Polycystic ovarian syndrome (PCOS) remains one of the leading endocrine disorders encountered by women of reproductive age; however, the etiology and pathogenesis of this syndrome remain an area of continued debate and controversy.
PCOS is considered a syndrome due to heterogeneity in the features of this complex disorder. The Rotterdam criteria were established to confirm diagnosis of PCOS in women who have the presence of at least two of the following symptoms; hyperandrogenism, polycystic ovaries and oligo- and/or anovulation [1]. Hyperandrogenism, an increase in circulating androgen levels such as testosterone, androstenedione, or dehydroepiandrosterone, may lead to a variety of clinical features such as hirsutism, a masculine pattern of facial and body hair growth, presents as an increase in terminal hairs on the lip, chin, chest, back, abdomen, or thigh and acne, inflamed sebaceous glands of the skin [2]. Hyperandrogenemia also causes hair loss (alopecia) on the scalp as a result of sensitivity of the hair follicles to androgen [3]. Polycystic ovaries, the presence of multiple (> 10) cysts in an ovary [4], is caused by the arrest of follicle development at an immature stage (Fig. 1). PCOS is named in reference to this morphological change. As the development of these follicles is arrested well before the point of dominant follicle selection, and therefore positive estrogen feedback to the hypothalamus and pituitary axis is lacking, the LH surge is absent in PCOS patients [5-7]. Consequently, ovulation and menstrual cycles are interrupted (oligovulation and oligoamenorrhea, respectively).
PCOS is widely recognized as an endocrine disorder with numerous metabolic consequences. Unfortunately, the metabolic consequences of PCOS are interwoven with the metabolic consequences of obesity and causal relationships are therefore hard to define (i.e. while PCOS is classified as an endocrine disorder, obesity will affect the endocrine parameters of this disorder). Insulin resistance is one of the metabolic diseases associated with PCOS, and is perhaps the most well documented symptom [8]. The clinical manifestation of insulin resistance at a patient level reflects the complexities described above. Approximately half the women with PCOS are obese [9] and therefore prone to the presentation of insulin resistance [8]. However, insulin resistance is also observed in women with PCOS of a normal body weight [10], together indicating that the hyperinsulinemia associated with this syndrome is not specific to the stressors associated with obesity. The relationship between glucose and insulin is also somewhat patient-specific and affected by age, race, and physical condition. Therefore, insulin resistance/glucose tolerance is a metabolic disease associated with PCOS that can manifest itself in more severe outcomes such as diabetes. Cardiovascular risk is increased in patients with PCOS [11-14] and hypertension [15] is the most easily recognized manifesta tion. This metabolic disorder is also associated with obesity and again, insulin resistance is a known cardiovascular risk factor [11]. Insulin affects myocardial and skeletal energy metabolism and physical activity improves the insulin sensitivity of skeletal muscle [10,16-18]. Downstream effects of exercise-dependent changes in insulin include better blood flow, more efficient glycogen synthase activity and glucose transportation [18]. Similar to the individuality of glucose/insulin tolerances, measures of cardiovascular function such as maximal O2 consumption (VO2max) are also subject to age, physical condition and other diseases with VO2max positively correlated to both testosterone and insulin sensitivity [19].
The relationship between steroids, lipids and glucose metabolism is not easily defined. Interactions between these parameters are known and aberrations of one will affect the other as a metabolic consequence will modulate the endocrine environment. As such, many of the symptoms and features of PCOS play on one another to exacerbate the presentation of the others. The interconnection of the symptoms of PCOS has thus made it a large challenge to pinpoint the causal factor(s) as well as those that are secondary to that initial aberration.
PCOS is a very complex disorder with heterogeneity of clinical as well as endocrine features. To treat symptoms caused by the hyperandrogenemia of PCOS (hirsutism, acne, seborrhea and hair loss), antiandrogens such as flutamide and spironolactone are routinely administered [20-27]. Most of all, however, treatment of PCOS patients has been centered on inducing ovulation. Due to an inability to naturally ovulate, PCOS patients receive either pharmacological or surgical treatments to induce ovulation together with other assisted reproductive technology (ART) procedures [28-34]. The most commonly used procedures are to increase sensitivity to insulin, induce the gonadotropin surge, or lower circulating testosterone levels. For each of the symptoms, the following treatments are used.
While the etiology is unclear, it is well established that PCOS and obesity/diabetes are tightly associated. In support of this physiological relationship, weight loss not only improves insulin sensitivity but also often restores ovulatory cycles in some women with PCOS [35-40]. This finding has led to the application of a pharmacological approach for recovering ovulatory cycles in PCOS patients using metformin, an insulin-sensitizing drug [41-45]. Metformin lowers blood sugar levels by decreasing the amount of sugar produced by the liver, increasing the amount of sugar absorbed by muscle cells and decreasing the body's resistance to insulin. Recent studies show that metformin is safe and effective in lowering insulin and improving fertility [46,47].
For a PCOS patient seeking to become pregnant, the first line of therapy may be to treat her with clomiphene citrate (CC). Clomiphene citrate is a selective estrogen receptor modulator (SERM) that acts as an estrogen receptor antagonist in the hypothalamus [48-51]. When administered, CC prevents the negative feedback effect of estrogen, thus stimulating gonadotropin releasing hormone (GnRH) from the hypothalamus, which in turn increases the secretion of gonadotropins from the pituitary. Therefore, CC is widely used to stimulate folliculogenesis and ovulation in PCOS patients. However, in some women, CC is not successful at inducing ovulation. In these cases, the direct injection gonadotropins can be used to stimulate follicle development and ovulation [52-54].
In women for whom CC or gonadotropin treatment is unsuccessful, another commonly used approach is ovarian surgery. Although there are several different techniques, they all involve acute ovarian tissue damage. Various types of ovarian surgery have been employed (wedge resection, electrocautery, laser vaporization, multiple ovarian biopsies and others [55-60]. All of these procedures result in a positively altered endocrine profile after surgery. While the current hypothesis is that a small amount of damage to the ovary works to break the cycle of excessive androgen production and abnormal negative feedback, the mechanism behind the reversal of endocrinological dysfunction in PCOS after ovarian surgery remains incompletely understood.
While the initiating factor of PCOS has yet to be determined, we can evaluate the collective pool of known and suspected features of PCOS to better clarify how each symptom relates and what may be driving the symptoms as a whole. In Figure 2, we summarize the known extraovarian and intraovarian factors, and propose causal relationships among them and their effects on inducing hyperandrogenemia and polycystic ovary (arrows). In this particular 'hypothetical' diagram, theca cell hyperplasia, an established cause of PCOS, is proposed as a driving factor for the development of hyperandrogenemia and polycystic ovaries. Of the extraovarian factors, metabolic disorders (abnormal regulation of insulin and/or IGFs) and obesity may stimulate thecal hyperplasia, noting that approximately half of PCOS patients are obese [9]. Hypergonadism caused by dysregulation of LH secretion may also induce thecal hyperplasia, as LH stimulates theca cell proliferation [61-63]. Concurrently, intraovarian factors such as defects in steroidogenesis, regulation of the cell cycle, or ovulatory defects may result in thecal hyperplasia and hyperandrogenemia.
In regard to the mechanism of how hyperandrogenism may lead to ovulatory defect and therefore formation of follicular cyst in the ovary, it has been proposed that elevated testosterone disrupts the regulation of GnRH secretion [64]. In support of this, either GnRH agonists or LH treatment induces ovulation in PCOS patients. A few animal studies have shown that androgen suppresses progesterone receptor (PR) expression in the hypothalamus [65-67]. As progesterone down-regulates GnRH secretion by activating PR activity in the hypothalamus [68-70], androgen-mediated suppression of PR expression may ameliorate GnRH secretion, and therefore LH secretion (Fig. 3). This altered release of gonadotropins will lead to defects in follicle development, the development may arrest outright leading to failure to ovulate (anovulation) or be reduced leading to irregular ovulation (oligo-ovulation). The arrested follicle may thus form a cystic structure, leading to the polycystic ovarian phenotype and loss of fertility in PCOS patients.
Insulin resistance and obesity themselves are also known to negatively affect the secretion of pituitary hormones, and therefore follicular development [71-73]. It is not known whether altered steroidogenesis, pituitary hormone regulation or the defects in follicular development lead to an alteration of metabolism such as insulin resistance and obesity.
To identify the complex nature of this disease, suitable animal models are needed. Researchers during the past three decades have identified different animal models that mimic many of the features of PCOS in women (these are summarized in Table 1). These models have afforded valuable information into complex nature of PCOS. Currently, although a genetic component to PCOS has been identified, a specific "PCOS gene" has not, and therefore a specifically targeted gene deletion for PCOS as an animal model is not available. Therefore, the majority of PCOS models that are available to date rely upon external chemical treatments with steroids, steroid precursors or steroid receptor antagonist to achieve the pathology. Many of these models do not produce consistent results or cease to produce PCOS like symptoms once the treatment stops. Recently, Hill et al. [74] noted that their model for cardiovascular disease, the hypothalamic pro-opiomelanocortin (POMC) specific leptin-insulin double receptor knockout mouse exhibited some similar features to PCOS. Our recently developed theca specific Esr1 specific knockout mouse model reproduces the clinical pathologic features of PCOS in 100% of animals.
Prenatal androgen (PNA) treatment in sheep and monkeys results in multiple metabolic and reproductive abnormalities. In monkeys, daily subcutaneous injections of 15 mg of testosterone propionate for 40-80 days gestation are needed to induce the syndrome. In ewes, an injection of 100 mg of testosterone propionate twice a week for 60 days between days 30 and 90 of the 147-day pregnancy result in the ovarian abnormalities. In both models, the abnormalities mirror the symptoms found in women with PCOS. These models produce a long lasting effect in the female offspring mimicking many similar features of PCOS in humans. However, ewes and monkeys incur a large financial commitment for a long gestational period. However, it is noted that although PA monkeys exhibit hyperandrogenemia, the increases are not as extreme as in PCOS women 0.3-0.4 ng/mL (~50-100% elevation above normal) [76,90]; PCOS women, 0.5-0.7 ng/mL (~70-200% elevation above normal) [91-94] and that although anovulation observed in PNA monkeys, its prevalence is also significantly less than that of PCOS women (PA monkeys: ~40%; PCOS women ~90%) [90].
This model exploits the association of elevated androgen levels during puberty and PCOS. Immature rats (approximately 21 days old) are treated for 7-35 days with ~100 µg/day testosterone propionate or dihydrotestosterone. Similar to the PNA animal models, pre-pubertal androgen (PPA) animal models of PCOS utilize a unique window where administration of exogenous androgens results in permanent damage to the ovarian tissue and recapitulated the hallmark symptoms of PCOS in an animal model. PPA model shows many similar features to PCOS in women with the exception of the hallmark increase in basal LH levels [80,81]. This model is reliant on artificial hyperandrogenemia and therefore does not help identify abnormalities upstream of hyperandrogenemia.
Letrozole is an oral non-steroidal aromatase inhibitor. Inhibition of aromatase prevents the conversion of androgens to estrogens and therefore this model has similar features to the prepubertal androgen treatment [80,82]. Immature rats (approximately 21 days old) are treated for 7-35 days with 400 µg/day letrozole. Similarly to pre-pubertal androgen, this model is also reliant on artificial hyperandrogenemia and does not help identify abnormalities upstream of hyperandrogenemia.
Immature rats or mice (approximately 21-22 days old) are treated with daily s.c. DHEA injections (rats; 6 mg/100 g body weight, mice 6 mg/kg body weight) for 15-20 days. This dose of DHEA is sufficient to induce a hyperandrogenized state similar to that in PCOS women. This model is also reliant on artificial hyperandrogenemia and does not help identify abnormalities upstream of hyperandrogenemia.
Mature cycling female rats are treated daily with RU486 (20 mg/kg body weight) for more than 8 days starting on the day of estrus. These animals exhibit increased basal LH, polycystic ovaries, ovulation blockade and metabolic defects [87]. However, this model is reversible and symptoms decrease upon cessation of the antiprogestin treatment.
The immune system is now a well-recognized component of reproductive biology. Immune cells are involved in all aspects of normal reproductive function including ovulation, corpus luteal formation, uterine receptiveness and maintenance of pregnancy. The recently developed PCOS model developed by Chapman and colleagues [88] now provides evidence that aberrant immune function may play a role in the pathogenesis of PCOS and that PCOS may result from as autoimmune disease. This model exploits a 2-3 day window of neonatal period (5-7 days of age) when estradiol administration (20 µg/day) disrupts thymic maturation. As a consequence of this, increased vascular permeability in the thymus allows autoreactive T-cells to escape into the circulation. The ultimate effect of these "escapees" is a damage to tissues throughout the body including the ovaries. This model offers an abrupt change in the direction of PCOS research. The major deficiencies in this model are a lack of hyperandrogenemia and the fact that the loss of regulatory T-cell function would not only impact the ovary, but multiple other tissues resulting in pathologies not associated with PCOS. It will be interesting to follow the progress of this novel concept of autoimmune responses resulting in PCOS in women and if, PCOS women have deficiencies in regulatory T-cells.
Hill and her colleagues were interested in insulin resistance and the development of type II diabetes when they developed their Pomc-Cre, Leprflox/flox IRflox/flox mice, effectively removing both leptin and insulin receptors specifically from the POMC [74]. However, together with the anticipated glucose intolerance and insulin deficiency these mice suffer from hyperandrogenemia and polycystic ovaries. These two pathological conditions secure its eligibility as a model for the study of human PCOS.
The unraveling of a biological pathway for PCOS and assigning etiologies to this syndrome requires the development of a novel animal model that consistently present PCOS phenotypes. We therefore launched a project to develop a mouse model of PCOS that is genetically modified and thus stably displays PCOS phenotypes. In doing so, we utilized the underlying concept of a vicious cycle [95-97], where the abnormalities are reinforced. As a way to initiate this cycle, we targeted the ovarian steroidogenic pathway to induce a preference to androgen synthesis and therefore create hyperandrogenemia.
The ovary is comprised of follicles at different stages of development. During follicular growth, the oocyte becomes surrounded by the granulosa cells, a basement membrane forms, and layers of theca cells develop, surrounding the follicle. The two cell layers act cooperatively in the production of androgens and estrogens. Theca cells produce androgens that traverse the basement membrane to the granulosa cells, where aromatase converts the androgens to estrogens. The estrogens in turn negatively feedback to the theca cells causing a decrease of androgen production. This negative feedback action of estrogen is mediated by estrogen receptor 1 (Esr1; ERα) in the theca cells, the prime site of Esr1 expression in the ovary. Upon activation by the binding of estrogen, Esr1 suppresses the expression of Cyp17, a critical enzyme that catalyzes a rate-limiting step in androgen production (Fig. 4), resulting in the decrease of androgen production. Therefore, deleting Esr1 gene would be a logical choice for achieving sustained high levels of androgens because loss of Esr1 will exclude negative regulation of Cyp17 gene expression by estrogen. This will ultimately result in increased production of androgens. The deletion of Esr1 gene has to be, however, limited to theca cells because Esr1 is expressed in so many different tissues/organs including all of the tissues of the hypothalamic-pituitary-ovary-uterus axis [98,99]. Otherwise, the Esr1 deletion in non-theca cells would cause other defects, making it difficult to isolate the Esr1 deletion effect in the theca cells.
This goal was achieved by selectively knocking out Esr1in the steroidogenic theca cells while keeping the gene intact elsewhere. In principle, this approach would result in the increased expression of Cyp17 gene that encodes a late-limiting enzyme (17apha-hydroxylase) in androgen synthesis pathway, because deletion of Esr1 will relieve Cyp17 transcription from the well-established suppression of this gene by Esr1. We achieved this aim by first generating a mouse line that expresses Cre recombinase under the regulation of the Cyp17 promoter (Cyp17iCre) [100] and then breeding the mice successively with floxed Esr1 (Esr1flox) mice [89]. The offspring with the genotype Esr1flox/flox Cyp17iCre, as was expected, expresses higher level of Cyp17 mRNA expression in the ovary and maintains higher serum testosterone levels (1.5-2 folds over wild type littermates) (ref). The Esr1flox/flox Cyp17iCre mice display other phenotypic symptoms of PCOS patients: (i) irregular estrous cycles, (ii) an age-dependent decrease in ovulatory capacity and fertility defect and (iii) arrest of follicular development at the early antral stage (Figs. 5, 6). This novel animal model generated from the proof-of-principal experiment should bring a new opportunity for the study of PCOS as well as for the study of hyperandrogenemia itself.
In contrast to the seriousness of the PCOS in women's health, the pathogenesis of this disease is poorly understood. In recent years, efforts by multiple laboratories have led to the development of novel animal models for this complicated disorder. In the next few years, use of these animal models and those to be newly developed will undoubtedly accelerate our understanding on PCOS pathogenesis, and possibly lead to the development of new therapeutic protocols for treating and preventing PCOS in women.
Figures and Tables
Fig. 1
Ultrasound image of human polycystic ovary. Ovarian cysts are shown in the periphery of the ovary as dark circles.
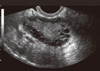
Fig. 3
Proposed dysregulation of HPO axis by elevated testosterone (T) level. The numbers represent sequence of events. (1) Positive estrogen (E2) feedback to E2 receptor (ER) in the hypothalamus to GnRH secretion and LH surge and progesterone (P) production in the ovary. Upon E2 stimulation, ER induces P receptor (PR) in the hypothalamus. (2) P induced suppression of GnRH secretion. (3) Hyperandrogenism-induced suppression of P receptor expression in the hypothalamus.
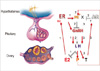
Fig. 4
Targeted modulation of ovarian steroidogenesis. A. CYP17 expression pattern in the 2-month old mouse ovary. CYP17-positve cells (theca cells; TC) are shown in brownish color. A follicle is made of oocyte (O), granulosa cells (GC) and theca cells (TC). B. ovarian steroidogenic pathway.
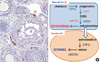
Fig. 5
Hyperandrogenic mouse ovary. 3-month old-mouse ovary stained with Hematoxylin and Eosin. Multiple early antral stage follicles and a cyst (C) are shown in the periphery of the ovary. Insert, age-matching wild type mouse ovary.
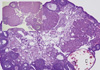
References
1. Broekmans FJ, Knauff EA, Valkenburg O, Laven JS, Eijkemans MJ, Fauser BC. PCOS according to the Rotterdam consensus criteria: Change in prevalence among WHO-II anovulation and association with metabolic factors. BJOG. 2006. 113:1210–1217.
2. Moghetti P, Toscano V. Treatment of hirsutism and acne in hyperandrogenism. Best Pract Res Clin Endocrinol Metab. 2006. 20:221–234.
3. Shum KW, Cullen DR, Messenger AG. Hair loss in women with hyperandrogenism: four cases responding to finasteride. J Am Acad Dermatol. 2002. 47:733–739.
4. Shah B, Parnell L, Milla S, Kessler M, David R. Endometrial thickness, uterine, and ovarian ultrasonographic features in adolescents with polycystic ovarian syndrome. J Pediatr Adolesc Gynecol. 2010. 23:146–152.
5. Mitwally MF, Casper RF. Aromatase inhibition reduces the dose of gonadotropin required for controlled ovarian hyperstimulation. J Soc Gynecol Investig. 2004. 11:406–415.
6. Hall JE, Taylor AE, Hayes FJ, Crowley WF Jr. Insights into hypothalamic-pituitary dysfunction in polycystic ovary syndrome. J Endocrinol Invest. 1998. 21:602–611.
7. Barnes RB. Pathophysiology of ovarian steroid secretion in polycystic ovary syndrome. Semin Reprod Endocrinol. 1997. 15:159–168.
8. Angioni S, Portoghese E, Milano F, Melis GB, Fulghesu AM. Diagnosis of metabolic disorders in women with polycystic ovary syndrome. Obstet Gynecol Surv. 2008. 63:796–802.
9. Hoeger KM. Obesity and lifestyle management in polycystic ovary syndrome. Clin Obstet Gynecol. 2007. 50:277–294.
10. Holte J, Bergh T, Berne C, Wide L, Lithell H. Restored insulin sensitivity but persistently increased early insulin secretion after weight loss in obese women with polycystic ovary syndrome. J Clin Endocrinol Metab. 1995. 80:2586–2593.
11. Giallauria F, Orio F, Palomba S, Lombardi G, Colao A, Vigorito C. Cardiovascular risk in women with polycystic ovary syndrome. J Cardiovasc Med (Hagerstown). 2008. 9:987–992.
12. Giallauria F, Palomba S, De Sio I, Maresca L, Vuolo L, Savastano S, Lombardi G, Colao A, Vigorito C, Orio F. Inflammatory markers and visceral fat are inversely associated with maximal oxygen consumption in women with polycystic ovary syndrome (PCOS). Clin Endocrinol (Oxf). 2009. 70:394–400.
13. Cascella T, Palomba S, De Sio I, Manguso F, Giallauria F, De Simone B, Tafuri D, Lombardi G, Colao A, Orio F. Visceral fat is associated with cardiovascular risk in women with polycystic ovary syndrome. Hum Reprod. 2008. 23:153–159.
14. Orio F, Giallauria F, Palomba S, Manguso F, Orio M, Tafuri D, Lombardi G, Carmina E, Colao A, Vigorito C. Metabolic and cardiopulmonary effects of detraining after a structured exercise training programme in young PCOS women. Clin Endocrinol (Oxf). 2008. 68:976–981.
15. Trakakis E, Balanika A, Baltas C, Loghis C, Simeonidis G, Vaggopoulos V, Papakonstantinou O, Gouliamos A, Salamalekis G, Kassanos D. Hemodynamic alterations and wall properties in large arteries of young, normotensive, and non-obese women with polycystic ovary syndrome. J Endocrinol Invest. 2008. 31:1001–1007.
16. Tremblay A, Boule N, Doucet E, Woods SC. Is the insulin resistance syndrome the price to be paid to achieve body weight stability? Int J Obes (Lond). 2005. 29:1295–1298.
17. Boule NG, Weisnagel SJ, Lakka TA, Tremblay A, Bergman RN, Rankinen T, Leon AS, Skinner JS, Wilmore JH, Rao DC, Bouchard C. Effects of exercise training on glucose homeostasis: the HERITAGE Family Study. Diabetes Care. 2005. 28:108–114.
18. Goodyear LJ, Hirshman MF, Knutson SM, Horton ED, Horton ES. Effect of exercise training on glucose homeostasis in normal and insulin-deficient diabetic rats. J Appl Physiol. 1988. 65:844–851.
19. Sigal RJ, Kenny GP, Wasserman DH, Castaneda-Sceppa C, White RD. Physical activity/exercise and type 2 diabetes: a consensus statement from the American Diabetes Association. Diabetes Care. 2006. 29:1433–1438.
20. Couzinet B, Pholsena M, Young J, Schaison G. The impact of a pure anti-androgen (flutamide) on LH, FSH, androgens and clinical status in idiopathic hirsutism. Clin Endocrinol (Oxf). 1993. 39:157–162.
21. Ibanez L, de Zegher F. Low-dose flutamide-metformin therapy for hyperinsulinemic hyperandrogenism in non-obese adolescents and women. Hum Reprod Update. 2006. 12:243–252.
22. Saha L, Kaur S, Saha PK. Pharmacotherapy of polycystic ovary syndrome-an update. Fundam Clin Pharmacol. 2011.
23. Studen KB, Sebestjen M, Pfeifer M, Prezelj J. Influence of spironolactone treatment on endothelial function in non-obese women with polycystic ovary syndrome. Eur J Endocrinol. 2011. 164:389–395.
24. Armanini D, Castello R, Scaroni C, Bonanni G, Faccini G, Pellati D, Bertoldo A, Fiore C, Moghetti P. Treatment of polycystic ovary syndrome with spironolactone plus licorice. Eur J Obstet Gynecol Reprod Biol. 2007. 131:61–67.
25. Christy NA, Franks AS, Cross LB. Spironolactone for hirsutism in polycystic ovary syndrome. Ann Pharmacother. 2005. 39:1517–1521.
26. Zulian E, Sartorato P, Benedini S, Baro G, Armanini D, Mantero F, Scaroni C. Spironolactone in the treatment of polycystic ovary syndrome: effects on clinical features, insulin sensitivity and lipid profile. J Endocrinol Invest. 2005. 28:49–53.
27. Archer JS, Chang RJ. Hirsutism and acne in polycystic ovary syndrome. Best Pract Res Clin Obstet Gynaecol. 2004. 18:737–754.
28. Farhi J, Homburg R, Lerner A, Ben-Rafael Z. The choice of treatment for anovulation associated with polycystic ovary syndrome following failure to conceive with clomiphene. Hum Reprod. 1993. 8:1367–1371.
29. Marantides D. Management of polycystic ovary syndrome. Nurse Pract. 1997. 22:34–38. 40–41.
30. Homburg R. Ovulation induction. Expert Opin Pharmacother. 2003. 4:1995–2004.
31. Urman B, Yakin K. Ovulatory disorders and infertility. J Reprod Med. 2006. 51:267–282.
32. Nader S. Ovulation induction in polycystic ovary syndrome. Minerva Ginecol. 2008. 60:53–61.
33. Vause TD, Cheung AP, Sierra S, Claman P, Graham J, Guillemin JA, Lapensee L, Stewart S, Wong BC. Ovulation induction in polycystic ovary syndrome. J Obstet Gynaecol Can. 2010. 32:495–502.
34. Hart R. PCOS and infertility. Panminerva Med. 2008. 50:305–314.
35. Pasquali R, Casimirri F, Vicennati V. Weight control and its beneficial effect on fertility in women with obesity and polycystic ovary syndrome. Hum Reprod. 1997. 12:Suppl 1. 82–87.
36. Moran LJ, Norman RJ. The obese patient with infertility: a practical approach to diagnosis and treatment. Nutr Clin Care. 2002. 5:290–297.
37. Duggal PS, Ryan NK, Van der Hoek KH, Ritter LJ, Armstrong DT, Magoffin DA, Norman RJ. Effects of leptin administration and feed restriction on thecal leucocytes in the preovulatory rat ovary and the effects of leptin on meiotic maturation, granulosa cell proliferation, steroid hormone and PGE2 release in cultured rat ovarian follicles. Reproduction. 2002. 123:891–898.
38. Pasquali R, Pelusi C, Genghini S, Cacciari M, Gambineri A. Obesity and reproductive disorders in women. Hum Reprod Update. 2003. 9:359–372.
39. Pasquali R, Gambineri A. Role of changes in dietary habits in polycystic ovary syndrome. Reprod Biomed Online. 2004. 8:431–439.
40. Hoeger KM. Role of lifestyle modification in the management of polycystic ovary syndrome. Best Pract Res Clin Endocrinol Metab. 2006. 20:293–310.
41. Kolodziejczyk B, Duleba AJ, Spaczynski RZ, Pawelczyk L. Metformin therapy decreases hyperandrogenism and hyperinsulinemia in women with polycystic ovary syndrome. Fertil Steril. 2000. 73:1149–1154.
42. Awartani KA, Cheung AP. Metformin and polycystic ovary syndrome: a literature review. J Obstet Gynaecol Can. 2002. 24:393–401.
43. Barbieri RL. Metformin for the treatment of polycystic ovary syndrome. Obstet Gynecol. 2003. 101:785–793.
44. Stadtmauer L, Oehninger S. Management of infertility in women with polycystic ovary syndrome: a practical guide. Treat Endocrinol. 2005. 4:279–292.
45. Mathur R, Alexander CJ, Yano J, Trivax B, Azziz R. Use of metformin in polycystic ovary syndrome. Am J Obstet Gynecol. 2008. 199:596–609.
46. Harwood K, Vuguin P, DiMartino-Nardi J. Current approaches to the diagnosis and treatment of polycystic ovarian syndrome in youth. Horm Res. 2007. 68:209–217.
47. Boudhraa K, Jellouli MA, Amri M, Farhat M, Torkhani F, Gara MF. [Indication of metformin in the management of hormonal dysfunction secondary to polycystic ovarian syndrome: prospective comparative study of 63 cases]. Tunis Med. 2010. 88:335–340.
48. Brown J, Farquhar C, Beck J, Boothroyd C, Hughes E. Clomiphene and anti-oestrogens for ovulation induction in PCOS. Cochrane Database Syst Rev. 2009. CD002249.
49. Casper RF. Aromatase inhibitors in ovarian stimulation. J Steroid Biochem Mol Biol. 2007. 106:71–75.
50. Eckmann KR, Kockler DR. Aromatase inhibitors for ovulation and pregnancy in polycystic ovary syndrome. Ann Pharmacother. 2009. 43:1338–1346.
51. Hughes E, Brown J, Collins JJ, Vanderkerchove P. Clomiphene citrate for unexplained subfertility in women. Cochrane Database Syst Rev. 2010. CD000057.
52. Jansen RP, Handelsman DJ, Boylan LM, Conway A, Shearman RP, Fraser IS, Anderson JC. Pulsatile intravenous gonadotropin-releasing hormone for ovulation-induction in infertile women. II. Analysis of follicular and luteal phase responses. Fertil Steril. 1987. 48:39–44.
53. Larsen T, Larsen JF, Schioler V, Bostofte E, Felding C. Comparison of urinary human follicle-stimulating hormone and human menopausal gonadotropin for ovarian stimulation in polycystic ovarian syndrome. Fertil Steril. 1990. 53:426–431.
54. Nakamura Y, Yamada H, Yoshida K, Manno T, Ubukata Y, Suzuki M, Yoshimura Y. Induction of ovulation with pulsatile subcutaneous administration of human menopausal gonadotropin in patients with polycystic ovary syndrome. Horm Res. 1990. 33:Suppl 2. 43–48.
55. Cohen J. Laparoscopic procedures for treatment of infertility related to polycystic ovarian syndrome. Hum Reprod Update. 1996. 2:337–344.
56. Donesky BW, Adashi EY. Surgical ovulation induction: the role of ovarian diathermy in polycystic ovary syndrome. Baillieres Clin Endocrinol Metab. 1996. 10:293–309.
57. Liguori G, Tolino A, Moccia G, Scognamiglio G, Nappi C. Laparoscopic ovarian treatment in infertile patients with polycystic ovarian syndrome (PCOS): endocrine changes and clinical outcome. Gynecol Endocrinol. 1996. 10:257–264.
58. Tulandi T, Watkin K, Murray C, Mathur S. Reproductive Performance and Three-Dimension-Ultrasound Volume Determination of Polycystic Ovaries after Laparoscopic Ovarian Drilling. J Am Assoc Gynecol Laparosc. 1996. 3:S50–S51.
59. Farquhar C, Vandekerckhove P, Arnot M, Lilford R. Laparoscopic "drilling" by diathermy or laser for ovulation induction in anovulatory polycystic ovary syndrome. Cochrane Database Syst Rev. 2000. CD001122.
60. Farquhar C, Lilford RJ, Marjoribanks J, Vandekerckhove P. Laparoscopic 'drilling' by diathermy or laser for ovulation induction in anovulatory polycystic ovary syndrome. Cochrane Database Syst Rev. 2007. CD001122.
61. Onagbesan OM, Peddie MJ, Williams J. Regulation of cell proliferation and estrogen synthesis by ovine LH, IGF-I, and EGF in theca interstitial cells of the domestic hen cultured in defined media. Gen Comp Endocrinol. 1994. 94:261–272.
62. Jia Y, Lin J, Zeng W, Zhang C. Effect of prostaglandin on luteinizing hormone-stimulated proliferation of theca externa cells from chicken prehierarchical follicles. Prostaglandins Other Lipid Mediat. 2010. 92:77–84.
63. Palaniappan M, Menon KM. Human chorionic gonadotropin stimulates theca-interstitial cell proliferation and cell cycle regulatory proteins by a cAMP-dependent activation of AKT/mTORC1 signaling pathway. Mol Endocrinol. 2010. 24:1782–1793.
64. Kalro BN, Loucks TL, Berga SL. Neuromodulation in polycystic ovary syndrome. Obstet Gynecol Clin North Am. 2001. 28:35–62.
65. Ozawa H. Steroid Hormones, their receptors and neuroendocrine system. J Nihon Med Sch. 2005. 72:316–325.
66. Lea RW, Clark JA, Tsutsui K. Changes in central steroid receptor expression, steroid synthesis, and dopaminergic activity related to the reproductive cycle of the ring dove. Microsc Res Tech. 2001. 55:12–26.
67. Wennstrom KL, Crews D. Effect of long-term castration and long-term androgen treatment on sexually dimorphic estrogen-inducible progesterone receptor mRNA levels in the ventromedial hypothalamus of whiptail lizards. Horm Behav. 1998. 34:11–16.
68. Pastor CL, Griffin-Korf ML, Aloi JA, Evans WS, Marshall JC. Polycystic ovary syndrome: evidence for reduced sensitivity of the gonadotropin-releasing hormone pulse generator to inhibition by estradiol and progesterone. J Clin Endocrinol Metab. 1998. 83:582–590.
69. Goodman RL. Neural systems mediating the negative feedback actions of estradiol and progesterone in the ewe. Acta Neurobiol Exp (Wars). 1996. 56:727–741.
70. Heikinheimo O, Gordon K, Williams RF, Hodgen GD. Inhibition of ovulation by progestin analogs (agonists vs antagonists): preliminary evidence for different sites and mechanisms of actions. Contraception. 1996. 53:55–64.
71. Chang RJ. The reproductive phenotype in polycystic ovary syndrome. Nat Clin Pract Endocrinol Metab. 2007. 3:688–695.
72. Franco C, Bengtsson BA, Johannsson G. Visceral obesity and the role of the somatotropic axis in the development of metabolic complications. Growth Horm IGF Res. 2001. 11:Suppl A. S97–S102.
73. Dunaif A. Insulin resistance and the polycystic ovary syndrome: mechanism and implications for pathogenesis. Endocr Rev. 1997. 18:774–800.
74. Hill JW, Elias CF, Fukuda M, Williams KW, Berglund ED, Holland WL, Cho YR, Chuang JC, Xu Y, Choi M, Lauzon D, Lee CE, Coppari R, Richardson JA, Zigman JM, Chua S, Scherer PE, Lowell BB, Bruning JC, Elmquist JK. Direct insulin and leptin action on pro-opiomelanocortin neurons is required for normal glucose homeostasis and fertility. Cell Metab. 2010. 11:286–297.
75. Abbott DH, Barnett DK, Levine JE, Padmanabhan V, Dumesic DA, Jacoris S, Tarantal AF. Endocrine antecedents of polycystic ovary syndrome in fetal and infant prenatally androgenized female rhesus monkeys. Biol Reprod. 2008. 79:154–163.
76. Abbott DH, Dumesic DA, Eisner JR, Colman RJ, Kemnitz JW. Insights into the development of polycystic ovary syndrome (PCOS) from studies of prenatally androgenized female rhesus monkeys. Trends Endocrinol Metab. 1998. 9:62–67.
77. Abbott DH, Bruns CR, Barnett DK, Dunaif A, Goodfriend TL, Dumesic DA, Tarantal AF. Experimentally induced gestational androgen excess disrupts glucoregulation in rhesus monkey dams and their female offspring. Am J Physiol Endocrinol Metab. 2010. 299:E741–E751.
78. Dumesic DA, Abbott DH, Eisner JR, Goy RW. Prenatal exposure of female rhesus monkeys to testosterone propionate increases serum luteinizing hormone levels in adulthood. Fertil Steril. 1997. 67:155–163.
79. Forsdike RA, Hardy K, Bull L, Stark J, Webber LJ, Stubbs S, Robinson JE, Franks S. Disordered follicle development in ovaries of prenatally androgenized ewes. J Endocrinol. 2007. 192:421–428.
80. Manneras L, Cajander S, Holmang A, Seleskovic Z, Lystig T, Lonn M, Stener-Victorin E. A new rat model exhibiting both ovarian and metabolic characteristics of polycystic ovary syndrome. Endocrinology. 2007. 148:3781–3791.
81. Beloosesky R, Gold R, Almog B, Sasson R, Dantes A, Land-Bracha A, Hirsh L, Itskovitz-Eldor J, Lessing JB, Homburg R, Amsterdam A. Induction of polycystic ovary by testosterone in immature female rats: Modulation of apoptosis and attenuation of glucose/insulin ratio. Int J Mol Med. 2004. 14:207–215.
82. Kafali H, Iriadam M, Ozardali I, Demir N. Letrozole-induced polycystic ovaries in the rat: a new model for cystic ovarian disease. Arch Med Res. 2004. 35:103–108.
83. Lee GY, Croop JM, Anderson E. Multidrug resistance gene expression correlates with progesterone production in dehydroepiandrosterone-induced polycystic and equine chorionic gonadotropin-stimulated ovaries of prepubertal rats. Biol Reprod. 1998. 58:330–337.
84. Lee MT, Anderson E, Lee GY. Changes in ovarian morphology and serum hormones in the rat after treatment with dehydroepiandrosterone. Anat Rec. 1991. 231:185–192.
85. Motta AB. Dehydroepiandrosterone to induce murine models for the study of polycystic ovary syndrome. J Steroid Biochem Mol Biol. 2010. 119:105–111.
86. Zhang HY, Zhu FF, Xiong J, Shi XB, Fu SX. Characteristics of different phenotypes of polycystic ovary syndrome based on the Rotterdam criteria in a large-scale Chinese population. BJOG. 2009. 116:1633–1639.
87. Priyadarshani A. Relevance of an opioid, noscapine in reducing cystogeneses in rat experimental model of polycystic ovary syndrome. J Endocrinol Invest. 2009. 32:837–843.
88. Chapman JC, Min SH, Freeh SM, Michael SD. The estrogen-injected female mouse: new insight into the etiology of PCOS. Reprod Biol Endocrinol. 2009. 7:47.
89. Lee S, Kang DW, Hudgins-Spivey S, Krust A, Lee EY, Koo Y, Cheon Y, Gye MC, Chambon P, Ko C. Theca-specific estrogen receptor-alpha knockout mice lose fertility prematurely. Endocrinology. 2009. 150:3855–3862.
90. Abbott DH, Tarantal AF, Dumesic DA. Fetal, infant, adolescent and adult phenotypes of polycystic ovary syndrome in prenatally androgenized female rhesus monkeys. Am J Primatol. 2009. 71:776–784.
91. DeVane GW, Czekala NM, Judd HL, Yen SS. Circulating gonadotropins, estrogens, and androgens in polycystic ovarian disease. Am J Obstet Gynecol. 1975. 121:496–500.
92. Christian RC, Dumesic DA, Behrenbeck T, Oberg AL, Sheedy PF 2nd, Fitzpatrick LA. Prevalence and predictors of coronary artery calcification in women with polycystic ovary syndrome. J Clin Endocrinol Metab. 2003. 88:2562–2568.
93. Phy JL, Conover CA, Abbott DH, Zschunke MA, Walker DL, Session DR, Tummon IS, Thornhill AR, Lesnick TG, Dumesic DA. Insulin and messenger ribonucleic acid expression of insulin receptor isoforms in ovarian follicles from nonhirsute ovulatory women and polycystic ovary syndrome patients. J Clin Endocrinol Metab. 2004. 89:3561–3566.
94. Foong SC, Abbott DH, Zschunke MA, Lesnick TG, Phy JL, Dumesic DA. Follicle luteinization in hyperandrogenic follicles of polycystic ovary syndrome patients undergoing gonadotropin therapy for in vitro fertilization. J Clin Endocrinol Metab. 2006. 91:2327–2333.
95. Vrbikova J, Hainer V. Obesity and polycystic ovary syndrome. Obes Facts. 2009. 2:26–35.
96. Sozen I, Arici A. Hyperinsulinism and its interaction with hyperandrogenism in polycystic ovary syndrome. Obstet Gynecol Surv. 2000. 55:321–328.
97. Kopelman PG. Hormones and obesity. Baillieres Clin Endocrinol Metab. 1994. 8:549–575.
98. Couse JF, Lindzey J, Grandien K, Gustafsson JA, Korach KS. Tissue distribution and quantitative analysis of estrogen receptor-alpha (ERalpha) and estrogen receptor-beta (ERbeta) messenger ribonucleic acid in the wild-type and ERalpha-knockout mouse. Endocrinology. 1997. 138:4613–4621.
99. Hatoya S, Torii R, Kumagai D, Sugiura K, Kawate N, Tamada H, Sawada T, Inaba T. Expression of estrogen receptor alpha and beta genes in the mediobasal hypothalamus, pituitary and ovary during the canine estrous cycle. Neurosci Lett. 2003. 347:131–135.
100. Bridges PJ, Koo Y, Kang DW, Hudgins-Spivey S, Lan ZJ, Xu X, DeMayo F, Cooney A, Ko C. Generation of Cyp17iCre transgenic mice and their application to conditionally delete estrogen receptor alpha (Esr1) from the ovary and testis. Genesis. 2008. 46:499–505.