Abstract
Background
Glucagon-like peptide-1 (GLP-1) is an incretin hormone produced by cleavage of proglucagon in intestinal L-cells. In the pancreas, GLP-1 stimulates post-prandial insulin secretion, promotes insulin biosynthesis, and improves insulin sensitivity. Because of its insulinotropic activity, GLP-1 has been considered a good candidate drug for treatment of diabetes mellitus. However, clinical use of GLP-1 has been limited by its short half-life, as a result of rapid degradation by dipeptidyl peptidase-IV (DPP-IV).
Methods
We designed a novel GLP-1 analog, Xenopus GLP-1 (xGLP)-E4. The Ala residue in the second position of xGLP was replaced with a Ser residue to increase the half-life in the body. The C-terminal tail of exendin-4 was added to enhance the binding affinity for the GLP-1 receptor (GLP1R). The potency of GLP-1 and its analogs was determined by luciferase assay. The stability of GLP1R agonists was evaluated by determining the activity of agonists that had been preincubated in the presence of fetal bovine serum, which contains innate DPP-IV activity. The effects of xGLP-E4 on insulin secretion and β-cell growth were investigated using insulin enzyme-linked immunosorbent assay and cell counting.
After the idea of intestinal factors regulating the function of endocrine secretion from the pancreas was first described by Moore and his colleagues in 1906, the incretins were introduced in the 1930s [1,2]. Incretins are intestinal hormones that regulate glucose-dependent insulin secretion in response to nutrient ingestion. Glucagon-like peptide-1 (GLP-1) and glucose-dependent insulinotropic polypeptide are incretins [2]. GLP-1 is a 30-amino acid peptide secreted by intestinal L-cells [3] that regulates insulin secretion through binding to its receptor, GLP1R [4]. It is well established that GLP-1 augments insulin secretion in animal models and patients with type 2 diabetes [5,6]. GLP-1 stimulates insulin biosynthesis and growth, differentiation, and survival of β-cells [7,8,9,10]. In addition, GLP-1 decreases glucagon secretion on pancreatic β-cells [11]. In the brain, GLP-1 is known to promote satiety by regulating appetite and food intake, resulting in loss of body weight [12]. These diverse physiological functions of GLP-1 contribute to regulation of normal blood glucose level. The advantage of GLP-1 as a drug for type 2 diabetes is that it does not cause hypoglycemia under conditions of normal plasma glucose concentration due to its glucose-dependent action [11,13]. GLP-1 is able to stimulate insulin secretion in patients resistant to sulfonylurea drugs [14]. However, GLP-1 has an extremely short plasma half-life due to rapid degradation by dipeptidyl peptidase-IV (DPP-IV) [15]. DPP-IV selectively cleaves peptides with Pro and Ala in the second position. Exendin-4, which is found in the venom of the Gila monster, Heloderma suspectum, is a more potent GLP1R agonist than GLP-1 and is used clinically for treatment of type 2 diabetes [16,17]. Exendin-4 consists of 39 amino acids and exhibits 53% amino acid sequence similarity with GLP-1 [16]. The exendin-4 C-terminal tail, which consists of nine amino acid residues, enhances the binding affinity of exendin-4 to the N-terminal extracellular domain (ECD) of GLP1R [18]. Exendin-4 has a Gly residue in the second position, making it resistant to DPP-IV-mediated degradation [19]. However, exendin-4 is recognized as an antigen by the human immune system due to differences in amino acid sequence compared with GLP-1 [20]. Thus, more potent and long-acting GLP1R agonists are needed.
The amino acid sequence of GLP-1 is highly conserved among mammals and exhibits a high degree of identity among lower vertebrates [2,21,22,23]. It has been reported that non-mammalian GLP-1s are able to activate human GLP1R with high potency. Introduction of amino acid residues present in nonmammalian GLP-1 into human GLP-1 is a useful tool for developing novel GLP-1 analogs that are more potent than GLP-1. In the present study, we designed GLP-1 analog based on the amino acid sequences of Xenopus GLP-1 (xGLP) [24] and exendin-4. This analog exhibits resistance to DPP-IV mediated degradation and shows more potent activity toward GLP1R than wild-type human GLP-1.
GLP-1, exendin-4, xGLP, and the chimeric xGLP-E4 peptide were synthesized by AnyGen (Gwangju, Korea). The amino acid sequences of native GLP-1, exendin-4, xGLP, and xGLP-E4 are shown in Table 1.
The CRE-luc vector, which contains four copies of the cyclic AMP-responsive element (CRE: TGACGTCA) was from Stratagene (La Jolla, CA, USA). GLP1R cDNA was kindly provided by Dr. Bernard Thorens (Institute of Pharmacology and Toxicology, Lausanne, Switzerland).
HEK 293T cells were maintained at 37℃ in Dulbecco's Modified Eagle's medium (DMEM) supplemented with 10% heat-inactivated fetal bovine serum (FBS). INS-1 cells, rat pancreatic β-cells, were grown in RPMI 1640 medium containing 10% FBS at 37℃. Mouse pancreatic cells, β-TC-6 cells, were cultured in DMEM in the presence of 15% FBS at 37℃.
HEK 293T cells were plated in 48-well plates and transfected with Effectene reagent (Qiagen, Hilden, Germany) according to the manufacturer's instructions. Approximately 48 hours after transfection, cells were treated with the respective ligands for 6 hours. Cells were then harvested, and luciferase activity was determined in cell extracts using a luciferase assay system according to standard methods for the Synergy 2 Multi-Mode Microplate Reader (BioTek, Winooski, VT, USA).
To evaluate the stability of GLP-1 and its analogs against DPP-IV activity, peptides were incubated at an initial concentration of 100 nM in DMEM containing 10% FBS or in 100% FBS at 37℃ for 0, 12, 24, 48, 72, and 96 hours in the presence or absence of 0.2 mM DPP-IV inhibitor diprotin A (Sigma-Aldrich, St Louis, MO, USA). FBS contains innate DPP-IV activity [25,26]. Residual peptide activity was then assessed by measuring luciferase activity after treatment of the GLP1R-and CRE-luc-cotransfected cells.
INS-1 cells and β-TC-6 cells were cultured in 24-well plates until 90% confluence. Cells were washed and incubated in KRB buffer (118.5 mM NaCl, 4.8 mM KCl, 2.7 mM CaCl2, 1.2 mM KH2PO4, 1.1 mM MgSO4·7H2O, 25 mM NaHCO3, and 4% bovine serum albumin, pH 7.4) and treated with low (2.8 mM) or high (16.7 mM) glucose for 2 hours at 37℃. Insulin secretion in response to treatment with 10 nM GLP-1 analogs was determined, after another 2-hour incubation of cells in KRB buffer containing low and high glucose, using an enzyme-linked immunosorbent assay kit according to the manufacturer's instructions (Millipore, Billerica, MA, USA).
INS-1 cells were seeded in 12-well plates at a density of 4×104 cells/well. At seeding and every 2 days thereafter, the respective peptides were added to the cells in fresh medium containing the appropriate concentration of glucose. Six and 10 days after cell seeding cells were washed with phosphate-buffered saline and detached with trypsin-ethylenediaminetetraacetic acid (Sigma-Aldrich). Harvested cells were counted with a hemocytometer.
To develop a potent and long-acting GLP-1 analog, we performed amino acid sequence alignment of human GLP-1, xGLP, and exendin-4. These peptides share a high degree of amino acid sequence similarity at their N-terminal parts. Exendin-4 has a Gly residue in the second position and an additional nine residues at the C-terminal tail compared with human and xGLP (Table 1). This C-terminal tail is known to enhance the binding affinity of exendin-4 to the ECD of GLP1R [18]. We engrafted the nine C-terminal residues onto the C-terminal end of xGLP to increase the potency toward GLP1R. The Ala residue in the second position of xGLP was replaced with Ser to prevent DPP-IV mediated degradation.
The potency of xGLP-E4 was examined by luciferase assay in GLP1R and CRE-luc cotransfected HEK293T cells. The potency of exendin-4 (log EC50 value, -9.71±0.21) was slightly stronger than that of human GLP-1 (log EC50 value, -9.20±0.11) and xGLP (log EC50 value, -9.08±0.12). xGLP activated human GLP1R with potency equal to that of human GLP-1. xGLP-E4 exhibited higher activity (log EC50 value, -9.81±0.17) toward GLP1R than that of native human GLP-1 (Fig. 1).
The stability of GLP-1 analogs was evaluated after incubation in DMEM containing FBS, which has innate DPP-IV activity. This assay was designed to measure bioactivity of the residual peptide to activate GLP1R in HEK 293T cells cotransfected with GLP1R and CRE-luc. When GLP-1 was incubated with 10% FBS, luciferase activity rapidly decreased. However, when diprotin A, a specific DPP-IV inhibitor, was added at the beginning of the incubation in the presence of FBS, the activity of GLP-1 was maintained for more than 72 hours (Fig. 2A). This result suggests that decreased activity of GLP-1 after incubation with FBS was due to degradation by DPP-IV. xGLP-E4 remained stable for more than 72 hours in DMEM containing 10% FBS, as did exendin-4 (Fig. 2A). xGLP-E4 and exendin-4 sustained more than 50% of their initial activity after 96-hour incubation with 100% FBS (Fig. 2B). GLP-1, however, completely lost its ability to activate GLP1R 72 hours after incubation in 100% FBS (Fig. 2B). These results show that xGLP-E4 is more stable in serum than wild-type human and xGLP.
To investigate the effect of xGLP-E4 on insulin secretion, glucose-dependent insulin secretion was measured in rat (INS-1) and mouse (β-TC-6) pancreatic β-cells. In INS-1 cells, xGLP-E4 induced a higher level of insulin secretion than human GLP-1 in the presence of a high (16.7 mM) concentration of glucose (Fig. 3A). xGLP-E4 significantly increased insulin release at a high concentration of glucose in β-TC-6 cells (Fig. 3B).
It is well known that GLP-1 increases β-cell proliferation [10]. To evaluate the effect of GLP-1 and analogs on β-cell growth, cells were treated with peptides every other day in the presence of low and high concentrations of glucose, and cells were counted 6 and 10 days after seeding. Under conditions of low glucose concentration, GLP-1 did not increase β-cell growth. However, exendin-4 and xGLP-E4 significantly stimulated β-cell proliferation 6 days after seeding (Fig. 4A). Ten days after cell seeding, GLP-1, exendin-4, and xGLP-E4 significantly increased β-cell proliferation. The effect of exendin-4 and xGLP-E4 on β-cell growth was greater than that of GLP-1 (Fig. 4B). It is well known that high concentration of glucose promotes cell proliferation and growth [27]. Under high glucose condition, the effect of glucose on cell growth is likely greater than that of GLP-1 analogs. Thus, growth effect of GLP-1 analogs may not be seen under high glucose concentration condition (Fig. 4). However, 10 days after cell seeding, only xGLP-E4 significantly increased β-cell growth (Fig. 4B).
As GLP-1 stimulates insulin secretion and inhibits glucagon secretion in type 2 diabetes patients with residual β-cell function, the use of GLP-1 has been proposed as a novel therapeutic option. The short biological half-life, however, considerably limits the use of GLP-1 in diabetes therapy [28]. Therefore, two strategies have been pursued, the development of inhibitors of DPP-IV and DPP-IV-resistant analogs of GLP-1 [29,30]. Administration of DPP-IV inhibitors reduces glycemic excursion, enhances insulin secretion, and inhibits degradation of GLP-1 in normal and diabetic rodents, as well as in human subjects [30,31]. Experiments using these DPP-IV inhibitors in animals showed that GLP-1 degradation could be prevented, and glucose tolerance was improved [31,32]. However, because DPP-IV ubiquitously degrades important regulatory peptides in addition to GLP-1 [33], the use of a DPP-IV inhibitor might theoretically cause widespread deleterious biological effects. The strategy of using GLP-1 analogs for diabetes therapy has the advantage over pharmacological DPP-IV inhibition that it is highly specific and does not lead to ubiquitous inhibition of the degradation of multiple regulatory peptides. Therefore, there has been much interest in designing more effective and long-acting GLP-1 analogs.
His1, Gly4, Thr7, and Asp9 in the N-terminal portion of GLP-1 are important for receptor binding and activation [34,35,36]. The hydrophobic face of GLP-1, formed by the conserved residues Phe22, Ile/Val23, Trp25, and Leu26, is responsible for interacting with the hydrophobic binding pocket in the ECD of GLP1R [37]. These critical amino acid residues are conserved between human and xGLP. For this reason, xGLP is able to activate human GLP1R with high potency (Fig. 1). The C-terminal region of exendin-4 binds to the N-terminal domain of the receptor and provides an additional interaction [18]. We generated xGLP-E4 having the C-terminal tail of exendin-4. xGLP-E4 showed higher activity toward GLP1R than wild-type human GLP-1. In addition, the stability and half-life of a drug are important prerequisites for clinical application. Human and xGLP have Ala at the second position, which is a target site of DPP-IV mediated degradation [38]. To prevent this degradation, we replaced Ala of xGLP-E4 with a Ser residue. The C-terminal tail derived from exendin-4 also protects this peptide from DPP-IV activity. As a result, the longevity of xGLP-E4 was extended, as was that of exendin-4.
The most important effect of a GLP-1 analog is the ability to stimulate glucose-dependent insulin secretion in β-cells. xGLP-E4 increased insulin secretion in rat and mouse β-cell lines at a high glucose concentration. Because xGLP-E4 did not increase insulin secretion at a low glucose concentration, long-term exposure of xGLP-E4 may not cause hypoglycemia. xGLP-E4 also induced β-cell growth, suggesting that this analog may be useful for treatment of type 1 diabetes. Currently, exendin-4 is clinically used for treatment of type 2 diabetes. Many studies have investigated the safety of it, and no evidence for cardiovascular, pulmonary, hepatic, or renal toxicity of exendin-4 has been reported [17,39]. The incidence of serious and severe adverse events is low although the most frequent adverse events are gastrointestinal symptoms, particularly nausea, vomiting, and diarrhea [17,39]. Since the amino acid sequence of xGLP-E4 is more similar to that of natural GLP-1 than exendin-4, xGLP-E4 may have fewer side effects when it is applied in vivo.
In summary, this study shows that modification of the amino acid sequence of native GLP-1 generates a novel GLP-1 analog with greater receptor affinity, biological activity, and stability. This analog may be a useful therapeutic agent for treatment of type 1 and 2 diabetes.
Figures and Tables
Fig. 1
Potency of glucagon-like peptide-1 (GLP-1) analogs toward GLP-1 receptor (GLP1R). Ligand potencies of GLP-1 analogs were examined using HEK293T cells expressing GLP1R. Cells were treated with increasing concentrations of GLP-1 analogs for 6 hours, and luciferase activity was measured. The data on the sigmoidal curves and EC50 values are presented as means±standard error of the mean of at least three independent experiments. CRE-luc, cAMP response element-luciferase; xGLP, Xenopus GLP-1.
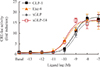
Fig. 2
Stability of glucagon-like peptide-1 (GLP-1) analogs. The stability of GLP-1 and GLP-1 analogs against dipeptidyl peptidase-IV (DPP-IV) activity was evaluated by incubating the individual peptides in medium containing 10% fetal bovine serum (FBS) (A) or in 100% FBS (B). Peptides were incubated at an initial concentration of 100 nM in Dulbecco's Modified Eagle's medium containing 10% FBS or 100% FBS at 37℃ in the presence or absence of 0.2 mM of the peptidase inhibitor diprotin A. Peptide activity was then assessed by measuring luciferase activity in cells expressing GLP1R and CRE-luc. Results are presented as mean±standard error of the mean of at least three independent experiments.
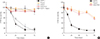
Fig. 3
Induction of glucose-dependent insulin secretion by (xGLP-E4). (A) INS-1 cells and (B) β-TC-6 cells were cultured in 24-well plates until 90% confluence. Cells were washed and incubated in Krebs-Ringer Bicarbonate (KRB) buffer and treated with glucose for 2 hours at 37℃. Cells were incubated with 10 nM glucagon-like peptide-1 (GLP-1) analog in KRB buffer containing low and high glucose for another 2 hours, and insulin secretion into the buffer was then determined. CTL, control; GLP-1, glucagon-like peptide-1; Exe-4, exendin-4; xGLP, Xenopus GLP-1.
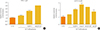
Fig. 4
Induction of β-cell growth by (xGLP-E4). INS-1 cells were seeded in 12-well plates at a density of 4×104 cells/well. Every 2 days, the respective peptides were added to the cultures in fresh medium containing the appropriate concentration of glucose. (A) Six and (B) 10 days after cell seeding, cells were washed, harvested, and counted. Results are presented as mean±standard error of the mean of at least three independent experiments. GLP-1, glucagon-like peptide-1; Exe-4, exendin-4; xGLP, Xenopus GLP-1. a vs. control (CTL) (P<0.05); b vs. CTL (P<0.001).
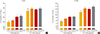
ACKNOWLEDGMENTS
This work was supported by grants from the Brain Research Program (2011-0019205) and Basic Science Research Program (2013R1A1A2010481) of the National Research Foundation of Korea.
References
1. Moore B. On the treatment of diabetus mellitus by acid extract of duodenal mucous membrane. Biochem J. 1906; 1:28–38.
2. Fehmann HC, Goke R, Goke B. Cell and molecular biology of the incretin hormones glucagon-like peptide-I and glucose-dependent insulin releasing polypeptide. Endocr Rev. 1995; 16:390–410.
3. Orskov C, Holst JJ, Knuhtsen S, Baldissera FG, Poulsen SS, Nielsen OV. Glucagon-like peptides GLP-1 and GLP-2, predicted products of the glucagon gene, are secreted separately from pig small intestine but not pancreas. Endocrinology. 1986; 119:1467–1475.
4. Graziano MP, Hey PJ, Borkowski D, Chicchi GG, Strader CD. Cloning and functional expression of a human glucagon-like peptide-1 receptor. Biochem Biophys Res Commun. 1993; 196:141–146.
5. Shen HQ, Roth MD, Peterson RG. The effect of glucose and glucagon-like peptide-1 stimulation on insulin release in the perfused pancreas in a non-insulin-dependent diabetes mellitus animal model. Metabolism. 1998; 47:1042–1047.
6. Drucker DJ. Minireview: the glucagon-like peptides. Endocrinology. 2001; 142:521–527.
7. Fehmann HC, Habener JF. Insulinotropic hormone glucagon-like peptide-I(7-37) stimulation of proinsulin gene expression and proinsulin biosynthesis in insulinoma beta TC-1 cells. Endocrinology. 1992; 130:159–166.
8. Perfetti R, Merkel P. Glucagon-like peptide-1: a major regulator of pancreatic beta-cell function. Eur J Endocrinol. 2000; 143:717–725.
9. Perfetti R, Zhou J, Doyle ME, Egan JM. Glucagon-like peptide-1 induces cell proliferation and pancreatic-duodenum homeobox-1 expression and increases endocrine cell mass in the pancreas of old, glucose-intolerant rats. Endocrinology. 2000; 141:4600–4605.
10. Drucker DJ. Glucagon-like peptides: regulators of cell proliferation, differentiation, and apoptosis. Mol Endocrinol. 2003; 17:161–171.
11. Nauck MA, Heimesaat MM, Behle K, Holst JJ, Nauck MS, Ritzel R, Hufner M, Schmiegel WH. Effects of glucagon-like peptide 1 on counterregulatory hormone responses, cognitive functions, and insulin secretion during hyperinsulinemic, stepped hypoglycemic clamp experiments in healthy volunteers. J Clin Endocrinol Metab. 2002; 87:1239–1246.
12. Nauck MA, Niedereichholz U, Ettler R, Holst JJ, Orskov C, Ritzel R, Schmiegel WH. Glucagon-like peptide 1 inhibition of gastric emptying outweighs its insulinotropic effects in healthy humans. Am J Physiol. 1997; 273(5 Pt 1):E981–E988.
13. Baggio LL, Drucker DJ. Harnessing the therapeutic potential of glucagon-like peptide-1: a critical review. Treat Endocrinol. 2002; 1:117–125.
14. Nauck MA, Sauerwald A, Ritzel R, Holst JJ, Schmiegel W. Influence of glucagon-like peptide 1 on fasting glycemia in type 2 diabetic patients treated with insulin after sulfonylurea secondary failure. Diabetes Care. 1998; 21:1925–1931.
15. Kieffer TJ, McIntosh CH, Pederson RA. Degradation of glucose-dependent insulinotropic polypeptide and truncated glucagon-like peptide 1 in vitro and in vivo by dipeptidyl peptidase IV. Endocrinology. 1995; 136:3585–3596.
16. Goke R, Fehmann HC, Linn T, Schmidt H, Krause M, Eng J, Goke B. Exendin-4 is a high potency agonist and truncated exendin-(9-39)-amide an antagonist at the glucagon-like peptide 1-(7-36)-amide receptor of insulin-secreting beta-cells. J Biol Chem. 1993; 268:19650–19655.
17. Buse JB, Henry RR, Han J, Kim DD, Fineman MS, Baron AD. Exenatide-113 Clinical Study Group. Effects of exenatide (exendin-4) on glycemic control over 30 weeks in sulfonylurea-treated patients with type 2 diabetes. Diabetes Care. 2004; 27:2628–2635.
18. Al-Sabah S, Donnelly D. A model for receptor-peptide binding at the glucagon-like peptide-1 (GLP-1) receptor through the analysis of truncated ligands and receptors. Br J Pharmacol. 2003; 140:339–346.
19. Edwards CM, Stanley SA, Davis R, Brynes AE, Frost GS, Seal LJ, Ghatei MA, Bloom SR. Exendin-4 reduces fasting and postprandial glucose and decreases energy intake in healthy volunteers. Am J Physiol Endocrinol Metab. 2001; 281:E155–E161.
20. Baggio LL, Holland D, Wither J, Drucker DJ. Lymphocytic infiltration and immune activation in metallothionein promoter-exendin-4 (MT-Exendin) transgenic mice. Diabetes. 2006; 55:1562–1570.
21. Moon MJ, Park S, Kim DK, Cho EB, Hwang JI, Vaudry H, Seong JY. Structural and molecular conservation of glucagon-like peptide-1 and its receptor confers selective ligand-receptor interaction. Front Endocrinol (Lausanne). 2012; 3:141.
22. Hwang JI, Moon MJ, Park S, Kim DK, Cho EB, Ha N, Son GH, Kim K, Vaudry H, Seong JY. Expansion of secretin-like G protein-coupled receptors and their peptide ligands via local duplications before and after two rounds of whole-genome duplication. Mol Biol Evol. 2013; 30:1119–1130.
23. Park CR, Moon MJ, Park S, Kim DK, Cho EB, Millar RP, Hwang JI, Seong JY. A novel glucagon-related peptide (GCRP) and its receptor GCRPR account for coevolution of their family members in vertebrates. PLoS One. 2013; 8:e65420.
24. Irwin DM, Satkunarajah M, Wen Y, Brubaker PL, Pederson RA, Wheeler MB. The Xenopus proglucagon gene encodes novel GLP-1-like peptides with insulinotropic properties. Proc Natl Acad Sci U S A. 1997; 94:7915–7920.
25. Gossrau R. Peptidases II. Localization of dipeptidylpeptidase IV (DPP IV). Histochemical and biochemical study. Histochemistry. 1979; 60:231–248.
26. Burcelin R, Dolci W, Thorens B. Long-lasting antidiabetic effect of a dipeptidyl peptidase IV-resistant analog of glucagon-like peptide-1. Metabolism. 1999; 48:252–258.
27. Kaung HC. Effect of glucose on beta cell proliferation and population size in organ culture of foetal and neonatal rat pancreases. J Embryol Exp Morphol. 1983; 75:303–312.
28. Deacon CF, Nauck MA, Toft-Nielsen M, Pridal L, Willms B, Holst JJ. Both subcutaneously and intravenously administered glucagon-like peptide I are rapidly degraded from the NH2-terminus in type II diabetic patients and in healthy subjects. Diabetes. 1995; 44:1126–1131.
29. Gallwitz B, Ropeter T, Morys-Wortmann C, Mentlein R, Siegel EG, Schmidt WE. GLP-1-analogues resistant to degradation by dipeptidyl-peptidase IV in vitro. Regul Pept. 2000; 86:103–111.
30. Ahren B, Simonsson E, Larsson H, Landin-Olsson M, Torgeirsson H, Jansson PA, Sandqvist M, Bavenholm P, Efendic S, Eriksson JW, Dickinson S, Holmes D. Inhibition of dipeptidyl peptidase IV improves metabolic control over a 4-week study period in type 2 diabetes. Diabetes Care. 2002; 25:869–875.
31. Pauly RP, Demuth HU, Rosche F, Schmidt J, White HA, Lynn F, McIntosh CH, Pederson RA. Improved glucose tolerance in rats treated with the dipeptidyl peptidase IV (CD26) inhibitor Ile-thiazolidide. Metabolism. 1999; 48:385–389.
32. Holst JJ, Deacon CF. Inhibition of the activity of dipeptidyl-peptidase IV as a treatment for type 2 diabetes. Diabetes. 1998; 47:1663–1670.
33. Chen X. Biochemical properties of recombinant prolyl dipeptidases DPP-IV and DPP8. Adv Exp Med Biol. 2006; 575:27–32.
34. Adelhorst K, Hedegaard BB, Knudsen LB, Kirk O. Structure-activity studies of glucagon-like peptide-1. J Biol Chem. 1994; 269:6275–6278.
35. Moon MJ, Kim HY, Kim SG, Park J, Choi DS, Hwang JI, Seong JY. Tyr1 and Ile7 of glucose-dependent insulinotropic polypeptide (GIP) confer differential ligand selectivity toward GIP and glucagon-like peptide-1 receptors. Mol Cells. 2010; 30:149–154.
36. Moon MJ, Kim HY, Park S, Kim DK, Cho EB, Park CR, You DJ, Hwang JI, Kim K, Choe H, Seong JY. Evolutionarily conserved residues at glucagon-like peptide-1 (GLP-1) receptor core confer ligand-induced receptor activation. J Biol Chem. 2012; 287:3873–3884.
37. Underwood CR, Garibay P, Knudsen LB, Hastrup S, Peters GH, Rudolph R, Reedtz-Runge S. Crystal structure of glucagon-like peptide-1 in complex with the extracellular domain of the glucagon-like peptide-1 receptor. J Biol Chem. 2010; 285:723–730.
38. Mentlein R, Gallwitz B, Schmidt WE. Dipeptidyl-peptidase IV hydrolyses gastric inhibitory polypeptide, glucagon-like peptide-1(7-36)amide, peptide histidine methionine and is responsible for their degradation in human serum. Eur J Biochem. 1993; 214:829–835.
39. Kendall DM, Riddle MC, Rosenstock J, Zhuang D, Kim DD, Fineman MS, Baron AD. Effects of exenatide (exendin-4) on glycemic control over 30 weeks in patients with type 2 diabetes treated with metformin and a sulfonylurea. Diabetes Care. 2005; 28:1083–1091.