eGFR of the subjects [46]. The investigators of the MARLINA-T2D study proposed that their study subjects showed lower reductions in albuminuria compared to those in the SAVOR-TIMI 53 study, because the MARLINA-T2D study population contained insufficient numbers of advanced CKD patients to fully unveil the anti-albuminuric effect of linagliptin [46]. Nevertheless, consistent with the results of the aforementioned preclinical studies, these clinical trials also support the potentially renoprotective effect of DPP-4 inhibitors, although the exact mechanism is yet to be discovered.
DR is a major diabetic microvascular complication that can lead to decreased visual acuity and blindness [47]. Increased vascular permeability, edema, recruitment of inflammatory cells, elevated cytokine levels, tissue damage, and neovascularization have been observed in DR, implicating oxidative stress and inflammation as the key mechanisms [48].
Early signs in the pathogenesis of DR include retinal neurodegeneration [49] and vascular leakage due to damage to the blood-retinal barrier (BRB), which is formed by tight junction structures that link retinal vascular endothelial (VE) cells [50]. Albeit limited, evidence exists that GLP-1 RAs might reverse these early pathologic findings in DR [4951]. Hernandez et al. [49] first identified the expression of GLP-1 and GLP-1 receptors in the human retina, and observed that systemic treatment with liraglutide prevented retinal neurodegeneration in db/db mice via activation of the AKT pathway, which is essential for the survival of retinal neurons. In this study, liraglutide, native GLP-1, lixisenatide, or exenatide was also administered topically to delineate whether the protective effect of GLP-1 against DR was attributable to its glucose-lowering effect. As a result, topical administration of each of the aforementioned agents replicated the same protective effect, suggesting that GLP-1 and GLP-1 RAs exert neuroprotective effects in the retina of a diabetic animal model, regardless of the blood glucose levels and the type of GLP-1 RA [49].
Intraocular pressure-induced retinal ischemia-reperfusion injury provides a useful model of DR that is characteristic of vascular endothelial growth factor (VEGF)-driven vascular permeability followed by inflammatory response that maintains BRB loss [52]. Goncalves et al. [51] used this technique to induce BRB breakdown and inflammation, and observed that treatment with exendin-4 significantly reduced the BRB permeability, which was associated with a decreased mRNA expression of proinflammatory cytokines, including interleukin-1β (IL-1β), IL-6, tumor necrosis factor, and C-C motif chemokine ligand 2. In addition, because the intraocular pressure-induced retinal ischemia-reperfusion injury model does not alter systemic metabolic pathways, the authors concluded that the protective effect of GLP-1 against DR was attributable to its direct effect on diabetic retinas beyond glycemic control [51]. They also observed reduced inflammatory response to lipopolysaccharide and inhibited NF-κB activation with exendin-4 treatment in cultured cells [51]. Similarly, Fan et al. [53] observed that intravitreal injections of exendin-4 in a rat model protected BRB from vascular leakage via the downregulation of tight junction proteins (i.e., claudin-5 and occludin). Taken together, these findings suggest that GLP-1 and GLP-1 RAs possess a protective effect against DR by reversing and preventing early changes, such as neurodegeneration and BRB permeability, via their antiapoptotic and anti-inflammatory mechanisms.
Unlike the protective effect observed in the aforementioned preclinical studies, studies on the role of GLP-1 and GLP-1 RAs in humans are limited and inconclusive. For example, in 2011, Varadhan et al. [54] observed transient worsening of DR in 30% of diabetic subjects treated with GLP-1 RA, which was associated with a rapid reduction in HbA1c levels. This finding was rather surprising because, theoretically, incretin-based drugs, such as GLP-1 RA, were considered to have very low capacities to provoke hypoglycemia, which is a risk factor for aggravating DR as garnered from previous studies [55]. These results clearly emphasize the need for further clinical studies to evaluate the potential effectiveness of GLP-1 RA for DR.
Several recent clinical trial results evaluating cardiovascular safety have reported DR as the secondary outcome. In the LEADER study, the incidence rate of DR, defined as the need for retinal photocoagulation or treatment with intravitreal agents, vitreous hemorrhage, or the onset of diabetes-related blindness, was slightly higher in the liraglutide group (i.e., 0.6 events/100 patient-years) than in the placebo group (i.e., 0.5/100 patient-years), and the HR for DR was 1.15 (95% CI, 0.87 to 1.52), although it did not reach statistical significance (P=0.33) [11]. Because the differences in glycemic control between the two treatment groups over a long period of follow-up (a median of 3.8 years) were subtle (i.e., a mean difference, −0.40% points; 95% CI, −0.45 to −0.34), the possibility of GLP-1 RAs worsening DR independently of glycemic control cannot be ruled out, despite the lack of statistical significance.
In September 2016, SUSTAIN-6 study investigators reported the superiority of semaglutide for cardiovascular complications [10]. However, in this study, rates of DR (vitreous hemorrhage, blindness, or conditions requiring treatment with an intravitreal agent or photocoagulation) were significantly higher in the semaglutide group (HR, 1.76; 95% CI, 1.11 to 2.78; P=0.02) than in the placebo group [10]. As a response to rising concerns of the potential association of GLP-1 RAs and DR, further insights were provided by the SUSTAIN-6 investigators at the 77th scientific session of the American Diabetes Association [56]. There were 79 DR events that were adjudicated by an external event adjudication committee (EAC), 50 (3.0%) of which occurred in the semaglutide arm and 29 (1.8%) in the placebo arm [56]. Further analysis revealed that the subjects with EAC-confirmed events had a longer duration of diabetes, higher HbA1c levels, and higher percentages of insulin treatment, which suggests that those with the DR events during the study period had more progressed DM at baseline [56]. In addition, those with the retinopathy events had much higher incidences of DR medical history, proliferative diabetic retinopathy (PDR), and treatment with laser therapy or intravitreal agents due to the PDR; they also showed a much steeper, dose-dependent decline in HbA1c within the first 16 weeks, compared with that of the overall study population [56]. Subgroup analysis revealed that, among patients without a history of DR, the risk of retinopathy complications was low and comparable for both the semaglutide and placebo arms [56].
There are several noteworthy points that should be addressed before drawing any premature conclusions on the association between GLP-1 RAs and the progression of DR. First, the SUSTAIN-6 study had a shorter duration of follow-up (i.e., 2 years) compared to that of the LEADER study (i.e., 3.8 years). Hence, this shorter follow-up period could not have provided enough time to obtain a sufficient outcome using the standard methods for measuring DR progression [71011]. There also was a lack of detailed evaluation for the DR at the initiation of the trials. For example, a criterion for dilation, as well as a grading system of the retinopathy did not exist, and any study investigator, optometrist, or ophthalmologist could perform the examinations [756]. Hence, data from future clinical trials of GLP-1 RAs with DR as an outcome with more precise randomization and detailed evaluation of DR at baseline and at follow-up should be accumulated to judge whether this is a class effect. Moreover, the beneficial effect of GLP-1 on outcomes other than DR should always be considered when evaluating the risk-benefit profile of GLP-1 RAs, because favorable cardiovascular and renal outcomes can lead to overall reductions in morbidity and mortality among patients with DM.
Goncalves et al. [57] evaluated the effect of sitagliptin administration on the changes in the tight junction in a T2DM animal model (i.e., Zucker diabetic fatty rats); thereby, determining the permeability of BRB, a hallmark of DR development. In this study, 6-week treatment with sitagliptin (10 mg/kg/day) resulted in a better glycemic outcome, and prevented the changes in the glucose-mediated damage of the BRB [57]. Sitagliptin administration did not alter the total level of tight junction proteins (i.e., occludin and claudin-5), but it attenuated the intracellular accumulation of these proteins that was induced by DM [57]. This redistribution of tight junction proteins implicates junctional complex stabilization, which leads to maintenance of BRB integrity, leading to the protective effect of sitagliptin against DR [57].
In addition, Goncalves et al. [57] observed that sitagliptin administration ameliorated the decrease in the number of endothelial progenitor cells (EPCs) in a T2DM animal model. EPCs are stem cells that are indicative of the endogenous endothelial regenerative capacity and neovascularization, through which vascular protection is provided (Fig. 1) [58], and T2DM is associated with reduced circulating EPCs [59]. Collectively, findings from this preclinical study suggest that sitagliptin attenuates diabetes-related damages to the BRB by stabilizing the tight junction proteins and by enhancing vascular homeostasis through the mobilization of EPCs. This team also observed similar anti-inflammatory and antiapoptotic effects of sitagliptin in the retina of STZ-induced animal models [60], indicating that similar mechanisms might exist in the setting of T1DM. Treatment with vildagliptin in Otsuka Long-Evans Tokushima Fatty rats (OLETF rats), an animal model of obese T2DM, resulted in reduced gene expression levels of inflammatory and thrombogenic markers, such as VEGF, intercellular adhesion molecule 1, PAI-1, and pigment epithelium-derived factor, in the retina [61], showing beneficial effects of vildagliptin on retinal injury in T2DM animal models.
Recently, opposing study results were reported by Lee et al. [15]. Using various in vivo and in vitro DR models, they demonstrated that DPP-4 inhibitors increased vascular permeability through the SDF-1α/C-X-C chemokine receptor type 4 (CXCR4) axis, followed by Src activation and phosphorylation of VE-cadherin. Because these were the first data showing an association between DPP-4 inhibitors and increased vascular permeability in the retina, albeit preclinical, concerns of the safety of incretin-based drugs with DR in T2DM patients were raised.
To date, limited clinical studies exist with regard to the effect of DPP-4 inhibitors on EPCs and DR. Fadini et al. [62] showed that a 4-week administration of sitagliptin elevated circulating EPCs in T2DM human subjects already treated with metformin and/or insulin secretagogues. Moreover, this increased level of EPCs was associated with upregulation of SDF-1α [62], which is a chemokine that promotes the mobilization of EPCs from bone marrow into the circulation by binding to its receptor, CXCR4 [63]. This finding is consistent with that of the aforementioned preclinical study that observed the amelioration of decreased EPC mobilization upon sitagliptin administration in a T2DM animal model [57].
Retinal hyperperfusion is an early hemodynamic change in DR, and a clinical study to investigate the effect of saxagliptin on these early retinal microvascular changes in patients with T2DM was conducted [63]. In this double-blind, placebo-controlled, cross-over trial of 50 patients with T2DM, 6 weeks of saxagliptin treatment resulted in normalization of the retinal capillary flow and improved central hemodynamics [64].
Therefore, taken together, it is plausible that the pharmacologic inhibition of DPP-4 can exert an incretin-independent, retinoprotective effect via the upregulation of the SDF-1α/CXCR4 pathway, which in turn restores the number of EPCs, enhances vascular homeostasis, and possibly normalizes early DR-related hemodynamic changes in the retinas of human subjects with T2DM. A single, opposing preclinical study result would not outweigh the multiple clinical benefits that we have observed from the aforementioned studies to date, but the multiple limitations of these studies do warrant a careful interpretation. Nevertheless, the possibility of DR aggravation by DPP-4 inhibitors should not be overlooked; thereby, emphasizing the need for more clinical data to clarify this disparity and better understand safety outcomes that are associated with the use of DPP-4 inhibitors.
Incretin-based therapies possess potent, pleiotropic effects that are beneficial in glycemic control and in vascular protection. To date, most GLP-1 RAs and DPP-4 inhibitors have shown good efficacy and safety profiles in both preclinical and clinical studies. The beneficial effects of these incretin-based therapies, through various mechanisms that are dependent and independent of glycemic improvement, indicate additional advantages against microvascular complications beyond glycemic control. Some preclinical and clinical study results have questioned the association between incretin-based therapies and the progression of DR. Due to the limitations of these studies, such as short-term follow-up periods and an imprecise method of evaluating retinopathy, any premature conclusion should be avoided. Nevertheless, in a clinical setting, evaluation of retinopathy before and during the administration of GLP-1 RAs or DPP-4 inhibitors, as well as a slower rate of glycemic control in patients with uncontrolled hyperglycemia prior to administration of these agents might be helpful strategies, given that progression of DR was associated with the existence of retinopathy at baseline and faster HbA1c reduction. Results from upcoming trials, such as the EXSCEL trial of exenatide and the REWIND trial of dulaglutide, are critical to strengthen the potential benefits and to clarify the recently raised concerns regarding the possible association between incretin-based therapies and the progression of DR.
ACKNOWLEDGMENTS
This research was supported by Basic Science Research Program through the National Research Foundation of Korea (NRF) funded by the Ministry of Education (2015R1D1A1A01057720).
References
1. UK Prospective Diabetes Study (UKPDS) Group. Intensive blood-glucose control with sulphonylureas or insulin compared with conventional treatment and risk of complications in patients with type 2 diabetes (UKPDS 33). Lancet. 1998; 352:837–853. PMID: 9742976.
2. Holman RR, Paul SK, Bethel MA, Matthews DR, Neil HA. 10-Year follow-up of intensive glucose control in type 2 diabetes. N Engl J Med. 2008; 359:1577–1589. PMID: 18784090.


3. Korean Diabetes Association. Diabetes fact sheet in Korea 2016 [Internet]. Seoul: Korean Diabetes Association;c2011. cited 2017 Aug 7. Available from: http://www.diabetes.or.kr/temp/KDA_fact_sheet%202016.pdf.
4. Aroor AR, Sowers JR, Jia G, DeMarco VG. Pleiotropic effects of the dipeptidylpeptidase-4 inhibitors on the cardiovascular system. Am J Physiol Heart Circ Physiol. 2014; 307:H477–H492. PMID: 24929856.


5. Fava S. Glucagon-like peptide 1 and the cardiovascular system. Curr Diabetes Rev. 2014; 10:302–310. PMID: 25360712.


6. Kang YM, Jung CH. Cardiovascular effects of glucagon-like peptide-1 receptor agonists. Endocrinol Metab (Seoul). 2016; 31:258–274. PMID: 27118277.


7. Simo R, Hernandez C. GLP-1R as a target for the treatment of diabetic retinopathy: friend or foe? Diabetes. 2017; 66:1453–1460. PMID: 28533296.
8. Zoungas S, Patel A, Chalmers J, de Galan BE, Li Q, Billot L, et al. Severe hypoglycemia and risks of vascular events and death. N Engl J Med. 2010; 363:1410–1418. PMID: 20925543.


9. Green JB, Bethel MA, Armstrong PW, Buse JB, Engel SS, Garg J, et al. Effect of sitagliptin on cardiovascular outcomes in type 2 diabetes. N Engl J Med. 2015; 373:232–242. PMID: 26052984.


10. Marso SP, Bain SC, Consoli A, Eliaschewitz FG, Jodar E, Leiter LA, et al. Semaglutide and cardiovascular outcomes in patients with type 2 diabetes. N Engl J Med. 2016; 375:1834–1844. PMID: 27633186.


11. Marso SP, Daniels GH, Brown-Frandsen K, Kristensen P, Mann JF, Nauck MA, et al. Liraglutide and cardiovascular outcomes in type 2 diabetes. N Engl J Med. 2016; 375:311–322. PMID: 27295427.


12. Pfeffer MA, Claggett B, Diaz R, Dickstein K, Gerstein HC, Kober LV, et al. Lixisenatide in patients with type 2 diabetes and acute coronary syndrome. N Engl J Med. 2015; 373:2247–2257. PMID: 26630143.


13. Scirica BM, Bhatt DL, Braunwald E, Steg PG, Davidson J, Hirshberg B, et al. Saxagliptin and cardiovascular outcomes in patients with type 2 diabetes mellitus. N Engl J Med. 2013; 369:1317–1326. PMID: 23992601.


14. White WB, Cannon CP, Heller SR, Nissen SE, Bergenstal RM, Bakris GL, et al. Alogliptin after acute coronary syndrome in patients with type 2 diabetes. N Engl J Med. 2013; 369:1327–1335. PMID: 23992602.


15. Lee CS, Kim YG, Cho HJ, Park J, Jeong H, Lee SE, et al. Dipeptidyl peptidase-4 inhibitor increases vascular leakage in retina through VE-cadherin phosphorylation. Sci Rep. 2016; 6:29393. PMID: 27381080.


16. Defronzo RA. Banting lecture. From the triumvirate to the ominous octet: a new paradigm for the treatment of type 2 diabetes mellitus. Diabetes. 2009; 58:773–795. PMID: 19336687.
17. Silva Junior WS, Godoy-Matos AF, Kraemer-Aguiar LG. Dipeptidyl peptidase 4: a new link between diabetes mellitus and atherosclerosis? Biomed Res Int. 2015; 2015:816164. PMID: 26146634.
18. Yamagishi S, Fukami K, Matsui T. Crosstalk between advanced glycation end products (AGEs)-receptor RAGE axis and dipeptidyl peptidase-4-incretin system in diabetic vascular complications. Cardiovasc Diabetol. 2015; 14:2. PMID: 25582643.


19. Kawanami D, Matoba K, Sango K, Utsunomiya K. Incretin-based therapies for diabetic complications: basic mechanisms and clinical evidence. Int J Mol Sci. 2016; 17:E1223. PMID: 27483245.


20. Jung CH, Lee MJ, Kang YM, Hwang JY, Kim EH, Park JY, et al. The risk of chronic kidney disease in a metabolically healthy obese population. Kidney Int. 2015; 88:843–850. PMID: 26108064.


21. Whaley-Connell A, Bomback AS, McFarlane SI, Li S, Roberts T, Chen SC, et al. Diabetic cardiovascular disease predicts chronic kidney disease awareness in the kidney early evaluation program. Cardiorenal Med. 2011; 1:45–52. PMID: 22258465.


22. Hadjiyanni I, Siminovitch KA, Danska JS, Drucker DJ. Glucagon-like peptide-1 receptor signalling selectively regulates murine lymphocyte proliferation and maintenance of peripheral regulatory T cells. Diabetologia. 2010; 53:730–740. PMID: 20225396.


23. Hamilton A, Holscher C. Receptors for the incretin glucagon-like peptide-1 are expressed on neurons in the central nervous system. Neuroreport. 2009; 20:1161–1166. PMID: 19617854.


24. Hansen L, Deacon CF, Orskov C, Holst JJ. Glucagon-like peptide-1-(7-36)amide is transformed to glucagon-like peptide-1-(9-36)amide by dipeptidyl peptidase IV in the capillaries supplying the L cells of the porcine intestine. Endocrinology. 1999; 140:5356–5363. PMID: 10537167.
25. Hansen LB. GLP-2 and mesenteric blood flow. Dan Med J. 2013; 60:B4634. PMID: 23673268.
26. Harrison DG, Marvar PJ, Titze JM. Vascular inflammatory cells in hypertension. Front Physiol. 2012; 3:128. PMID: 22586409.


27. Mima A, Hiraoka-Yamomoto J, Li Q, Kitada M, Li C, Geraldes P, et al. Protective effects of GLP-1 on glomerular endothelium and its inhibition by PKCβ activation in diabetes. Diabetes. 2012; 61:2967–2979. PMID: 22826029.


28. Ishibashi Y, Nishino Y, Matsui T, Takeuchi M, Yamagishi S. Glucagon-like peptide-1 suppresses advanced glycation end product-induced monocyte chemoattractant protein-1 expression in mesangial cells by reducing advanced glycation end product receptor level. Metabolism. 2011; 60:1271–1277. PMID: 21388644.


29. Hendarto H, Inoguchi T, Maeda Y, Ikeda N, Zheng J, Takei R, et al. GLP-1 analog liraglutide protects against oxidative stress and albuminuria in streptozotocin-induced diabetic rats via protein kinase A-mediated inhibition of renal NAD(P)H oxidases. Metabolism. 2012; 61:1422–1434. PMID: 22554832.


30. Zhang H, Zhang X, Hu C, Lu W. Exenatide reduces urinary transforming growth factor-β1 and type IV collagen excretion in patients with type 2 diabetes and microalbuminuria. Kidney Blood Press Res. 2012; 35:483–488. PMID: 22687869.


31. Gutzwiller JP, Tschopp S, Bock A, Zehnder CE, Huber AR, Kreyenbuehl M, et al. Glucagon-like peptide 1 induces natriuresis in healthy subjects and in insulin-resistant obese men. J Clin Endocrinol Metab. 2004; 89:3055–3061. PMID: 15181098.


32. von Scholten BJ, Lajer M, Goetze JP, Persson F, Rossing P. Time course and mechanisms of the anti-hypertensive and renal effects of liraglutide treatment. Diabet Med. 2015; 32:343–352. PMID: 25251901.


33. von Scholten BJ, Hansen TW, Goetze JP, Persson F, Rossing P. Glucagon-like peptide 1 receptor agonist (GLP-1 RA): long-term effect on kidney function in patients with type 2 diabetes. J Diabetes Complications. 2015; 29:670–674. PMID: 25935863.


34. Kodera R, Shikata K, Takatsuka T, Oda K, Miyamoto S, Kajitani N, et al. Dipeptidyl peptidase-4 inhibitor ameliorates early renal injury through its anti-inflammatory action in a rat model of type 1 diabetes. Biochem Biophys Res Commun. 2014; 443:828–833. PMID: 24342619.


35. Liu WJ, Xie SH, Liu YN, Kim W, Jin HY, Park SK, et al. Dipeptidyl peptidase IV inhibitor attenuates kidney injury in streptozotocin-induced diabetic rats. J Pharmacol Exp Ther. 2012; 340:248–255. PMID: 22025647.


36. Marques C, Mega C, Goncalves A, Rodrigues-Santos P, Teixeira-Lemos E, Teixeira F, et al. Sitagliptin prevents inflammation and apoptotic cell death in the kidney of type 2 diabetic animals. Mediators Inflamm. 2014; 2014:538737. PMID: 24817793.


37. Nakashima S, Matsui T, Takeuchi M, Yamagishi SI. Linagliptin blocks renal damage in type 1 diabetic rats by suppressing advanced glycation end products-receptor axis. Horm Metab Res. 2014; 46:717–721. PMID: 24710699.


38. Vavrinec P, Henning RH, Landheer SW, Wang Y, Deelman LE, Dokkum RP, et al. Vildagliptin restores renal myogenic function and attenuates renal sclerosis independently of effects on blood glucose or proteinuria in Zucker diabetic fatty rat. Curr Vasc Pharmacol. 2014; 12:836–844. PMID: 24066937.


39. Jackson EK, Dubinion JH, Mi Z. Effects of dipeptidyl peptidase IV inhibition on arterial blood pressure. Clin Exp Pharmacol Physiol. 2008; 35:29–34. PMID: 18047624.


40. Tofovic DS, Bilan VP, Jackson EK. Sitagliptin augments angiotensin II-induced renal vasoconstriction in kidneys from rats with metabolic syndrome. Clin Exp Pharmacol Physiol. 2010; 37:689–691. PMID: 20374254.


41. Hattori S. Sitagliptin reduces albuminuria in patients with type 2 diabetes. Endocr J. 2011; 58:69–73. PMID: 21206136.
42. Fujita H, Taniai H, Murayama H, Ohshiro H, Hayashi H, Sato S, et al. DPP-4 inhibition with alogliptin on top of angiotensin II type 1 receptor blockade ameliorates albuminuria via up-regulation of SDF-1α in type 2 diabetic patients with incipient nephropathy. Endocr J. 2014; 61:159–166. PMID: 24225429.
43. Tani S, Nagao K, Hirayama A. Association between urinary albumin excretion and low-density lipoprotein heterogeneity following treatment of type 2 diabetes patients with the dipeptidyl peptidase-4 inhibitor, vildagliptin: a pilot study. Am J Cardiovasc Drugs. 2013; 13:443–450. PMID: 23990203.


44. Groop PH, Cooper ME, Perkovic V, Emser A, Woerle HJ, von Eynatten M. Linagliptin lowers albuminuria on top of recommended standard treatment in patients with type 2 diabetes and renal dysfunction. Diabetes Care. 2013; 36:3460–3468. PMID: 24026560.


45. Avogaro A, Fadini GP. The effects of dipeptidyl peptidase-4 inhibition on microvascular diabetes complications. Diabetes Care. 2014; 37:2884–2894. PMID: 25249673.


46. Groop PH, Cooper ME, Perkovic V, Hocher B, Kanasaki K, Haneda M, et al. Linagliptin and its effects on hyperglycaemia and albuminuria in patients with type 2 diabetes and renal dysfunction: the randomized MARLINA-T2 trial. Diabetes Obes Metab. 2017; 6. 21. DOI: 10.1111/dom.13041. [Epub].
47. Klein R, Klein BE, Moss SE. Epidemiology of proliferative diabetic retinopathy. Diabetes Care. 1992; 15:1875–1891. PMID: 1464243.


48. Antonetti DA, Barber AJ, Bronson SK, Freeman WM, Gardner TW, Jefferson LS, et al. Diabetic retinopathy: seeing beyond glucose-induced microvascular disease. Diabetes. 2006; 55:2401–2411. PMID: 16936187.
49. Hernandez C, Bogdanov P, Corraliza L, Garcia-Ramirez M, Sola-Adell C, Arranz JA, et al. Topical administration of GLP-1 receptor agonists prevents retinal neurodegeneration in experimental diabetes. Diabetes. 2016; 65:172–187. PMID: 26384381.
50. Cunha-Vaz J, Faria de Abreu JR, Campos AJ. Early breakdown of the blood-retinal barrier in diabetes. Br J Ophthalmol. 1975; 59:649–656. PMID: 1203221.


51. Goncalves A, Lin CM, Muthusamy A, Fontes-Ribeiro C, Ambrosio AF, Abcouwer SF, et al. Protective effect of a GLP-1 analog on ischemia-reperfusion induced blood-retinal barrier breakdown and inflammation. Invest Ophthalmol Vis Sci. 2016; 57:2584–2592. PMID: 27163772.
52. Abcouwer SF, Lin CM, Wolpert EB, Shanmugam S, Schaefer EW, Freeman WM, et al. Effects of ischemic preconditioning and bevacizumab on apoptosis and vascular permeability following retinal ischemia-reperfusion injury. Invest Ophthalmol Vis Sci. 2010; 51:5920–5933. PMID: 20554620.


53. Fan Y, Liu K, Wang Q, Ruan Y, Ye W, Zhang Y. Exendin-4 alleviates retinal vascular leakage by protecting the blood-retinal barrier and reducing retinal vascular permeability in diabetic Goto-Kakizaki rats. Exp Eye Res. 2014; 127:104–116. PMID: 24910901.


54. Varadhan L, Humphreys T, Hariman C, Walker AB, Varughese GI. GLP-1 agonist treatment: implications for diabetic retinopathy screening. Diabetes Res Clin Pract. 2011; 94:e68–e71. PMID: 21906831.


55. The Diabetes Control and Complications Trial Research Group. Early worsening of diabetic retinopathy in the Diabetes Control and Complications Trial. Arch Ophthalmol. 1998; 116:874–886. PMID: 9682700.
56. Vilsboll T. Cardiovascular outcomes with semaglutide in subjects with type 2 diabetes mellitus (SUSTAIN 6) (presentation 1-AC-SY09). Paper presented at: 77th Scientific Sessions, American Diabetes Association 2017. 2017 Jun 9-13; San Diego, CA.
57. Goncalves A, Leal E, Paiva A, Teixeira Lemos E, Teixeira F, Ribeiro CF, et al. Protective effects of the dipeptidyl peptidase IV inhibitor sitagliptin in the blood-retinal barrier in a type 2 diabetes animal model. Diabetes Obes Metab. 2012; 14:454–463. PMID: 22151893.
58. Fadini GP, Agostini C, Sartore S, Avogaro A. Endothelial progenitor cells in the natural history of atherosclerosis. Atherosclerosis. 2007; 194:46–54. PMID: 17493626.


59. Fadini GP, Sartore S, Albiero M, Baesso I, Murphy E, Menegolo M, et al. Number and function of endothelial progenitor cells as a marker of severity for diabetic vasculopathy. Arterioscler Thromb Vasc Biol. 2006; 26:2140–2146. PMID: 16857948.


60. Goncalves A, Marques C, Leal E, Ribeiro CF, Reis F, Ambrosio AF, et al. Dipeptidyl peptidase-IV inhibition prevents blood-retinal barrier breakdown, inflammation and neuronal cell death in the retina of type 1 diabetic rats. Biochim Biophys Acta. 2014; 1842:1454–1463. PMID: 24769045.
61. Maeda S, Yamagishi S, Matsui T, Nakashima S, Ojima A, Maeda S, et al. Beneficial effects of vildagliptin on retinal injury in obese type 2 diabetic rats. Ophthalmic Res. 2013; 50:221–226. PMID: 24081217.


62. Fadini GP, Boscaro E, Albiero M, Menegazzo L, Frison V, de Kreutzenberg S, et al. The oral dipeptidyl peptidase-4 inhibitor sitagliptin increases circulating endothelial progenitor cells in patients with type 2 diabetes: possible role of stromal-derived factor-1alpha. Diabetes Care. 2010; 33:1607–1609. PMID: 20357375.
63. Petit I, Jin D, Rafii S. The SDF-1-CXCR4 signaling pathway: a molecular hub modulating neo-angiogenesis. Trends Immunol. 2007; 28:299–307. PMID: 17560169.


64. Ott C, Raff U, Schmidt S, Kistner I, Friedrich S, Bramlage P, et al. Effects of saxagliptin on early microvascular changes in patients with type 2 diabetes. Cardiovasc Diabetol. 2014; 13:19. PMID: 24423149.


Fig. 1
Schematic representation of the pleiotropic effect of incretin-based drugs with respect to chronic diabetic vascular complications. Modified from Aroor et al. [4], with permission from American Physiological Society. GLP-1 RA, glucagon-like peptide-1 receptor agonist; GLP-1R, glucagon-like peptide-1 receptor; GIP, gastric inhibitory peptide; AGE, advanced glycation end product; DPP-4, dipeptidyl peptidase-4; SDF-1α, stromal cell-derived factor 1α; EPC, endothelial progenitor cell; BNP, brain natriuretic peptide; NPY-1, neuropeptide Y-1; IL, interleukin.
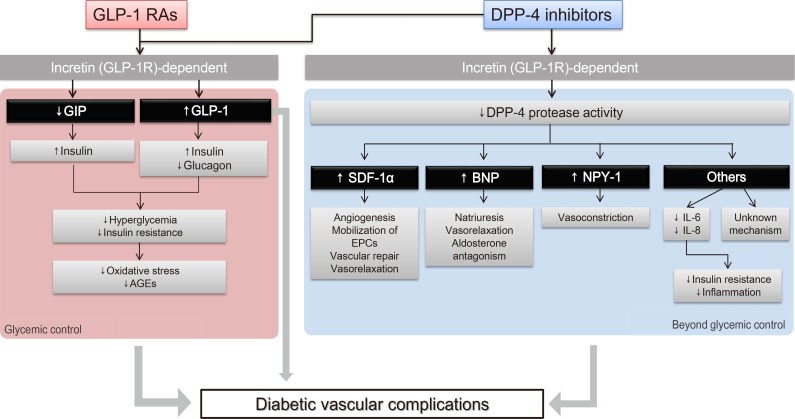