Abstract
Background
Adult neural stem cells have the potential for self-renewal and differentiation into multiple cell lineages via symmetric or asymmetric cell division. Preso1 is a recently identified protein involved in the formation of dendritic spines and the promotion of axonal growth in developing neurons. Preso1 can also bind to cell polarity proteins, suggesting a potential role for Preso1 in asymmetric cell division.
Methods
To investigate the distribution of Preso1, we performed immunohistochemistry with adult mouse brain slice. Also, polarized distribution of Preso1 was assessed by immunocytochemistry in cultured neural stem cells.
Results
Immunoreactivity for Preso1 (Preso1-IR) was strong in the rostral migratory stream and subventricular zone, where proliferating transit-amplifying cells and neuroblasts are prevalent. In cultured neural stem cells, Preso1-IR was unequally distributed in the cell cytosol. We also observed the distribution of Preso1 in the subgranular zone of the hippocampal dentate gyrus, another neurogenic region in the adult brain. Interestingly, Preso1-IR was transiently observed in the nuclei of doublecortin-expressing neuroblasts immediately after asymmetric cell division.
Preso1 (also known as Frmpd4) is a member of the Frmpd family of proteins. It contains several functional domains including 4.1, ezrin, radixin, and moesin (FERM), WW (domain with two conserved Trp residues), and postsynaptic density (PSD) 95/Dig/ZO-1 (PDZ) [1]. In particular, the FERM domain acts as a molecular linker between the membrane and cytoplasm and is involved in control of cell actin dynamics. In addition, the PDZ domain, a well-known protein interaction module, is incorporated into protein complexes such as postsynaptic density (PSD) in many proteins.
Preso appears to promote the formation of dendritic spine in neurons [1]. Preso1 interacts with one of the guanine nucleotide exchange factors, PAK-interacting exchange factor-β, through its PDZ domain to promote dendritic spine formation in neurons. In addition, Preso1 is distributed at the tip of growing dendrites in immature neurons and plays an important role in dendritic branch formation [2]. Inhibition of Preso1 activity in vivo causes defects in synaptic plasticity and pain perception, suggesting the importance of Preso1 in neuronal functions [3].
Recently, Preso1 has also been shown to bind to cell polarization proteins such as Leu-Gly-Asn repeat-enriched protein (LGN) and activator of G-protein signaling 3 [4]. Unequal distribution of these proteins within a cell provides the basis for asymmetric cell division and differentiation, which are essential features of stem cells. The actin cytoskeleton serves as a platform for molecular networks of protein cargo and myosin motors, which are required for unequal protein distribution.
Here, we demonstrated that immunoreactivity for Preso1 (Preso1-IR) was predominantly expressed in neurogenic areas such as the subventricular zone (SVZ) of the lateral ventricle and the subgranular zone (SGZ) of the dentate gyrus (DG) in the adult mouse brain. In particular, Preso1-IR was asymmetrically distributed in the cytosol and nuclei of neural stem/progenitor cells. Considering that asymmetric cell division is critical for cell fate specification during stem cell division, asymmetric distribution of Preso1 may contribute to cell fate determination.
Two-month-old male C57BL/6 mice were obtained from Orient Bio, Inc. (Seongnam, Korea). All experiments were carried out in accordance with the ethical guidelines of Korea University and with the approval of the Animal Care and Use Committee of Korea University.
The SVZ was isolated from sections of adult mice brains and then digested with 0.8% papain (Worthington, Lakewood, NJ, USA) and 0.08% dispase II (Roche Applied Science, Indianapolis, IN, USA) in HBSS for 45 minutes at 37℃ for dissociation [5]. Cells were then seeded in an ultra-low attachment surface dish, maintained in suspension culture with DMEM/F12 medium containing 1% N2, 2% B27 supplement, 1% penicillin-streptomycin (Gibco BRL, Grand island, NY, USA), and treated daily with basic fibroblast growth factor (20 ng/mL, Invitrogen, Carlsbad, CA, USA), epidermal growth factor (20 ng/mL, Invitrogen), and L-ascorbic acid (20 ng/mL, Sigma-Aldrich, St. Louis, MO, USA) until neurospheres were formed. The neurospheres were passaged by dissociation into single cells via incubation with accutase (Innovative Cell Technologies, San Diego, CA, USA). Dissociated single cells (passage 1 to 3) were plated on poly-D-lysine (50 µg/mL, Sigma)-coated plates.
For immunohistochemical analysis, the mice were deeply anesthetized with urethane (100 mg/kg, intraperitoneal injection) and perfused with 0.9% saline, followed with 4% paraformaldehyde (PFA). Brains were dissected and fixed overnight in 4% PFA and cryoprotected as previously described [6]. Brains were sectioned at 40 µm and were blocked with 3% bovine serum albumin and 0.1% Triton X-100 in phosphate-buffered saline (PBS) for 1 hour. Samples were incubated overnight at 4℃ with primary antibodies: rabbit anti-Preso1 (1:500) [1], mouse anti-Nestin (1:500, Millipore, Billerica, MA, USA), rat antiglial fibrillary acidic protein (anti-GFAP; 1:1,000, Invitrogen) goat antidoublecortin (anti-DCX; 1:500, Santa Cruz Biotechnology, Santa Cruz, CA, USA), mouse anti-GM130 (1:500, BD Transduction Laboratories, San Jose, CA, USA), mouse anti-Trim32 (1:500, Abnova, Taipei, Taiwan), and mouse anti-NeuN (1:1,000; Millipore). To visualize F-actin in the cells, neural stem cells (NSCs) were stained with rhodamine-phalloidin (1:500, Molecular Probes, Eugene, OR, USA) for 30 minutes. The specificity of the anti-Preso1 antibody has been confirmed in previous reports [1,2]. Sections were washed with PBS and incubated with secondary antibodies at room temperature for 30 minutes. Subsequently, sections were washed, mounted, and examined under confocal microscopy (LSM510, Zeiss, Goettingen, Germany).
The parasagittal image was used to investigate the distribution of Preso1 in the adult brain (Fig. 1). Preso1-IR was expressed in many brain regions, as previously reported [1]. In particular, we observed that Preso1-IR was highly expressed in the rostral migratory stream (RMS), which is a migratory route for immature neuroblasts (Fig. 1A, B). Double labeling of Preso1 with Nestin (a stem/progenitor cells marker) (Fig. 1C-E) or DCX (a neuroblast marker) (Fig. 1F-H) revealed that Preso1-IR was predominantly localized in DCX-expressing neuroblasts in the RMS. Upon arrival at the olfactory bulb (OB), neuroblasts matured into NeuN-expressing neurons. In the OB, Preso1-IR was substantially stronger in NeuN-negative cells (which are immature cells found in the subependyma) compared to mature NeuN-expressing OB neurons (Fig. 1I-K). These results clearly indicate that Preso1 expression is down-regulated during OB neuronal maturation.
As we observed strong Preso1-IR expression in migrating neuroblasts, we further investigated the expression patterns of Preso1-IR in NSCs in the SVZ via double labeling of Preso1 with GFAP (a marker for NSCs in the SVZ) (Fig. 2A-C), Nestin (Fig. 2D-F), and DCX (Fig. 2G-I). We found that most GFAP-expressing cells did not exhibit Preso1-IR, while a subset of Nestin-expressing cells expressed Preso1-IR, and the majority of DCX-expressing neuroblasts exhibited strong Preso1-IR. Thus, it appeared that Preso1-IR first appeared in transit-amplifying cells, and then was maintained by DCX-expressing neuroblasts (Fig. 2J).
Next, we explored the distribution of Preso1-IR in cultured adult NSCs (Fig. 3). Interestingly, NSCs dissociated from neurospheres exhibited a flat morphology and Preso1-IR showed unequal distribution in the cell cytosol (Fig. 3A-C), in contrast to the F-actin counter-staining. However, when neurospheres adhered to the culture dish and were allowed to migrate out from the core, NSCs formed a typical bipolar morphology (Fig. 3D-F). In migrating NSCs, however, Preso1-IR was rather evenly distributed in the leading and tail processes, which were distinguishable by the localization of the Golgi complex at the leading process. Thus, unequal distribution of Preso1 was not related to the polarization of cells during cell migration.
Next, we examined the distribution of Preso1-IR in another neurogenic region, the SGZ of the DG (Fig. 4). As previously reported, Preso1-IR was strongly expressed in the CA1 region of the hippocampal formation [1]. The DG also exhibited strong Preso1-IR expression. Interestingly, Preso1-IR was localized in the nuclei of a subset of cells in the SGZ. Double labeling of Preso1 with Nestin (Fig. 4A-F) and DCX (Fig. 4G-L) indicated that nuclear Preso1-IR occurred exclusively in DCX-expressing neuroblasts. Since neuroblasts are produced from NSCs, this exclusive distribution of nuclear Preso1-IR may suggest the transient translocalization of Preso1 into the nucleus of neuronal daughter cells during asymmetric cell division. To confirm this, we used Trim32, a neuroblast marker generated from NSCs [7]. As expected, we also found that Trim32 signals were exclusively localized in Tuj-1-expressing neuroblasts during asymmetric cell division in DG NSCs in vitro (Fig. 5A-C). We next tested whether nuclear Preso1-IR was localized in newly generated neuroblasts in the DG. Double labeling of Trim32 and Preso1 revealed that the Preso1 signal was exclusively localized in Trim32-expressing cells in the SGZ (Fig. 5D-G). These results indicate that nuclear Preso1-IR first appeared immediately after asymmetric cell division, and was maintained transiently during early maturation as cells expressed DCX. Following this period, Preso1 appeared to be translocated to the cytoplasm (Fig. 5H).
In this study, we explored the distribution of Preso1-IR in NSCs and neuronal progenitor populations in the adult mouse brain. As we clearly demonstrated, Preso1-IR was enhanced in the cytosol of neuroblasts in the RMS and in the nuclei of neuroblasts in the SGZ. Upon differentiation, OB neurons exhibited a low level of Preso1-IR, whereas DG neurons maintained a moderate level of Preso1-IR in their cytosol. These results indicate that Preso1 function is controlled by its expression level and intracellular localization in a cell type- and stage-specific manner. We recently found that ezrin/radixin/moesin (ERM) family proteins which also have FERM domains were expressed differently among cells localized in the stem cell niche [5]. For example, ezrin is strongly expressed in the NSCs and astrocytes surrounding the RMS, but is transiently expressed in migrating neuroblasts. On the other hand, radixin is exclusively found in migrating neuroblasts. Furthermore, these ERM proteins play critical roles in the regulation of neuroblast migration following brain injury [5]. They also exhibit distinct cell-type-dependent expression profiles, suggesting the specificity of their biological functions.
We found that Preso1-IR was asymmetrically localized in the cytosol of proliferating NSCs. In particular, Preso1-IR was found in the nuclei of neuroblasts following asymmetric cell division of NSCs in the SGZ. PDZ domains play an essential role in the polarized localization of integral membrane proteins [8]. Several Frmpd family proteins are involved in asymmetric protein distribution during cell division and in lateral protein targeting via interactions with polarity proteins [4,9]. For asymmetric cell division, the asymmetric protein LGN plays an essential role in orienting the mitotic spindle along the axis of cell polarity. Frmpd proteins may be involved in asymmetric cell division via protein-protein interacting modules [4,10]. Although Preso1-IR was not found in the mitotic spindle, its distribution was unequal within the cell and independent of cell polarity formed along the migratory axis. Therefore, it is plausible that unequal distribution of Preso1 is mediated by interactions with other novel proteins.
It is interesting that Preso1-IR was found in the nuclei of hippocampal neuroblasts. Preso1 contains one WW, one FERM, and two PDZ domains that are associated with various intracellular effectors. A number of members of the FERM family of proteins are localized to the plasma membrane-actin cytoskeleton interface through their FERM domain [11,12]. However, several FERM-containing proteins, such as ERM-binding phosphoprotein 50 (EBP50) and ezrin/moesin, have also been found in the nuclei under certain biological conditions [13,14]. Although the function of nuclear ERM proteins remains unclear, these results suggest that some of cytoskeletal linker proteins can contribute to nuclear function through nucleo-cytoplasmic shuttling. Since ERM proteins can bind to monomeric and polymerized forms of actin, they may have nuclear functions related to nuclear actin binding. For nuclear transport of these proteins, short nuclear localization sequences (NLSs) are required. Nuclear ERM proteins, such as EBP50 or CD44ICD, have functional NLSs [14,15,16]; however, we failed to identify any NLSs in Preso1, and it remains unclear whether Preso1 has atypical NLSs or if its nucleo-cytoplasmic shuttling is mediated by binding with uncharacterized nuclear proteins.
In addition, there are several transcription factors that contain PDZ domains. For instance, similar to Preso1, Yes-associated protein 65 (YAP) contains PDZ and WW domains, while it lacks a FERM domain [17]. The YAP protein plays a critical role in neural induction and regulates pax3 transcription in neural crest progenitors during early development [18]. As a homolog of YAP, transcriptional coactivator with PDZ-binding motif (TAZ) also has PDZ and WW domains [19]. Interestingly, TAZ activity is regulated by nucleo-cytoplasmic shuttling during stem cell differentiation [20]. Phosphorylated TAZ binds to the 14-3-3 protein and thus localizes to the cytoplasm [19]. When TAZ is dephosphorylated, it is translocated to the nucleus, where it interacts with other transcription factors such as Pax3 [21], Pax8 [22], and RUNX2 [23]. This contributes to the tissue-specific differentiation of mesenchymal stem cells. Moreover, interaction of nuclear TAZ with SMAD2/3 is required to ensure the self-renewal capacity of human embryonic stem cells [24]. Collectively, these results suggest that nuclear Preso1 may be involved in renewal vs. differentiation of hippocampal neural progenitor cells.
Figures and Tables
Fig. 1
Distribution of Preso1-IR in the adult forebrain. (A-E) In the parasagittal view, Nestin (red)-expressing neuroblasts in the rostral migratory stream (RMS) are colabeled with Preso1 (green). (F-H) In the coronal section of the RMS, doublecortin (DCX)-expressing neuroblasts (red) also exhibited immunoreactivity for Preso1 (Preso1-IR; green). (I-K) In the subependymal layer (SEL) of the olfactory bulb (OB), NeuN-negative migrating neuroblasts strongly exhibited Preso1-IR, but Preso1-IR was markedly reduced in mature NeuN-expressing neurons (red) in the granule cell layer (GCL). Nuclei were counterstained with Hoechst33342 (blue). The scale bar in B=500 µm, in E, H, K=20 µm. LV, lateral ventricle.
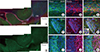
Fig. 2
Immunoreactivity for Preso1 (Preso1-IR) in the subventricular zone (SVZ) of the adult mouse. (A-C) The majority of glial fibrillary acidic protein (GFAP)-expressing astrocytes (red) and stem cells did not exhibit Preso1-IR (green). (D-F) A few Nestin-expressing transit amplifying stem cells (red) exhibited Preso1-IR (green). (G-I) The majority of doublecortin (DCX)-expressing neuroblasts (red) also exhibited strong Preso1-IR (green). (J) Schematic diagram of the Preso1-expressing stage (green) of adult neural stem cells (NSCs) in the SVZ. The scale bar in I=10 µm, enlarged inset in I=5 µm. TAC, transit-amplifying cell.
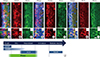
Fig. 3
Polarized distributions of immunoreactivity for Preso1 (Preso1-IR) in cultured neural stem cells (NSCs). (A-C) NSCs were labeled with Preso1IR (green), F-actin (red), and nuclei (blue) were counterstained with rhodamine-phalloidin and Hoechst33342, respectively. Preso1-IR was unequally distributed in the cell cytosol. (D-F) NSCs migrating out from the neurosphere cores were stained with Preso1 (green) and GM130 (red), a Golgi apparatus marker. Preso1-IR was evenly distributed regardless of the cell polarity in migrating NSCs.
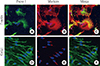
Fig. 4
Distribution of immunoreactivity for Preso1 (Preso1-IR) in the subgranular zone (SGZ) of the hippocampal formation. (A-C) Preso1-IR (green) was found throughout the adult hippocampus, while Nestin (red) was expressed in the SGZ. (D-F) In the SGZ, Preso1-IR was strongly expressed in the nuclei of a subset of cells. Cells with nuclear Preso1-IR did not express Nestin (red). (G-L) Most doublecortin (DCX)-expressing neuroblasts (red) exhibited nuclear Preso1-IR (green). Nuclei were counter-stained with Hoechst33342 (blue). The scale bar in F=5 µm, I=50 µm.
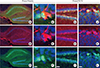
Fig. 5
Polarized Preso1 expression during asymmetric cell division. (A-C) Tuj-1 (red) expressing neuronal daughter cell-expressed Trim32 (green) in cultured neural stem cells (NSCs). (D-G) In the subgranular zone (SGZ), Trim32 (red)-expressing daughter cells also expressed nuclear Preso1-IR (green). (H) Schematic diagram of the Preso1 expression stages of the adult NSCs of the SGZ. RGL, radial glia-like; IPCs, intermediate precursor cells; GFAP, glial fibrillary acidic protein; DCX, doublecortin.
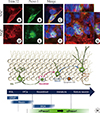
ACKNOWLEDGMENTS
This research was supported by the Brain Research Program through the National Research Foundation of Korea (NRF) funded by the Ministry of Science, ICT and Future Planning (NRF-2010-0020237, NRF-2011-0019212, NRF-2012M3A 9C6049933, and NRF-2012R1A1A3014385).
References
1. Lee HW, Choi J, Shin H, Kim K, Yang J, Na M, Choi SY, Kang GB, Eom SH, Kim H, Kim E. Preso, a novel PSD-95-interacting FERM and PDZ domain protein that regulates dendritic spine morphogenesis. J Neurosci. 2008; 28:14546–14556.
2. Mo J, Lee D, Hong S, Han S, Yeo H, Sun W, Choi S, Kim H, Lee HW. Preso regulation of dendritic outgrowth through PI(4,5)P2-dependent PDZ interaction with betaPix. Eur J Neurosci. 2012; 36:1960–1970.
3. Hu JH, Yang L, Kammermeier PJ, Moore CG, Brakeman PR, Tu J, Yu S, Petralia RS, Li Z, Zhang PW, Park JM, Dong X, Xiao B, Worley PF. Preso1 dynamically regulates group I metabotropic glutamate receptors. Nat Neurosci. 2012; 15:836–844.
4. Yuzawa S, Kamakura S, Iwakiri Y, Hayase J, Sumimoto H. Structural basis for interaction between the conserved cell polarity proteins Inscuteable and Leu-Gly-Asn repeat-enriched protein (LGN). Proc Natl Acad Sci U S A. 2011; 108:19210–19215.
5. Moon Y, Kim JY, Kim WR, Kim HJ, Jang MJ, Nam Y, Kim K, Kim H, Sun W. Function of ezrin-radixin-moesin proteins in migration of subventricular zone-derived neuroblasts following traumatic brain injury. Stem Cells. 2013; 31:1696–1705.
6. Kim WR, Chun SK, Kim TW, Kim H, Ono K, Takebayashi H, Ikenaka K, Oppenheim RW, Sun W. Evidence for the spontaneous production but massive programmed cell death of new neurons in the subcallosal zone of the postnatal mouse brain. Eur J Neurosci. 2011; 33:599–611.
7. Schwamborn JC, Berezikov E, Knoblich JA. The TRIM-NHL protein TRIM32 activates microRNAs and prevents self-renewal in mouse neural progenitors. Cell. 2009; 136:913–925.
8. Kim SK. Polarized signaling: basolateral receptor localization in epithelial cells by PDZ-containing proteins. Curr Opin Cell Biol. 1997; 9:853–859.
9. Stenzel N, Fetzer CP, Heumann R, Erdmann KS. PDZ-domain-directed basolateral targeting of the peripheral membrane protein FRMPD2 in epithelial cells. J Cell Sci. 2009; 122(Pt 18):3374–3384.
10. Pan Z, Shang Y, Jia M, Zhang L, Xia C, Zhang M, Wang W, Wen W. Structural and biochemical characterization of the interaction between LGN and Frmpd1. J Mol Biol. 2013; 425:1039–1049.
11. Tsukita S, Yonemura S. ERM (ezrin/radixin/moesin) family: from cytoskeleton to signal transduction. Curr Opin Cell Biol. 1997; 9:70–75.
12. Tsukita S, Yonemura S. Cortical actin organization: lessons from ERM (ezrin/radixin/moesin) proteins. J Biol Chem. 1999; 274:34507–34510.
13. Batchelor CL, Woodward AM, Crouch DH. Nuclear ERM (ezrin, radixin, moesin) proteins: regulation by cell density and nuclear import. Exp Cell Res. 2004; 296:208–222.
14. Lin YY, Hsu YH, Huang HY, Shann YJ, Huang CY, Wei SC, Chen CL, Jou TS. Aberrant nuclear localization of EBP50 promotes colorectal carcinogenesis in xenotransplanted mice by modulating TCF-1 and beta-catenin interactions. J Clin Invest. 2012; 122:1881–1894.
15. Legg JW, Isacke CM. Identification and functional analysis of the ezrin-binding site in the hyaluronan receptor, CD44. Curr Biol. 1998; 8:705–708.
16. Tsukita S, Oishi K, Sato N, Sagara J, Kawai A, Tsukita S. ERM family members as molecular linkers between the cell surface glycoprotein CD44 and actin-based cytoskeletons. J Cell Biol. 1994; 126:391–401.
17. Sudol M, Bork P, Einbond A, Kastury K, Druck T, Negrini M, Huebner K, Lehman D. Characterization of the mammalian YAP (Yes-associated protein) gene and its role in defining a novel protein module, the WW domain. J Biol Chem. 1995; 270:14733–14741.
18. Gee ST, Milgram SL, Kramer KL, Conlon FL, Moody SA. Yes-associated protein 65 (YAP) expands neural progenitors and regulates Pax3 expression in the neural plate border zone. PLoS One. 2011; 6:e20309.
19. Kanai F, Marignani PA, Sarbassova D, Yagi R, Hall RA, Donowitz M, Hisaminato A, Fujiwara T, Ito Y, Cantley LC, Yaffe MB. TAZ: a novel transcriptional co-activator regulated by interactions with 14-3-3 and PDZ domain proteins. EMBO J. 2000; 19:6778–6791.
20. Liu C, Huang W, Lei Q. Regulation and function of the TAZ transcription co-activator. Int J Biochem Mol Biol. 2011; 2:247–256.
21. Murakami M, Tominaga J, Makita R, Uchijima Y, Kurihara Y, Nakagawa O, Asano T, Kurihara H. Transcriptional activity of Pax3 is co-activated by TAZ. Biochem Biophys Res Commun. 2006; 339:533–539.
22. Di Palma T, D'Andrea B, Liguori GL, Liguoro A, de Cristofaro T, Del Prete D, Pappalardo A, Mascia A, Zannini M. TAZ is a coactivator for Pax8 and TTF-1, two transcription factors involved in thyroid differentiation. Exp Cell Res. 2009; 315:162–175.
23. Cui CB, Cooper LF, Yang X, Karsenty G, Aukhil I. Transcriptional coactivation of bone-specific transcription factor Cbfa1 by TAZ. Mol Cell Biol. 2003; 23:1004–1013.
24. Varelas X, Sakuma R, Samavarchi-Tehrani P, Peerani R, Rao BM, Dembowy J, Yaffe MB, Zandstra PW, Wrana JL. TAZ controls Smad nucleocytoplasmic shuttling and regulates human embryonic stem-cell self-renewal. Nat Cell Biol. 2008; 10:837–848.