Abstract
Background
Insulin-mediated glucose uptake in insulin target tissues is correlated with interstitial insulin concentration, rather than plasma insulin concentration. Therefore, insulin delivery to the interstitium of target tissues is very important, and the endothelium may also play an important role in the development of insulin resistance.
Methods
After treating bovine aortic endothelial cells with angiotensin II (ATII), we observed the changes in insulin binding capacity and the amounts of insulin receptor (IR) on the cell membranes and in the cytosol.
Results
After treatment of 10-7M ATII, insulin binding was decreased progressively, up to 60% at 60 minutes (P<0.05). ATII receptor blocker (eprosartan) dose dependently improved the insulin binding capacity which was reduced by ATII (P<0.05). At 200 µM, eprosartan fully restored insulin binding capacity, althogh it resulted in only a 20% to 30% restoration at the therapeutic concentration. ATII did not affect the total amount of IR, but it did reduce the amount of IR on the plasma membrane and increased that in the cytosol.
Conclusion
ATII decreased the insulin binding capacity of the tested cells. ATII did not affect the total amount of IR but did decrease the amount of IR on the plasma membrane. Our data indicate that ATII decreases insulin binding by translocating IR from the plasma membrane to the cytosol. The binding of insulin to IR is important for insulin-induced vasodilation and transendothelial insulin transport. Therefore, ATII may cause insulin resistance through this endothelium-based mechanism.
The actions of insulin on glucose metabolism are to increase glucose uptake in peripheral insulin target tissues (skeletal muscle and adipose tissue) and to inhibit glucose production by the liver. Before insulin can act on target tissues, it has to be secreted from pancreatic β-cells and transported from the blood across the endothelium to the interstitia of target tissues. Many studies have demonstrated that insulin-mediated glucose disposal increases progressively in a time- and dose-dependent manner; however, this transport is strongly correlated with lymphatic than plasma insulin concentration [1]. Thus, transcapillary insulin transport may be a rate-limiting step in insulin action, and impairment of the processes involved may contribute to insulin resistance.
It is believed that two steps are needed to deliver insulin to target tissues. The first step is the relaxation of terminal arterioles within target organs (capillary recruitment), and the second is the insulin transport to the interstitium across the endothelium. It is well known that insulin increases its own delivery to target organs by increasing nitric oxide (NO) production, due to activation of endothelial NO synthase (eNOS) through the PI3 kinase-Akt signal pathway [2]. However, the mechanism for insulin transport across the endothelium remains unclear. Receptor mediated transcytosis [3-5] and passive diffusion [6,7] have been suggested as possible mechanisms. It is generally accepted that insulin is transported by receptor-mediated transcytosis, and that insulin receptor (IR) and insulin-like growth factor-1 receptor (IGF-1R) play an important role in this process.
The purpose of this study is to examine whether angiotensin II (ATII), which is a known substance involved in the increase in insulin resistance, inhibits insulin binding on bovine aortic endothelial cells (BAECs). Insulin binding is considered as an initially required process for insulin signaling and receptor-mediated transcytosis on endothelium. Therefore, inhibition of insulin binding on endothelial cells may reduce the delivery of insulin to target tissues, causing insulin resistance.
Bovine insulin, ATII and eprosartan were purchased from Sigma-Aldrich (St. Louis, MO, USA). Anti-insulin receptor beta subunit (Anti-IRβ) was from Santa Cruz Biotechnology Inc. (Santa Cruz, CA, USA).
The bovine aortic endothelial cells (Lonza, Walkersville, MD, USA) were cultured in DMEM medium supplemented with 10% fetal bovine serum (FBS), 100 units/mL penicillin G and 0.1 mg/mL streptomycin. Cells were maintained at 37℃ in humidified air and 5% CO2. The medium was replaced three times a week. The cells were separated 5 to 10 times for use in the present experiments.
Insulin binding was studied using a modification of the methods of Kaiser et al. [8]. The cells were plated into dishes with DMEM containing 0.5% FBS for 24 hours and incubated at 37℃ with Tris-Hepes binding buffer (50 mM Tris, 50 mM Hepes, 10 mM MgC12, 2 mM EDTA, 10 mM dextrose, 10 mM CaC12, 50 mM NaCl, 5 mM KCl, 0.5% crystalline BSA, pH 7.8) and [125I]-insulin (0.5 µCi/well) for 0, 10, 20, 30, 60, and 90 minutes. To investigate whether insulin binding was affected by ATII, we added 10-7M ATII with or without ATII receptor blocker (eprosartan, 0.02, 0.2, 2, 20, and 200 µM) to the cells. After incubation, the supernatant was removed and the cells were immediately washed three times with 2 mL ice-cold phosphate-buffered saline (PBS). The cells were then treated with 1 mL 0.1 N NaOH to detach them from the dishes. The radioactivity of the insulin binding to the cells was counted in a Gamma counter (Packard Bioscience Company, Downers Grove, IL, USA). The insulin binding capacity was calculated using the following formula.
Insulin binding capacity=(radioactivity in cells/total radioactivity)×100
The cells were lysed with ice-cold lysis buffer (150 mM NaCl, 50 mM Tris-HCl, pH 7.4, 2 mM EDTA, 1% NP-40, 10 mM NaF, 1 mM Na3VO4, 10 mM sodium pyrophosphate, 1 mM PMSF, 10 mg/mL aprotinin, 10 mg/mL leupeptin, and 0.1 mg/mL soybean inhibitor) and centrifuged at 14,000 rpm for 10 minutes at 4℃. Extracted proteins were denatured at 95℃ for 3 minutes in 2× SDS sample buffer containing 125 mM Tris, 4% SDS, 20% glycerol, 10% 2-mercaptoethanol and 0.2% bromophenol blue. The proteins (50 µg) were fractionated in 10% SDS-PAGE at 20 mA for 2 to 5 hours and were transferred to nitrocellulose membrane in transfer buffer (25 mM Tris, pH 8.3, 192 mM glycine, 20% methanol) at 250 mA for 1 hour at 4℃. Following transfer, the membranes were blocked with 5% fat-free dry milk for 1 hour in Tris-buffered saline (TBS; 25 mM Tris-HCl, pH 7.6, and 150 mM NaCl) containing 0.1% Tween 20 (TBS-T). Primary antibodies for IRβ were used at 1:1,000 dilutions in TBS-T containing 5% fat-free dry milk at 4℃. Membrane were exposed to secondary antibodies conjugated with horseradish peroxidase (Calbiochem, San Diego, CA, USA) diluted 1:1,000 in 5% fat-free dry milk in TBS-T at room temperature for 1 hour. Staining was detected with an enhanced chemiluminescence kit (Santa Cruz Biotechnology Inc.).
As shown in Fig. 1, after treatment with insulin, the insulin binding capacity was increased over time and reached a peak at 20 minutes and a plateau at 30 minutes. When adding 10-7M ATII, insulin binding was decreased progressively, up to 60% at 60 minutes. These data show that ATII inhibits insulin binding on bovine aortic endothelial cell. To determine whether ARB restores this effect, we pretreated the cells with eprosartan (0.02, 0.2, 2, 20, and 200 µM) for 30 minutes, then added [125I]-insulin (0.5 µCi/well) and ATII (10-7M) for 60 minutes. As shown in Fig. 2, eprosartan dose dependently improved the insulin binding capacity which was reduced by ATII. At 200 µM, eprosartan fully restored insulin binding capacity, although only a 20% to 30% restoration was observed at the therapeutic concentration.
To determine whether insulin and ATII affect the amount of IR, we treated bovine aortic endothelial cells with 10-7M insulin and 10-7M ATII. As shown in Fig. 3A, neither insulin nor ATII affected the total amounts of IRβ. Instead, ATII reduced the amount of IRβ on the cell membrane and increased that in the cytosol. These data suggest that ATII translocates insulin receptor proteins from the cell membrane to the cytosol and thereby inhibits insulin binding with IR on endothelial cells. To determine whether this effect of ATII is offset by ARB, we pretreated cells with 200 µM eprosartan for 30 minutes and added 10-7M insulin and 10-7M ATII. As shown in Fig. 3B, eprosartan inhibited the translocation of IRβ by ATII.
Insulin-mediated glucose uptake is correlated with interstitial insulin concentration in insulin target tissues rather than with plasma concentration [1]. In human studies, interstitial insulin concentrations estimated through microdialysis are reported to be only 40% to 60% of simultaneous measured plasma concentrations and the time kinetics of insulin are slower in the interstitial fluid than they are in plasma [9-11]. Several studies have suggested that the maximal interstitial insulin concentration is similar in the normal and insulin-resistant states, but transcapillary insulin transport is delayed in the insulin-resistant state [10,12]. Therefore, transcapillary insulin transport may be important for insulin action, and the endothelium may play an important barrier role in the development of insulin resistance.
It is thought that insulin delivery to target tissues is achieved in a two-step process. The first step is the relaxation of terminal arterioles within target organs (capillary recruitment), and the second is insulin transport to the interstitium across the endothelium.
It is well known that insulin increases its own delivery to target organs by increasing the production of NO in endothelium. When insulin on endothelium binds insulin receptor, eNOS is activated via phosphorylation at Ser1177 through the PI3kinase-Akt signal pathway, resulting in increased NO production [2]. NO diffuses into the adjacent vascular smooth muscle cells and causes vasodilation of terminal arterioles and capillary recruitment [2].
Endothelia from different tissues have various structures and permeability [13]. The endothelium within skeletal muscle, which contributes to most of the peripheral glucose uptake, is relative tight and makes paracellular transport of most macromolecules (including insulin) difficult [13]. The mechanism for insulin transport across the endothelium in skeletal muscle is not yet known. Some in vitro studies have suggested that insulin is transported by receptor mediated transcytosis [3-5]. On the other hand, in vivo studies suggest that insulin is transported by passive diffusion because this process does not appear to be saturable [6,7]. But now, it is generally accepted that insulin is transported by receptor-mediated transcytosis (in relation to the formation of caveolae), and that IR and IGF-1R play an important role in this process.
In this study, we tried to investigate whether ATII affects insulin binding on endothelial cells. Because it is thought that insulin binding on the endothelium is an initial step in eNOS activation via insulin signaling and receptor-mediated insulin transcytosis, the impairment of insulin binding can affect transcapillary insulin transport and cause insulin resistance. As shown in our results, ATII decreased the insulin binding capacity on endothelium. We observed that treatment with ATII did not affect the total amount of IR, although the amount of IR on the cell membrane was decreased and that in the cytosol was increased. Various possibilities may be considered to explain these results, but the data indicate that ATII decreases insulin binding by decreasing the amount of IR on the cell membrane due to translocation of IR to the cytosol. In addition, this study showed that ARB improved the insulin binding capacity which was reduced by ATII and inhibited the translocation of IR by ATII. In conclusion, we think that ATII may cause insulin resistance through inhibiting insulin and insulin receptor binding on the endothelium, which may be another insulin target site which can cause insulin resistance through the regulation of transcapillary insulin transport. In addition, ARB may help to improve insulin resistance on the endothelium.
There are several limitations in our study. First, we can not tell whether eNOS activation or receptor-mediated transcytosis is more affected by a reduction in insulin binding, eNOS. Several studies have suggested that ATII decreases the bioavailability and production of NO in endothelium via ROS production and inhibition of the PI3 kinase-Akt signal pathway [14-16]. However, no study has found that ATII directly affects receptor-mediated transcytosis. Therefore, further studies are needed to determine if this is true. Because IGF-1R is an additional insulin binding receptor (it is more abundant in the endothelium than is IR [17]) and caveolin-1 and c-Src play an important role in the formation of caveolae, it will be necessary to observe the changes in the total amounts of IFG-1R and caveolin-1 and the amounts on the plasma membrane after treatment with ATII. Second, we used bovine aortic endothelial cells (BAECs), if we had used human capillary endothelial cell instead of BAECs, the result would have been more reliable. Third, we used ATII as the cause of insulin resistance. To verify that the endothelium is an insulin target site which causes insulin resistance, it is necessary to examine whether other substances such as tumor necrosis factor (TNF)-α, interleukin (IL)-1, IL-6, free fatty acid, etc., which are believed to increase insulin resistance, impair transcapillary insulin transport.
Figures and Tables
Fig. 1
The effect of angiotensin II (ATII) on insulin binding. Bovine aortic endothelial cells were pretreated with 10-7M ATII, then subjected to [125I]-insulin (0.5 µCi/well). Binding assays were performed as described in Methods. aP<0.05; control vs. ATII.
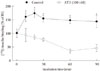
Fig. 2
The effect of angiotensin receptor blocker (eprosartan) on insulin binding. Bovine aortic endothelial cells were preincubated with eprosartan (0.02, 0.2, 2, 20, and 200 µM) for 30 minutes, and then [125I]-insulin (0.5 µCi/well) and angiotensin II (ATII) (10-7M) were added for 60 minutes. Binding assays were performed as described in Methods. aP<0.05; ATII vs. eprosartan.
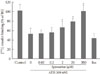
Fig. 3
The changes in the amounts of insulin receptor beta subunit (IRβ) on the plasma membrane and in the cytosol due to treatment with insulin and angiotensin II (ATII). (A) Bovine aortic endothelial cells were treated with 10-7M insulin and 10-7M ATII. (B) Bovine aortic endothelial cells were preincubated with eprosartan (200 µM) for 30 minutes, then 10-7M insulin and 10-7M ATII were added. The amounts of IRβ were measured using Western blotting. Membrane and cytosol proteins were separated and extracted using the MEM-PER mammalian membrane protein extraction kit.

References
1. Yang YJ, Hope ID, Ader M, Bergman RN. Insulin transport across capillaries is rate limiting for insulin action in dogs. J Clin Invest. 1989. 84:1620–1628.
2. Dimmeler S, Fleming I, Fisslthaler B, Hermann C, Busse R, Zeiher AM. Activation of nitric oxide synthase in endothelial cells by Akt-dependent phosphorylation. Nature. 1999. 399:601–605.
3. King GL, Johnson SM. Receptor-mediated transport of insulin across endothelial cells. Science. 1985. 227:1583–1586.
4. Eggleston EM, Jahn LA, Barrett EJ. Hyperinsulinemia rapidly increases human muscle microvascular perfusion but fails to increase muscle insulin clearance: evidence that a saturable process mediates muscle insulin uptake. Diabetes. 2007. 56:2958–2963.
5. Wang H, Liu Z, Li G, Barrett EJ. The vascular endothelial cell mediates insulin transport into skeletal muscle. Am J Physiol Endocrinol Metab. 2006. 291:E323–E332.
6. Steil GM, Ader M, Moore DM, Rebrin K, Bergman RN. Transendothelial insulin transport is not saturable in vivo. No evidence for a receptor-mediated process. J Clin Invest. 1996. 97:1497–1503.
7. Vicent D, Ilany J, Kondo T, Naruse K, Fisher SJ, Kisanuki YY, Bursell S, Yanagisawa M, King GL, Kahn CR. The role of endothelial insulin signaling in the regulation of vascular tone and insulin resistance. J Clin Invest. 2003. 111:1373–1380.
8. Kaiser N, Vlodavsky I, Tur-Sinai A, Fuks Z, Cerasi E. Binding, internalization, and degradation of insulin in vascular endothelial cells. Diabetes. 1982. 31:1077–1083.
9. Sjostrand M, Holmang A, Lonnroth P. Measurement of interstitial insulin in human muscle. Am J Physiol. 1999. 276(1 Pt 1):E151–E154.
10. Sjostrand M, Gudbjornsdottir S, Holmang A, Lonn L, Strindberg L, Lonnroth P. Delayed transcapillary transport of insulin to muscle interstitial fluid in obese subjects. Diabetes. 2002. 51:2742–2748.
11. Herkner H, Klein N, Joukhadar C, Lackner E, Langenberger H, Frossard M, Bieglmayer C, Wagner O, Roden M, Muller M. Transcapillary insulin transfer in human skeletal muscle. Eur J Clin Invest. 2003. 33:141–146.
12. Wascher TC, Wolkart G, Russell JC, Brunner F. Delayed insulin transport across endothelium in insulin-resistant JCR:LA-cp rats. Diabetes. 2000. 49:803–809.
13. Simionescu M, Gafencu A, Antohe F. Transcytosis of plasma macromolecules in endothelial cells: a cell biological survey. Microsc Res Tech. 2002. 57:269–288.
14. Griendling KK, FitzGerald GA. Oxidative stress and cardiovascular injury. Part I: basic mechanisms and in vivo monitoring of ROS. Circulation. 2003. 108:1912–1916.
15. Imanishi T, Kobayashi K, Kuroi A, Mochizuki S, Goto M, Yoshida K, Akasaka T. Effects of angiotensin II on NO bioavailability evaluated using a catheter-type NO sensor. Hypertension. 2006. 48:1058–1065.
16. Andreozzi F, Laratta E, Sciacqua A, Perticone F, Sesti G. Angiotensin II impairs the insulin signaling pathway promoting production of nitric oxide by inducing phosphorylation of insulin receptor substrate-1 on Ser312 and Ser616 in human umbilical vein endothelial cells. Circ Res. 2004. 94:1211–1218.
17. Chisalita SI, Arnqvist HJ. Insulin-like growth factor I receptors are more abundant than insulin receptors in human micro- and macrovascular endothelial cells. Am J Physiol Endocrinol Metab. 2004. 286:E896–E901.