Abstract
Background
Thyrotoxic periodic paralysis (TPP) is a rare complication of thyrotoxicosis characterized by acute attacks of muscle weakness and hypokalemia. Recently, variation in several genes was suggested to be associated with TPP. This study evaluated the genetic predisposition to TPP in terms of the β2-adrenergic receptor (ADRB2), androgen receptor (AR), and γ-aminobutyric acid receptor α3 subunit (GABRA3) genes.
Methods
This study enrolled 48 men with Graves disease (GD) and TPP, and 48 GD patients without TPP. We compared the frequencies of candidate polymorphisms between the two groups.
Results
The frequency of the Gly16/Gly16 genotype in ADRB2 was not significantly associated with TPP (P=0.32). More CAG repeats (≥26) in the AR gene were not correlated with TPP (odds ratio [OR], 2.46; 95% confidence interval [CI], 0.81 to 8.09; P=0.08). The allele frequency of the TT genotype in the GABRA3 gene was not associated with TPP (OR, 1.83; 95% CI, 0.54 to 6.74; P=0.41).
Thyrotoxic periodic paralysis (TPP) is a rare complication of thyrotoxicosis characterized by acute, reversible episodes of muscle weakness and low serum potassium levels [1]. TPP is prevalent in Asian populations, particularly among Chinese, Japanese, Vietnamese, Filipino, and Korean nationals [23]. Although the pathogenesis of TPP remains unclear, the recurrent paralytic muscle weakness is caused by hypokalemia followed by an intracellular potassium shift [3]. The clinical features of and factors precipitating TPP are similar to those of familial hypokalemic periodic paralysis (FHPP), which is an autosomal dominant trait that is common in Caucasians, although hyperthyroidism is not present [2].
Several genetic variants have been studied in patients with and without TPP. Mutations in the skeletal muscle calcium channel (CACNA1S) [456], sodium channel (SCN4A) [567], and voltage-gated potassium channel (KCNE3) genes [57] were found in patients with FHPP. The similar clinical manifestations of TPP and FHPP, and racial predilection, suggested a strong genetic predisposition in TPP. However, in TPP patients there were no mutations in the CACNA1S and SCN4A genes and only one patient (a 44-year-old Portuguese) was reported to have a KCNE3 mutation [8]. Recently, a genome-wide association study (GWAS) of a Thai group suggested that a single nucleotide polymorphism (SNP; rs750841) in intron 3 of the γ-aminobutyric acid receptor α3 subunit (GABRA3) gene was associated with TPP [9]. The GABRA3 gene is one of the chloride channel genes.
Recently, we evaluated the Arg16Gly polymorphism in the β2-adrenergic receptor (ADRB2) gene and the number of CAG repeats in the androgen receptor (AR) gene in a small number of patients with or without TPP [1011]. The genetic alterations in these genes were not associated with the genetic susceptibility to TPP in Korean males with Graves disease (GD).
In this study, we evaluated genetic differences in the ADRB2, AR, and GABRA3 genes in a larger case-control series of Korean males with GD.
We enrolled 48 male GD patients with TPP and 48 more without TPP between 2001 and 2006 at Asan Medical Center, Seoul, Korea. All of the subjects had been diagnosed with GD based on clinical and laboratory criteria, including suppressed serum thyroid stimulating hormone (TSH) levels and increased serum free thyroxine (T4) levels, a diffuse goiter, and positive for at least one antithyroid antibody. In the subjects, TPP was confirmed using acute-onset muscle weakness and biochemical hypokalemia (<3.5 mmol/L). We obtained informed consent from all patients, and the study was approved by the Institutional Review Board of Asan Medical Center.
Genomic DNA was extracted from peripheral blood samples using the Puregene DNA Isolation Kit (Gentra Systems, Minneapolis, MN, USA). To evaluate the frequencies of the three genetic variants, genes were amplified by polymerase chain reaction (PCR) using the primers 5' GCACCCGACAAGCTGA 3' (F-ADRB2), 5' TCCAAAACTCGCACCA 3' (R-ADRB2; J2960), 5'-ACCGAGGAGCTTTCCAGAAT-3' (F-AR), 5'-CTCATCCAGGAC CAGGTAGC-3' (R-AR; AL049564), 5' CACTGCCTGTTTCCCAAAAT 3' (F-GABRA3), and 5' TGGAATGCTGAGAGTGATGG 3' (R-GABRA3; NT 011726.13).
Each reaction was performed with 100 ng of genomic DNA, 0.5 units of Taq polymerase (Takara Shuzo, Otsu, Japan), 2 pmol of each primer, and 4 nmol of each deoxynucleotide in a total reaction volume of 20 µL using the following cycles: denaturation at 94℃ for 5 minutes, followed by 42 cycles of 45 seconds at 94℃, 45 seconds at 56℃, and 45 seconds at 72℃, with a final 10-minute extension at 72℃. The PCR products were subject to agarose gel electrophoresis and DNA was extracted using a gel extraction kit (QIAGEN, Hilden, Germany). The extracted DNA was sequenced using an automated DNA sequence analyzer (PRISM 373 A, Applied Biosystems, Foster City, CA, USA) via a PCR reaction.
As previously described, the serum levels of thyroid hormone (free T4) and TSH were measured with commercial radioimmunoassay (Abbott, North Chicago, IL, USA) and immunoradiometric assay (SPAC-5 TSH kit, Daiichi, Tokyo, Japan) kits. TBII activity was measured with a radioreceptor assay kit (R.S.R., Cardiff, UK) and anti-thyroglobulin (anti-Tg) and anti-thyroperoxidase (anti-TPO) antibodies were measured with a radioligand assay (Henning test anti-Tg or anti-TPO, Diagnostica, Wiesbaden, Germany) [12]. The serum potassium level was measured using an ion-specific electrode, and all patients with TPP had hypokalemia (<3.5 mmol/L).
Associations between variables were analyzed using contingency tables and Fisher exact test. For multiple comparisons of genotype frequencies among the patients with or without TPP, the corrected P value was calculated by multiplying the P value by the number of comparisons. The Hardy-Weinberg proportion was tested using free software available at http://www2.biology.ualberta.ca/jbrzusto/hwenj.html, which was based on a report by Guo and Thompson [13]. The odds ratio (OR) and 95% confidence interval (CI) were calculated using the modified method of Woolf and Haldane. P<0.05 was considered significant.
The mean age of the 96 men with GD was 35.07±10.9 years. Their mean serum TSH and free T4 levels were 0.023±0.13 µU/mL and 4.03±1.56 ng/dL, respectively. There was a significant (P=0.01) difference in mean age between the patients with and without TPP (Table 1). Although the TPP patients were younger than those without TPP, age does not affect DNA information. There was no significant difference in the serum TSH and free T4 levels between the two groups (P=0.25 and P=0.32, respectively). Five patients with TPP had a family history of thyroid disease versus six in the group without TPP. There was no history of TPP in a family member in either group.
The genotype frequencies of the Arg16Gly polymorphism in the ADRB2 gene were compared between patients with and without TPP. The Arg16/Arg16 genotype was found in 18 of 48 patients with TPP (38%) and 19 of 48 patients without TPP (40%) (Table 2). The Gly16/Gly16 genotype was identified in 7 of 48 patients with TPP (14%) and 12 of 48 patients without TPP (25%). There were no significant differences in the genotype of the Arg16Gly polymorphism between patients with and without TPP (P=0.32). The allele frequency did not differ (OR, 1.18; 95% CI, 0.64 to 2.2; P=0.65).
"Long AR" was defined as at least 26 CAG repeats in the promoter region of the AR gene, as previously described [11]. Long AR was found in 14 of 47 patients with TPP (30%) and 7 of 48 patients without TPP (15%) (Table 3). The number of CAG repeats in the AR gene was not associated with TPP (OR, 2.46; 95% CI, 0.81 to 8.09; P=0.08).
The SNP located in intron 3 of GABRA3 gene was evaluated in the two groups (Table 4). One study reported that allele A was significantly associated with TPP cases [9]. However, 6 of 48 patients (13%) with TPP and 10 of 48 patients without TPP had allele A in rs750841 (Table 3). Therefore, there was no significant association between this polymorphism and TPP (OR, 1.83; 95% CI, 0.54 to 6.74; P=0.41).
In this study, we evaluated the associations of TPP with genetic variants in the ADRB2, AR, and GABRA3 genes in men with GD. Recently, a GWAS of a Thai group suggested that a genetic variant in the GABRA3 gene was related to TPP [9]. The SNP is rs750841, which is located in intron 3 between exons 3 and 4 of GABRA3. GABRA3 encodes the GABA receptor α3 subunit, which is a member of the chloride channel family. That study found that allele A was significantly associated with TPP and identified putative thyroid hormone response elements. As it is located on the X chromosome, it might explain the sexual predominance in TPP. Therefore, we evaluated this polymorphism in our study, but found no significant association with TPP in Koreans with GD. Moreover, the allele frequency trend was also different. This may reflect ethnic differences.
The ADRB2 gene encodes a type of ADRB2 in skeletal muscle, and thyroid hormone sensitizes cells to β-adrenergic stimulation of the sodium pump [1415]. Many studies have reported that an ADRB2 gene polymorphism was associated with regulation of adrenergic activity. Several SNPs (-47T/C, Arg16Gly, and Gln27Glu) have been reported to be associated with increased ADRB2 expression. Although the 47T/C and Gln27Glu polymorphisms are rare in Asian populations [16], the Gly16 ADRB2 variant plays a role in endogenous agonist-induced downregulation [17]. It has been hypothesized that the Gly16/Gly16 allele in ADRB2 is associated with TPP development, but that study was limited due to the small number of patients [10]. In this study, we evaluated the ADRB2 gene allele frequencies in a relatively large cohort and found no significant correlation, consistent with our previous data [1011].
Patients with TPP tend to experience symptoms after heavy carbohydrate meals [1]. This suggests that hyperinsulinemia enhances the sodium-potassium pump activity [3]. The number of polymorphic CAG repeats in the AR gene is independently and positively associated with the serum insulin concentration [18]. Furthermore, there are ethnic differences in the number of CAG repeats of the AR gene. The mean number of CAG repeats is 21 to 22 in Caucasians and 22 to 23 in East Asians [19]. We thought that this ethnic difference could explain the different ethnic prevalence of TPP. However, having more CAG repeats in the AR gene was not associated with TPP.
This study had several limitations. The number of subjects limited the ability to provide statistical power to explain the genetic susceptibility of TPP in patients with GD. Moreover, it is difficult to generalize to the general population because this is a single-center study that included only Korean men with GD. However, our study is a relatively large cohort study that evaluated several candidate genes in patients with or without TPP who had confirmed GD. We were also limited because of the candidate gene approach used to evaluate the genetic predisposition to TPP. Further studies that evaluate the entire genomes of patients with or without TPP could provide definite evidence about the pathogenesis of TPP in patients with GD.
In conclusion, we evaluated genetic variants in the ADRB2, AR, and GABRA3 genes in patients with or without TPP. These genetic variants could not explain the genetic susceptibility to TPP in Korean men with GD.
Figures and Tables
Table 1
Baseline Clinical Characteristics of the Men with Graves Disease according to the Presence of TPP
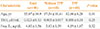
Table 2
Polymorphism in the β2-Adrenergic Receptor (ADRB2) Gene between Graves Disease Patients with and without TPP
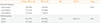
Table 3
The Number of CAG Repeats (≥26 Repeats) in the Androgen Receptor (AR) Gene in Graves Disease Patients with and without TPP
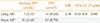
Without TPP (n=48) | TPP (n=47) | OR | 95% CI | P value | |
---|---|---|---|---|---|
Long ARa | 7 (0.15) | 14 (0.30) | 2.46 | 0.81–8.0 | 0.08 |
Short ARb | 41 (0.85) | 33 (0.70) |
Table 4
Allele Frequency of the Single Nucleotide Polymorphism (rs750841) in the γ-Aminobutyric Acid Receptor α3 Subunit (GABRA3) Gene in Graves Disease Patients with and without TPP
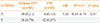
rs750841 | Without TPP (n=48) | TPP (n=48) | OR | 95% CI | P value |
---|---|---|---|---|---|
A | 10 (0.21) | 6 (0.13) | 1.83 | 0.54–6.74 | 0.41 |
T | 38 (0.79) | 42 (0.87) |
ACKNOWLEDGMENTS
This study was supported by a grant (2014-374) from the Asan Institute for Life Sciences, Seoul, Korea.
References
1. Maciel RM, Lindsey SC, Dias da Silva MR. Novel etiopathophysiological aspects of thyrotoxic periodic paralysis. Nat Rev Endocrinol. 2011; 7:657–667.
2. Kung AW. Clinical review. Thyrotoxic periodic paralysis: a diagnostic challenge. J Clin Endocrinol Metab. 2006; 91:2490–2495.
3. Ober KP. Thyrotoxic periodic paralysis in the United States. Report of 7 cases and review of the literature. Medicine (Baltimore). 1992; 71:109–120.
4. Chen L, Lang D, Ran XW, Joncourt F, Gallati S, Burgunder JM. Clinical and molecular analysis of chinese patients with thyrotoxic periodic paralysis. Eur Neurol. 2003; 49:227–230.
5. Phakdeekitcharoen B, Ruangraksa C, Radinahamed P. Hypokalaemia and paralysis in the Thai population. Nephrol Dial Transplant. 2004; 19:2013–2018.
6. Ng WY, Lui KF, Thai AC, Cheah JS. Absence of ion channels CACN1AS and SCN4A mutations in thyrotoxic hypokalemic periodic paralysis. Thyroid. 2004; 14:187–190.
7. Kung AW, Lau KS, Fong GC, Chan V. Association of novel single nucleotide polymorphisms in the calcium channel alpha 1 subunit gene (Ca(v)1.1) and thyrotoxic periodic paralysis. J Clin Endocrinol Metab. 2004; 89:1340–1345.
8. Dias Da Silva MR, Cerutti JM, Arnaldi LA, Maciel RM. A mutation in the KCNE3 potassium channel gene is associated with susceptibility to thyrotoxic hypokalemic periodic paralysis. J Clin Endocrinol Metab. 2002; 87:4881–4884.
9. Jongjaroenprasert W, Chanprasertyotin S, Butadej S, Nakasatien S, Charatcharoenwitthaya N, Himathongkam T, et al. Association of genetic variants in GABRA3 gene and thyrotoxic hypokalaemic periodic paralysis in Thai population. Clin Endocrinol (Oxf). 2008; 68:646–651.
10. Kim TY, Song JY, Kim WB, Shong YK. Arg16Gly polymorphism in beta2-adrenergic receptor gene is not associated with thyrotoxic periodic paralysis in Korean male patients with Graves’ disease. Clin Endocrinol (Oxf). 2005; 62:585–589.
11. Kim WG, Kim TY, Kim JM, Rhee YS, Choi HJ, Kim WB, et al. CAG repeats in the androgen receptor polymorphism do not correlate with thyrotoxic periodic paralysis. J Korean Endocr Soc. 2008; 23:117–122.
12. Kim WB, Han SM, Kim TY, Nam-Goong IS, Gong G, Lee HK, et al. Ultrasonographic screening for detection of thyroid cancer in patients with Graves’ disease. Clin Endocrinol (Oxf). 2004; 60:719–725.
13. Guo SW, Thompson EA. Performing the exact test of Hardy-Weinberg proportion for multiple alleles. Biometrics. 1992; 48:361–372.
14. Chan A, Shinde R, Chow CC, Cockram CS, Swaminathan R. In vivo and in vitro sodium pump activity in subjects with thyrotoxic periodic paralysis. BMJ. 1991; 303:1096–1099.
15. Marx A, Ruppersberg JP, Pietrzyk C, Rudel R. Thyrotoxic periodic paralysis and the sodium/potassium pump. Muscle Nerve. 1989; 12:810–815.
16. Green SA, Turki J, Innis M, Liggett SB. Amino-terminal polymorphisms of the human beta 2-adrenergic receptor impart distinct agonist-promoted regulatory properties. Biochemistry. 1994; 33:9414–9419.
17. Leineweber K, Brodde OE. Beta2-adrenoceptor polymorphisms: relation between in vitro and in vivo phenotypes. Life Sci. 2004; 74:2803–2814.
18. Zitzmann M, Gromoll J, von Eckardstein A, Nieschlag E. The CAG repeat polymorphism in the androgen receptor gene modulates body fat mass and serum concentrations of leptin and insulin in men. Diabetologia. 2003; 46:31–39.
19. Zitzmann M, Nieschlag E. The CAG repeat polymorphism within the androgen receptor gene and maleness. Int J Androl. 2003; 26:76–83.