Abstract
Background
A limitation in the number of insulin-producing pancreatic beta-cells is a special feature of diabetes. The identification of alternative sources for the induction of insulin-producing surrogate beta-cells is a matter of profound importance. PDX-1/VP16, BETA2/NeuroD, and MafA overexpression have been shown to influence the differentiation and proliferation of pancreatic stem cells. However, few studies have been conducted using adult animal pancreatic stem cells.
Methods
Adult pig pancreatic cells were prepared from the non-endocrine fraction of adult pig pancreata. Porcine neonatal pancreas cell clusters (NPCCs) were prepared from neonatal pigs aged 1-2 days. The dispersed pancreatic cells were infected with PDX-1/VP16, BETA2/NeuroD, and MafA adenoviruses. After infection, these cells were transplanted under the kidney capsules of normoglycemic nude mice.
Results
The adenovirus-mediated overexpression of PDX-1, BETA2/NeuroD and MafA induced insulin gene expression in NPCCs, but not in adult pig pancreatic cells. Immunocytochemistry revealed that the number of insulin-positive cells in NPCCs and adult pig pancreatic cells was approximately 2.6- and 1.1-fold greater than those in the green fluorescent protein control group, respectively. At four weeks after transplantation, the relative volume of insulin-positive cells in the grafts increased in the NPCCs, but not in the adult porcine pancreatic cells.
Conclusion
These data indicate that PDX-1, BETA2/NeuroD, and MafA facilitate the beta-cell differentiation of NPCCs, but not adult pig pancreatic cells. Therefore PDX-1, BETA2/NeuroD, and MafA-induced NPCCs can be considered good sources for the induction of pancreatic beta-cells, and may also have some utility in the treatment of diabetes.
Diabetes mellitus is characterized by chronic hyperglycemia, which results in many problems such as cerebrovascular disease, coronary artery disease, blindness, chronic kidney disease, and the amputation of lower extremities. To date, however, it is not a curable disease and intensified insulin treatment, which can improve glycemic control and reduce diabetic complications, may result in severe hypoglycemia and is technically inconvenient [1]. Islet transplantation is one of the most promising modalities to cure diabetes, as it is easy to apply to patients [2]; however, the absolute shortage of donor pancreas is the major limitation [3]. In order to solve this problem, stem cell differentiation can be used as an alternative to islet cell transplantation. Pancreatic ductal cells are adult stem cells that have been shown to differentiate into beta-cells by treatment with factors associated with proliferation and differentiation with subsequent transplantation into diabetic animal models [4]. Most studies have been conducted using fetal or neonatal pancreatic tissue, which has a good capacity for proliferation and differentiation. However, it is time-consuming to proliferate and differentiate these tissues, and human fetal pancreatic tissue is hard to obtain due to its short supply [5]. By contrast, acinar cells are very easy to obtain in the course of pancreas islet-separation for islet cell transplantation. If a technique for the proliferation and beta-cell differentiation of acinar cells could be established, the feasibility of islet transplantation would increase.
Recently, very diverse efforts to differentiate stem cells to beta-cells by transfection of pancreas-specific transcription factors have been reported. Pancreas duodenum homeobox factor 1 (PDX-1) is the most important transcription factor in the development of the pancreas, as it controls the expression of several transcription factors and induces stem cell differentiation into beta-cells. It is also essential in maintaining the function of differentiated beta-cells [6]. Non beta-cells, such as acinar cells and hepatocytes, can be differentiated into beta-cells by inducing the expression of PDX-1 [7]. The expression of PDX-1 is detected in high levels in ductal cells and islet cells during pancreatic regeneration, which indicates that it is an important factor in endocrine regeneration and in the induction of the differentiation of ductal cells into endocrine cells [8]. As transfection of PDX-1 is limited by the low efficacy of controlling gene transcription by intranuclear PDX-1 alone, the VP16 transcriptional activation domain was inserted into PDX-1, and PDX-1/VP16 has been reported to act more efficiently in the role of PDX-1 [9]. The beta-cell E-box transcription factor (BETA2/NeuroD) is a transcription factor also found in pancreatic cells, and is known to play a key role in the development of the pancreas, differentiation of the insulin-producing beta-cells, and maintenance of normal beta-cell function, such as increased insulin production [9]. Expression of V-maf Musculoaponeurotic Fibrosarcoma Oncogene Homolog A (MafA) is very specific to the pancreas and is the most important factor in the control of the insulin gene [7]. Many researchers have tried to differentiate hepatocytes or acinar cells into insulin-producing or insulin gene-expressing beta-cells using a combination of pancreas-specific transcription factors, and the three transcription factors described above are reported to be the most effective in inducing differentiation into insulin-producing cells [10].
Therefore, we investigated the effects of overexpression of PDX-1/VP-16, BETA2/NeuroD, and MafA on the proliferation and differentiation of porcine neonatal pancreas cell clusters (NPCCs) and pancreatic ductal and acinar cells from adult pigs into beta-cells. Furthermore, we compared the beta-cell differentiation characteristics between both cell types.
Hanks' balanced salt solution (HBSS), Dulbecco's Modified Eagle's Medium (DMEM; GIBCO, Grand Island, NY, USA), dissociation medium (Sigma Chemical, St. Louis, MO, USA), and Collagenase P (Boehringer-Mannheim, Indianapolis, IN, USA) were used to isolate and culture porcine NPCC and the pancreatic ductal and acinar cells from adult pigs. We added nicotinamide (1,220 mg/L), HEPES (2,380 mg/L), bicarbonate (2,000 mg/L), L-glutamine (600 mg/L), fetal bovine serum (FBS; 100 mL/L), penicillin (100 U/mL; Sigma Chemical), and streptomycin (100 µg/mL; Sigma Chemical) to DMEM (8 mM glucose) for cell culture. Minimum Essential Medium (MEM; GIBCO) with added bicarbonate (2,200 mg/L), FBS (100 mL/L), penicillin (100 U/mL), and streptomycin (100 µg/mL) was used for adenovirus replication, and cesium chloride (CsCl) was used for virus purification. For immunostaining, polyclonal rabbit anti-pancytokeratin, polyclonal guinea pig anti-insulin (Zymed, San Francisco, CA, USA), and rabbit anti-human α-amylase (Sigma, St. Louis, MO, USA) were used as primary antibodies, and biotin-conjugated anti-guinea pig IgG, avidin-biotin complex (Vectastain® ABC kit; VECTOR Laboratory, Burlingame, CA, USA) was used as the secondary antibody.
Porcine NPCCs were prepared from neonatal pigs aged 1-2 days [11]. The entire pancreas was removed from the piglet, cut into small pieces (2 mm3) in M199 solution and washed in HBSS three times. The tissue was finely minced and shaken twice at 160 rpm in a Collagenase P solution (2.5 mg/mL) at 37℃ for 7 minutes. The digested tissue was washed three times in HBSS at 4℃ for 2 minutes at 1,000 rpm centrifugation and cultured in Hams' F10 solution at 37℃ in a humidified atmosphere with 95% O2/5% CO2 for 12 hours. Cultured NPCCs were isolated as single cells by repetitive suction and excretion using a 5 mL-pipette (Greiner Bio-One, Monroe, NC, USA). Dispersed cells isolated from NPCCs were counted under a light microscope and cultured in DMEM with 10% FBS on a 6-well culture plate or in a 100 mm dish (1×106 and 4×106 cells per plate, respectively). The culture medium was changed on the third day after isolation, and every other day thereafter.
Pancreatic ductal cells and acinar cells obtained during an islet cell-isolation procedure performed on adult pig pancreata were kindly supplied from the Xenotransplantation Research Center at Seoul National University Hospital. Beta-cells were removed after dithizone (DTZ) staining, and the remnant pancreatic cells were scattered into single cells in 10 mL of dissociation medium using a 5 mL-pipette. Cells were cultured in DMEM containing 10% FBS (2×107 cells in a 150 mm culture dish [NUNC™, Roskilde, Denmark]) for 1 week. The culture medium was changed every other day. In order to analyze the characteristics and mRNA expression patterns of the cells, they were also plated on 6-well culture plates (NUNC™) at a concentration of 1.5×106 cells per well.
Recombinant viruses expressing green fluorescent protein (GFP), PDX-1/VP16, BETA2/NeuroD and MafA were donated from Dr. Kaneto (Osaka University Graduate School of Medicine, Japan) [7,9,10]. All recombinant viruses were produced by the Adeno-Easy system using the pAdTrack-CMV shuttle vector [9]. The PDX-1/VP16 and BETA2/NeuroD coding sequences also contain a GFP expressing sequence. To replicate recombinant virus, adenovirus was added to serum-free MEM culture media at 80 to 90% confluence of HEK 293 cells (Korean Cell Line Bank, Seoul, Korea) in a 100 mm culture dish. Two hours later, the culture media was replaced with MEM media containing 5% FBS. When 30 to 40% of cells were floating at 48 hours after virus-treatment, the cells were collected and centrifuged at 1,000 rpm for 5 minutes. A cell lysate was prepared by treatment with 5 mL of Tris-Cl (10 mM/pH 7.9) with subsequent centrifugation at 2,500 rpm for 30 minutes. The supernatant containing virus was removed and mixed with 3.47 mL (specific gravity 1.4) and 1.2 mL (specific gravity 1.2) of CsCl, and virus was isolated by ultra-centrifugation at 35,000 rpm for 90 minutes at 4℃. The isolated virus was dialyzed with PBS using the Slide-A-Lyzer® Dialysis Cassette (Thermo Fisher, Rockford, IL, USA) and subsequently was dialyzed with virus stock solution (10 mM Tris, 2 mM MgCl2, 5% Sucrose/pH 8.0) for 2 hours. The virus stock solution was stored at -70℃. We measured the concentration of virus with an Adeno-X™ Rapid Titer Kit (Clontech, Mountain View, CA, USA).
Cells from NPCCs and adult pig pancreas were transduced with adenovirus. The cells were transduced with PDX-1/VP16, BETA2/NeuroD, MafA, and Ad-GFP at 100 MOI for 12 hours in the minimum volume of media (15 mL) with a media change after 12 hours.
Flow cytometric analysis was conducted to identify the expression of adenovirus in the cultured pancreatic cells. At 48 hours after transduction, cells were collected and washed with PBS containing 1% FBS (pH 7.4). Then, 1 mL of PBS was added to the cells and the expression of GFP was analyzed using FACScan™ (Becton & Dickinson, San Jose, CA, USA).
Nude mice (BALB/cSIc-nu; Japan SLC Inc., Shizuoka, Japan) were anesthetized with an intraperitoneal injection of 0.1 mL of a 1:5 mixture of Rumpun (23 mg/mL; Bayer Korea, Ansan, Korea) and Ketamine (50 mg/mL; Yuhan Co., Seoul, Korea), which was diluted 1:1 with normal saline. The cells for transplantation were initially classified as porcine NPCCs and adult pig pancreatic cells according to their origin, and then each group was subdivided into two groups based on the virus vector received: 1) group Ad-GFP indicates pancreatic cells infected with Adenovirus-GFP, and 2) group Ad-MIX indicates pancreatic cells that over-express PDX-1/VP16, BETA2/NeuroD and MafA. One kidney was transplanted with group Ad-GFP and the other kidney was transplanted with group Ad-MIX. The renal capsule was dissected with a 26 G needle after exposing the kidney, and 4,000 IEq (islet equivalents=number of islets if all had a diameter of 150 µm) [12] of each group of cells were injected at the subcapsular area with a PE-50 tube (Becton & Dickinson). The graft was harvested at 4 weeks after transplantation (Fig. 1).
The graft was fixed in 10% neutral buffered formalin (Sigma) at room temperature for 16 hours and embedded in paraffin, was and subsequently cut into 4 µm sections using a microtome (Microtome DSC1; Leica, Tokyo, Japan). Tissues were reacted with guinea pig anti-insulin antibody (1:100) as the primary antibody at 4℃ for 12 hours and then with biotin-conjugated anti-guinea pig IgG as the secondary antibody for 30 minutes. We performed an avidin-biotin complex reaction. Diaminobenzidine tetrahydrochloride (Sigma) was added for the color reaction and counter staining with hematoxylin was performed a few minutes later. Characterization of the pancreatic cells culturing the cells in a 6-well plate for 4 days followed by stainings with rabbit anti-pancytokeratin antibody (1:100), guinea pig anti-insulin antibody (1:100), rabbit anti-human α-amylase antibody (1:1,000) and DAPI. Anti-rabbit FITC and anti-guinea pig Rhodamin (1:100) were used as the secondary antibodies. The stained cells were observed with a confocal microscope (BIORAD MRC 1024; Bio-Rad, Hercules, CA, USA).
The relative ratio of insulin-expressing cells in the tissue was determined for the entire graft by point count methods on a video screen projected from a light microscope (×200) [13]. In the case of cultured cells isolated from NPCCs or adult pig pancreas, insulin-expressing cells were counted under a fluorescence microscope and expressed as the proportion (%) of insulin-positive cells to DAPI-positive cells.
Total RNA was isolated with Trizol (Invitrogen, Carlsbad, CA, USA) and quantified by UV/VIS Spectrophotometry (ND-1000; Nanodrop, Wilmington, DE, USA). One microgram of RNA was mixed with Oligo (dT) 12-18 Primer (Invitrogen) and dNTP Mix (10 mM each; Invitrogen), and reacted with a mixture of 5X strand buffer, 100 mM dithiothreitol (DTT), RNase OUT™ (40 units/µL; Invitrogen), and SuperScript™ II Reverse Transcriptase (200 units; Invitrogen) at 25℃ for 10 minutes, 42℃ for 1 hour, and 90℃ for 5 minutes, sequentially. cDNA obtained from reverse transcription and the gene-specific primers (Table 1) were added to Perfect PreMix (Takara Biomedicals, Kyoto, Japan) and amplified by PCR. PCR products were quantified by measurement of the luminescence with a bright densitometer (VDS; Amersham Pharmacia Biotech, Uppsala, Sweden) after electrophoresis on a 2% agarose gel.
cDNA products, obtained as described above, was diluted in 100 ng/µL of ultra-pure water. Aliquots of 100 ng of cDNA were used as a template in 20 µL reaction mixtures including 1×SYBR Mastermix, 10 pM primers pair (Table 2), 0.4 µL of ROX reference dye. PCR products were confirmed by melting curve and electrophoretic analyses. The signal fluorescence magnitude was detected using MiniOpticon™ real-time system (Bio-Rad). The data were analyzed using Supports Opticon Monitor™ software (Bio-Rad).
Cultured cells were washed with Krebs-Ringer Bicarbonate (KRB) buffer and incubated in euglycemic KRB buffer (5.5 mM glucose) for 1 hour. The buffer was collected, and its insulin concentration was measured. The same measurement was repeated under the same conditions except that the glucose concentration in the KRB buffer was 25 mM.
Monolayer cells from NPCCs were stained with anti-pancytokeratin antibody, anti-α-amylase antibody, and anti-insulin antibody on the fifth day after the monolayer cell culture was started. Among the cells in the culture plate, 71.2±15.1% of cells were positive for anti-pancytokeratin antibody staining, 5.3±9.4% were positive for anti-α-amylase antibody staining, and 13.6±4.9% were positive for anti-insulin antibody staining.
Monolayer cells isolated from adult pig pancreas were stained with anti-pancytokeratin antibody, anti-α-amylase antibody, and anti-insulin antibody on the fifth day after monolayer cell culture. Among the cells in the culture plate, 41.7±8.4% of cells were positive for anti-pancytokerain antibody staining, 49.4±3.5% were positive for anti-α-amylase antibody staining, and 2.5±3.1% were positive for anti-insulin antibody staining.
We verified the expression of GFP in NPCCs and adult pig pancreatic cells at 48 hours after virus treatment. We confirmed the over-expression of GFP in the adenovirus treatment group, and flow cytometric analysis showed 52% of cells from NPCCs and 67% of cells from adult pig pancreas were positive for GFP expression (Fig. 2).
We confirmed the overexpression of PDX-1/VP16, BETA2/NeuroD, and MafA after 3 days of monolayer cell culture following virus infection (Fig. 3A and B). Both NPCCs and adult pig pancreatic cells exhibited significantly increased expression of insulin induced by the overexpression of PDX-1/VP16, BETA2/NeuroD, and MafA. The level of insulin expression was less in adult pig pancreatic cells than in NPCCs (Fig. 3C).
On the 7th day after transduction, we measured the degree of NPCC differentiation into beta-cells. Group Ad-MIX showed significantly higher expression of insulin compared to group Ad-GFP; 26.2±5.0% of cells in group Ad-MIX and 10.1±1.7% in group Ad-GFP were positively stained with insulin antibody (Fig. 4A).
Adult pig pancreatic cells were immunostained 7 days after transduction, and 2.2±0.6% of cells in group Ad-GFP and 2.4±0.7% in group Ad-MIX were positively stained with insulin antibody. The difference in the expression of insulin between the groups was not statistically significant (Fig. 4B).
We measured the glucose-stimulated insulin secretion of NPCCs and adult pig pancreatic cells on the 7th day post-transduction. The cells were serially exposed to 5.5 mM glucose solution for 1 hour and 25 mM glucose solution for 1 hour. In the case of NPCCs, insulin secretion in group Ad-GFP was 13.3±0.8 µU/mL under the 5.5 mM glucose condition and 19.0±1.5 µU/mL under the 25 mM glucose condition. The insulin secretion in group Ad-MIX was 11.0±3.6 µU/mL under the 5.5 mM glucose condition and 27.5±9.7 µU/mL under the 25 mM glucose condition. Glucose-stimulated insulin secretion increased significantly in group Ad-MIX. However, there was no change of glucose-stimulated insulin secretion in the case of the adult pig pancreatic cells (Fig. 5).
NPCCs were transplanted into the renal subcapsular area of nude mice. Four weeks after transplantation, the graft was immunostained with insulin antibody and the number of positive cells was calculated by the point counting method in order to determine whether the cells had differentiated into beta-cells. The proportion of insulin antibody-stained cells was 13.6±4.9% in the NPCCs before transplantation, 12.78±3.95% in the graft transplanted with group Ad-GFP, and 24.73±10.4% in the graft transplanted with group Ad-MIX (Fig. 6A). Compared to NPCCs before transplantation, the proportion of beta-cells in the graft transplanted with group Ad-MIX was significantly increased, whereas it was decreased in the graft transplanted with group Ad-GFP.
Adult pig pancreatic cells were transplanted into the renal subcapsular area of nude mice. Four weeks after transplantation, the graft was immunostained with insulin antibody and the number of positive cells was calculated by the point counting method in order to determine whether the cells had differentiated into beta-cells. The proportion of insulin-positive cells was 2.5±3.1% in the cultured cells before transplantation, 2.3±2.0% in the graft transplanted with group Ad-GFP and 2.7±2.3% in the graft transplanted with group Ad-MIX (Fig. 6B). The difference in the proportion of beta-cells in the grafts transplanted with group Ad-GFP and Ad-MIX compared to the cultured cells before transplantation was not statistically significant.
This study demonstrated the influence of PDX-1/VP-16, BETA2/NeuroD, and MafA overexpression on the differentiation of NPCCs and pancreatic ductal and acinar cells into beta-cells. We previously reported that most of the cells isolated from neonatal pancreas are ductal cells and that they have a potent capacity for proliferation and differentiation, making them an attractive potential source of islet tissue for transplantation [14]. However, it is time-consuming to proliferate and differentiate these cells with the added potential risk of zoonosis and immunogenic rejection after xenotransplantation, which limit the use of neonatal pancreatic ductal cells clinical application. In this study, transfection enhanced the differentiation capacity of NPCCs. The most commonly used genes in transfection are pancreas-specific transcription factors such as BETA2/NeuroD, Neurogenin 3 (Ngn3), PDX-1, and MafA, and among them, PDX-1 is the keystone [7,9]. PDX-1, also known as insulin promoter factor 1 (Ipf1), is a protein belonging to the homeodomain family that plays an important role in pancreatogenesis and endocrine cell differentiation; if its expression is blocked the pancreas cannot develop [15]. PDX-1 is expressed in insulin-secreting beta-cells and somatostatin-secreting δ cells after genesis of the pancreas [16-18], which implies that PDX-1 is essential to insulin production in beta-cells and to maintaining the glucose sensing system [17]. Furthermore, PDX-1 was reported to control a few genes associated with maintaining normal beta-cell function [18]. We also reported that Ad-PDX-1/VP16 induced the differentiation of adult dog pancreatic cells into beta-cells [19]. However, the lower capacity for differentiation compared to fetal tissue has been the limitation of adult dog pancreatic cells application, suggesting an additional method is needed to potentiate differentiation and proliferation. In this study, we used a multiple gene transfection method that included BETA2/NeuroD, MafA, and PDX-1. BETA2/NeuroD is a bHLH protein gene and its expression is induced by Ngn3 [20]. It is found in the pancreatic epithelium during pancreatogenesis and is expressed after birth in α, β, and δ cells, but not in acinar cells. BETA2/NeuroD transactivates insulin, glucagons and secretin genes [21]. MafA is a recently reported transcription factor that is found specifically in pancreatic beta-cells and plays a key role in controlling expression of the insulin gene. Although overexpression of PDX-1, BETA2/NeuroD or MafA alone, or dual overexpression of PDX-1 and BETA2/NeuroD, does not increase the expression of the insulin gene, simultaneous overexpression of PDX-1, BETA2/NeuroD and MafA significantly increases the expression of the insulin gene [7,22].
We studied the effect of PDX-1, BETA2/NeuroD, and MafA on beta-cell differentiation using an adenovirus transfection technique. Initially, cultured NPCCs were transfected, immunostained, and beta-cell specific gene expression was investigated by RT-PCR. Immunostaining results showed that transfection of group Ad-MIX increased the expression of insulin compared to group Ad-GFP. We previously reported that 22% of the total NPCCs were found to be endocrine cells. The proportion decreased as the cells were passaged until almost all cells were pancreatic epithelial cells and fibroblasts after 1 week of culture [5,14,23,24]. The fact that insulin expression in the group transfected with PDX-1, BETA2/NeuroD, and MafA was statistically different compared to the control group implies that PDX-1, BETA2/NeuroD, and MafA might have enhanced the transcription of the insulin gene and differentiation into beta-cells.
We also studied the adult pancreatic cells obtained from adult pig pancreas depleted of islet cells by DTZ staining. Immunostaining showed that almost all of the cultured pancreatic cells were acinar cells, which was a relatively higher proportion of acinar cells compared to a previous report in which 75.2±15.1% of cells were positive for pancytokeratin and 19.6±4.9% positive for hormone antibody [23]. This difference might be due to the fact that we obtained pancreatic cells from the remnant pancreas remaining after islet-isolation, and therefore it had a small number of beta-cells. Pancreatic acinar cells isolated from adult pancreas might have a lower capacity to transdifferentiate into ductal cells. Additionally, adult pancreatic ductal cells have a limited capacity for proliferation compared to NPCCs, which show prominent proliferation of ductal cells and transdifferentiation of acinar cells to ductal cells accompanied with a rapid loss of acinar cells after isolation. This might explain the smaller proportion of ductal cells and larger proportion of acinar cells in the adult pig pancreatic cells compared to NPCCs.
NPCCs can transdifferentiate into beta-cells and proliferate; data shows that 40% and 90% of cells transdifferentiate after 8 and 54 weeks post-transplantation to the renal subcapsular area of nude mice, respectively [14]. Our study used transfection for rapid transdifferentiation into beta-cells and showed that the proportion of beta-cells was 12.78±3.95% in group Ad-GFP and 24.73±10.4% in group Ad-MIX at 4 weeks after transplantation. This result was lower than the 40% at 8 weeks post-transplantation that was reported in neonatal pig pancreatic tissue. Toxicity of the adenovirus might be the cause of this; however, transfection enhanced the efficacy of beta-cell transdifferentiaion.
In the case of transplantation with adult pig pancreatic cells, there was no difference in the proportion of beta-cells between the Ad-GFP and Ad-MIX groups, which might be a result of the toxicity of the adenovirus and thus a lesser degree of overexpression of PDX-1 or BETA2/NeuroD. Furthermore, hypoxia during transplantation could have caused the loss of cells. The difference in the result of transplantation between NPCCs and adult pig pancreatic cells reflects the markedly different characteristics of the pancreatic cells obtained from a fetus and an adult. Adult pancreatic ductal cells have only a limited capacity for proliferation and differentiation, whereas they are relatively easy to obtain and can be applied in allogenic- or autologous transplantation. Prior to application in transplantation, adult pancreatic ductal cells must be induced to a state of active proliferation and differentiation.
Our study was performed in nude mice, and therefore the implications of immunosuppressants were not considered. In clinical application, however, immunosuppressants are needed and their toxicity must be considered. Additionally, less toxic methods for immune suppression such as encapsulation may also be beneficial.
In conclusion, overexpression of PDX-1/VP16, BETA2/NeuroD, and MafA significantly increased the beta-cell differentiation of transplanted NPCCs but not transplanted adult pig pancreatic cells, which showed a markedly lower capacity for transdifferentiation. Development of an additional method to increase the proliferation and transdifferentiation of adult pancreatic cells is needed in order to apply them to islet transplantation.
Figures and Tables
Fig. 1
Schematic of the experimental design. The general scheme of the pancreatic cell culture system was separated into two groups: porcine neonatal pancreatic cell clusters (NPCCs) and adult pig pancreatic cells. In monolayer cultures, we employed adenoviruses for PDX-1, BETA2/NeuroD, and MafA expression. Ad-GFP was used as an adenoviral control. On day 0, Ad-GFP or adenovirus-infected NPCCs and adult pig pancreatic cells were transplanted under the kidney capsule of normoglycemic nude mice. Finally, all grafts were harvested at 4 weeks.
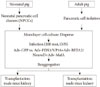
Fig. 2
Adenovirus-mediated expression of green fluorescent protein (GFP) and PDX-1+BETA2+MafA in the neonatal pancreatic cell clusters (NPCCs) and adult pig pancreatic cells. The NPCCs (A) and adult pig pancreatic cells (B) were visible 48 hours after infection with Ad-GFP (left) and Ad-PDX-1/VP16+BETA2/NeuroD+MafA (right).
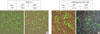
Fig. 3
The effect of adenovirus infection on beta-cell-specific gene expression in neonatal pancreatic cell clusters (NPCCs) (A)and adult pig pancreatic cells (B). (C) The bar graphs illustrates the quantification of the RT-PCR results. Results are expressed as means±standard deviation for n=10 independent experiments. GFP, green fluorescent protein. aP≤0.05, bP≤0.001.
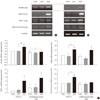
Fig. 4
Immunocytochemical staining of insulin and cytokeratin expression levels in neonatal pancreatic cell clusters (NPCCs) (A) and adult pig pancreatic cells (B). Immunocytochemical staining of insulin and cytokeratin in NPCCs and adult pig pancreatic cells was performed at 7th day after transduction. GFP, green fluorescent protein.
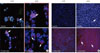
Fig. 5
Glucose-stimulated insulin secretion (GSIS) was observed between neonatal pancreatic cell clusters (NPCCs) and adult pig pancreatic cells. GFP, green fluorescent protein. aP≤0.05.
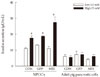
ACKNOWLEDGMENT
This work was supported by the Korea Research Foundation Grant funded by the Korean Government (KRF-2008-314-C00254) and by the Korea Health 21 R & D Project, Ministry of Health & Welfare, Republic of Korea (A040004). We thank Dr. Kaneto (Osaka University Graduate School of Medicine, Japan) for donating the recombinant virus.
References
1. Kaczorowski DJ, Patterson ES, Jastromb WE, Shamblott MJ. Glucose-responsive insulin-producing cells from stem cells. Diabetes Metab Res Rev. 2002. 18:442–450.
2. Robertson RP. Islet transplantation as a treatment for diabetes: a work in progress. N Engl J Med. 2004. 350:694–705.
3. Yoon KH, Ko SH, Cho JH, Lee JM, Ahn YB, Song KH, Yoo SJ, Kang MI, Cha BY, Lee KW, Son HY, Kang SK, Kim HS, Lee IK, Bonner-Weir S. Selective beta-cell loss and alpha-cell expansion in patients with type 2 diabetes mellitus in Korea. J Clin Endocrinol Metab. 2003. 88:2300–2308.
4. Swenne I. Pancreatic beta-cell growth and diabetes mellitus. Diabetologia. 1992. 35:193–201.
5. Yoon KH. Recent update for pancreas stem cells. Korean J Anat. 2004. 37:123–131.
6. Habener JF, Kemp DM, Thomas MK. Minireview: transcriptional regulation in pancreatic development. Endocrinology. 2005. 146:1025–1034.
7. Kaneto H, Miyatsuka T, Nakatani Y, Matsuoka TA. PDX-1 and MafA: key transcription factors in pancreas. Am J Biochem Biotechnol. 2005. 1:54–63.
8. Sharma A, Zangen DH, Reitz P, Taneja M, Lissauer ME, Miller CP, Weir GC, Habener JF, Bonner-Weir S. The homeodomain protein IDX-1 increases after an early burst of proliferation during pancreatic regeneration. Diabetes. 1999. 48:507–513.
9. Kaneto H, Nakatani Y, Miyatsuka T, Matsuoka TA, Matsuhisa M, Hori M, Yamasaki Y. PDX-1/VP16 fusion protein, together with NeuroD or Ngn3, markedly induces insulin gene transcription and ameliorates glucose tolerance. Diabetes. 2005. 54:1009–1022.
10. Kaneto H, Matsuoka TA, Nakatani Y, Miyatsuka T, Matsuhisa M, Hori M, Yamasaki Y. A crucial role of MafA as a novel therapeutic target for diabetes. J Biol Chem. 2005. 280:15047–15052.
11. Korbutt GS, Elliott JF, Ao Z, Smith DK, Warnock GL, Rajotte RV. Large scale isolation, growth, and function of porcine neonatal islet cells. J Clin Invest. 1996. 97:2119–2129.
12. Sutherland DE, Gores PF, Hering BJ, Wahoff D, McKeehen DA, Gruessner RW. Islet transplantation: an update. Diabetes Metab Rev. 1996. 12:137–150.
13. Weibel ER. Stereologic methods: practical methods for biologic morphometry. 1978. London: Academic Press;101–161.
14. Yoon KH, Quickel RR, Tatarkiewicz K, Ulrich TR, Hollister-Lock J, Trivedi N, Bonner-Weir S, Weir GC. Differentiation and expansion of beta cell mass in porcine neonatal pancreatic cell clusters transplanted into nude mice. Cell Transplant. 1999. 8:673–689.
15. Ramiya VK, Maraist M, Arfors KE, Schatz DA, Peck AB, Cornelius JG. Reversal of insulin-dependent diabetes using islets generated in vitro from pancreatic stem cells. Nat Med. 2000. 6:278–282.
16. Miller CP, McGehee RE Jr, Habener JF. IDX-1: a new homeodomain transcription factor expressed in rat pancreatic islets and duodenum that transactivates the somatostatin gene. EMBO J. 1994. 13:1145–1156.
17. Ahlgren U, Pfaff SL, Jessell TM, Edlund T, Edlund H. Independent requirement for ISL1 in formation of pancreatic mesenchyme and islet cells. Nature. 1997. 385:257–260.
18. Ohlsson H, Karlsson K, Edlund T. IPF1, a homeodomain-containing transactivator of the insulin gene. EMBO J. 1993. 12:4251–4259.
19. You YH, Park SC, Lee SH, Park HS, Ham DS, Rhee M, Kim JW, Song KH, Yoon KH. PDX-1/VP16 overexpression induce the transdifferentiation of canine adult pancreatic cells into beta-cells. J Korean Diabetes Assoc. 2007. 31:51–62.
20. Huang HP, Liu M, El-Hodiri HM, Chu K, Jamrich M, Tsai MJ. Regulation of the pancreatic islet-specific gene BETA2 (neuroD) by neurogenin 3. Mol Cell Biol. 2000. 20:3292–3307.
21. Lee JC, Smith SB, Watada H, Lin J, Scheel D, Wang J, Mirmira RG, German MS. Regulation of the pancreatic pro-endocrine gene neurogenin3. Diabetes. 2001. 50:928–936.
22. Kaneto H, Matsuoka TA, Katakami N, Matsuhisa M. Combination of MafA, PDX-1 and NeuroD is a useful tool to efficiently induce insulin-producing surrogate beta-cells. Curr Med Chem. 2009. 16:3144–3151.
23. Suh SH, Yoon KH, Kwon HS, Hong OK, Lee JM, Song KH, Yoo SJ, Son HS, Kang MI, Cha BY, Lee KW, Son HY, Kang SK. 3-dimensional long term culture of monolayer cultured dispersed neonatal porcine pancreas cells (NPCC). J Korean Diabetes Assoc. 2002. 26:383–395.
24. Weir GC, Quickel RR, Yoon KH, Tatarkiewicz K, Ulrich TR, Hollister-Lock J, Bonner-Weir S. Porcine neonatal pancreatic cell clusters (NPCCs): a potential source of tissue for islet transplantation. Ann Transplant. 1997. 2:63–68.