Abstract
This study was performed to evaluate the use of vibrating microneedles for the transdermal delivery of vitamin C. The microneedles were designed to vibrate at three levels of intensity. In vitro permeation by vitamin C was evaluated according to the specific conditions such as vibration intensity (levels 1, 2 and 3), application time (1, 3, 5, 7 and 10 min), and application power (500, 700 and 1,000 g). The highest permeation of vitamin C was observed at level 3 of vibration intensity, 5 min of application, and 1,000 g of application power. Vitamin C gel showed no cytotoxic effect against Pam212 cells or skin irritation effects. A pharmacokinetic study of the gel in rats was conducted under optimized conditions. The AUC0-∞ and Cmax increased 1.35-fold and 1.44-fold, respectively, compared with those after vitamin C gel without application with vibrating microneedles. The present study suggests that vibrating microneedles can be used to facilitate the skin permeability of vitamin C under optimal conditions.
Despite the advantages of transdermal drug delivery systems (TDDS), such as extreme convenience, better patient compliance, sustained delivery, avoidance of hepatic first-pass effect and degradation in the gastrointestinal tract,[12] the skin is a natural barrier. Only a few drugs can penetrate the skin easily with the aid of penetration enhancers, such as soybean phospholipids,[3] long-chain fatty alcohols, cyclic monoterpenes[45] or nonionic surfactants.[6]
In recent years, microneedle technology as proposed by Henry et al.[7] has been developed as an advanced technique for the penetration of large molecular weight and hydrophilic compounds into the skin. Micrometer-sized needles were shown to be long to penetrate the skin effectively breaching the stratum corneum barrier, but being sufficiently short enough to minimize pain. Generally, a microneedle-facilitated process results in up to several orders-of-magnitude enhancement of drug transdermal transport.[27] Microneedles have been designed to be coated with the drug or to encapsulate the drug for release into the skin by dissolution.[891011] Hollow microneedles have been coupled with a syringe for active drug infusion.[121314]
L-Ascorbic acid (vitamin C), a representative water-soluble vitamin, has a variety of biological, pharmaceutical and dermatological functions; it promotes collagen biosynthesis, provides photoprotection, causes melanin reduction, scavenges free radicals, and enhances the immunity (antiviral effect).[1516171819] These properties are closely related to the well-known antioxidant properties of this compound. However, the transdermal permeability of vitamin C is low. Therefore, various noninvasive technologies beyond chemical penetration enhancers have been developed to bypass or modulate the skin barrier by physical means, such as microporation (microneedles), electroporation, iontophoresis, or sonophoresis.[2021] Vitamin C is unstable when exposed to air, humidity, light, heat, metal ions, oxygen, and bases, whereupon it subsequently decomposes easily into biologically inactive compounds such as 2,3-diketo-L-gulonic acid, oxalic acid, L-threonic acid, L-xylonic acid or L-lyxonic acid. Therefore, the application of vitamin C in various fields of cosmetics, dermatologicals, or pharmaceuticals are limited despite its benefits.[1722232425]
Additionally, to exploit the TDDS more efficiently scientists have worked on some combinational approaches for manufacturing TDDS by iontophoresis, electroporation, ultrasound, and pressure waves, which subsequently reported the synergistic effect of TDDS.[26] In this study, vibrating microneedles designed to operate on three levels were used to increase in vitro and in vivo transdermal delivery of vitamin C.
Vitamin C was purchased from Samchun Co. (Pyeongtaek, Korea). Carbomer 940 NF was purchased from Lubrizol Co. (Wickliffe, USA). Glacial acetic acid and triethanolamine were purchased by Duksan Co. (Ansan, Korea). Methanol and acetonitrile were HPLC grade and purchased from JT Baker (USA). All other chemicals were reagent grade and used without further purification. Vibrating microneedles were provided by Korea Institute of Industrial Technology (Ansan, Korea). A microneedle array was attached magnetically to a vibrating handle.
The semisolid dosage forms for topical and transdermal drug deliveries include creams, ointments, gels, and lotions. Unlike transdermal patches that require highly specialized expertise for patch design and sophisticated manufacturing systems, semisolid dosage forms can easily be screened in a lab setting.[27] So, application formulations of a gel of semisolid and a gel were selected and produced. First, 1.5 g of vitamin C was dissolved completely in 10 mL of distilled water (D.W.). Previously, 1 g of carbomer 940 was hydrated in 80 mL of D.W. and then vitamin C solution was added to the carbomer 940 solution. The mixtures were stirred using a magnetic stirrer, and then vitamin C gel was freshly formed with triethanolamine.
Abdominal hair on rats was previously removed and then the abdominal skin was excised for a permeation study. Fat and other tissues attached to the abdominal skin were removed carefully and the excised rat skin was stored in a freezer at –20℃ before experiments. In vitro skin permeation tests were conducted with Franz-type diffusion cells with an effective diffusion area of 2.0 cm2 having the diameter of 16 mm and receptor volume of 12.5 mL. The receptor compartment contained pH 7.4 phosphate buffer and was maintained at 37℃ and stirred continuously with a magnetic stirrer at 300 rpm. Two hundred microliters of vitamin C solution (1.5 mg/mL) was loaded on the stratum corneum side, covered with parafilm and aluminum foil for shading. Permeability experiments of vitamin C solution were conducted until 24 h after applying a vibrating microneedles to the skin according to predetermined conditions such as different vibration intensities (levels 1, 2 and 3), application power (500 g, 700 g, 1,000 g) and application time (1 min, 3 min, 5 min, 7 min, 10 min). Samples were taken from the receptor compartment at predetermined intervals (0.5, 1, 2, 4, 8, 12 and 24 h) and replaced with the same volume of fresh phosphate buffer. The amount of vitamin C in the samples was measured by HPLC.
Three application variables such as vibration intensity, application power, and application time were considered to optimize application conditions for the vibrating microneedles. First, three different vibration intensities of levels 1, 2 or 3 were applied to study the in vitro skin permeation by vitamin C. Second, three different application powers of 500, 700 or 1,000 g were applied to study the in vitro skin permeation by vitamin C. Third, five different application times of 1, 3, 5, 7 or 10 min were applied to study in vitro skin permeation by vitamin C. All experiments were conducted by varying one parameter, while keeping all other process parameters constant.
The murine epidermal cell line, Pam212 was kindly subcultured by Dr. Kang, BK (College of Pharmacy, Chonnam National University, Gwangju, Korea). DMEM (GibcoBRL, Grand Island, NY, USA) was used for cell culture under an atmosphere of 5% CO2 at 37℃. The cytotoxicity of vitamin C solution, blank gel or vitamin C gel against Pam212 cells was measured using an MTT assay. After 24 h incubation of the cells (70% confluent) with control gel, vitamin C gel, or vitamin C solution, media of each well were replaced with MTT (3-(4,5-dimethyl-thiazol-2-yl)-2,5-diphenyl-2H-tetrazolium bromide) solution and incubated for 4 h at 37℃. DMSO was used to solubilize the crystal of viable cells and absorbance was determined at 570 nm in a microplate reader (Sunrise, Tecan, Austria). Cell viability was demonstrated as the percent absorbance affected to formulations relative to absorbance measured for cells that were not treated with vitamin C.
The experimental protocols were approved by the Animal Care Committee of Chungnam National University. Skin irritation was evaluated by observing the skin after the uniform spreading of the vitamin C gel over an area of 8.55 cm2. The skin surface was observed for any visible variation such as erythema (redness) after 24, 48 and 72 h of vitamin C gel. The mean erythema scores were recorded as 0 (no erythema), 1 (slight erythema), 2 (moderate erythema), 3 (moderate to severe erythema), and 4 (severe erythema) depending on the degree of erythema.
In vivo pharmacokinetic study in rats was conducted to confirm the optimized conditions established in vitro using Sprague Dawley (SD) rats. The SD rats (270–300 g) were purchased from Daehan Laboratory Animal Research Co. (Chungbuk, Korea), allowed free access to normal standard rodent diet (Jaeil Chow, Korea) and tap water. The animals were housed individually in laminar flow cages at 22 ± 2℃, and 50–60% relative humidity and stabilized in these facilities for at least 7 days before experiments in vivo. Procedures were approved by the Animal Care Committee of Chungnam National University. For percutaneous administration, the hair was removed from the skin of the dorsal region using electric hair clippers. The vitamin C gel alone was applied to the rat skin as a control. The vitamin C gel was loaded onto the shaved surface rat skin after applying the vibrating microneedles with the optimized conditions established by in vivo pharmacokinetic study in rats. Vibrating microneedles with vibration intensity of level 3, application power of 1,000 g and application of 5 min were pretreated for the transdermal administration of 0.5 g of the vitamin C gel (27.5 mg/kg of vitamin C). The application area was covered with a thin plastic film and fastened around the edges with adhesive tape to avoid loss of gel. Blood samples (about 1 mL) were collected at 0.5, 1, 2, 4, 8, 12, 24 and 72 h after percutaneous administration from the retro-orbital plexus using disposable capillary tubes that were prerinsed with heparin sodium in normal saline (20 IU). The collected blood samples were centrifuged at 11310 g for 10 min to obtain plasma, which was stored at −70℃ until analysis by HPLC.
Vitamin C in samples from the in vitro and in vivo studies was assayed using an Agilent 1100 liquid chromatography system fitted with an autosampler. The column used was a Zorbax SB C18 column (4.6 mm x 250 mm, 5∓mm particle size, Agilent, USA). The flow rate of the mobile phase was 0.5 mL/min and the detection wavelength was set at 245 nm. The mobile phase was D.W. and methanol (95:5 V/V). All procedures were conducted at room temperature.
In our study, the drug release rates were evaluated according to the simplified Higuchi diffusion equation (1), depicting the drug release from one side of a semisolid layer in which the drug is dissolved.
Where q is the amount of drug released into the receptor medium per unit area of exposure, C0 is the initial drug concentration in the vehicle, D is the apparent diffusion coefficient of the drug, and t is the time elapsed since the start of drug release. In case of passive diffusion, the steady-state flux through unit area of a membrane is given by Fick's law: where J is the flux per unit area, P represents the permeability coefficient, and Cd, Cr are the concentrations in the donor and receptor solutions, respectively. In case sink conditions are maintained on the receptor side, (Cd–Cr) is replaced by Cd.
The permeability coefficient, P, is the constant for a given drug under the same experimental condition. There should be a linear relationship between the flux and donor concentration.
The pharmacokinetic parameter was analyzed by noncompartment theory using the Winnolin program. Cmax and Tmax were provided from the experimental data. The AUC0–∞ from time zero to the time of the last observed plasma concentration (Clast) was calculated using the linear trapezoidal rule.
Microneedles were manufactured using a MEMS process (lithography and reactive ion etching with silicon wafer) and composite mold manufacturing process technology (high-precision metal technique using pulse reverse current electroforming) using a biocompatible polymer. The vibration intensity of microneedles was determined by pulse-width modulated (PWM) control. The PWM signal is a type of digital waveform. It alternates between bursts of “on” and “off ”, also known as high and low, respectively, at a fixed frequency. The specifications of microneedles are as follows: 159 of microneedles, 2.0 mm of microneedle spacing, 250 µm of microneedle height, and 35 mm of microneedle base (Fig. 1).
First, in vitro skin permeation study of vitamin C was conducted to determine the effect of three different levels of vibration intensities, namely 1, 2, or 3 for 3 min with an application power of 700 g (Fig. 2). With level 3 of vibration intensity, the highest permeation of vitamin C was observed 3601.33 µg/cm2 at 12 h after vitamin C gel (Table 1).
The influence of three different application powers with a fixed level 2 of vibration intensity and 3 min of application time on the permeation of vitamin C was also determined (Table 2). The highest permeability of 1795.83 µg/cm2 at 12 h after vitamin C gel was loaded was observed when an application power of 1,000 g was applied. This result was found by another study in which increasing the force used for microneedle application resulted in a significant increase in the depth of penetration achieved into neonatal porcine skin. For example, a microneedle of 600 µm height penetrated to a depth of 330 µm when inserted at a force of 4.4 N/array, while the penetration increased significantly to a depth of 520 µm when the force of application was increased to 16.4 N/array. At an application force of 11.0 N/array, it was found that, in each case, increasing microneedle height from 350 to 600 µm to 900 µm led to a significant increase in the depth of microneedle penetration achieved.[29]
When five different application times were applied, the highest permeability of 2051.39 µg/cm2 was shown in application time of 5 min with a fixed level 2 of vibration intensity and 700 g of application power (Table 3). Therefore, the optimal application conditions for the vibrating microneedles on permeability by vitamin C were vibration intensity of level 3, application power of 1,000 g, and application time of 5 min. Flux was calculated using equation 2 with mathematical modeling.
To determine the cytotoxic effect of gel formulation in this study, blank gel, vitamin C gel or vitamin C solution over the concentration of 0.01 µg/mL to 10 mg/mL against murine Pam212 cells was explored using an MTT assay (Fig. 3). After incubation for 24 h, no cytotoxicity of blank gel, vitamin C gel, or vitamin C solution against Pam212 was shown, suggesting the formulation applied to the skin did not have any cytotoxic effect, even with a high concentration of 10 mg/mL of vitamin C gel. Skin irritation was evaluated visually after applying vitamin C gel onto the rat skin. No irritation by the vitamin C gel was observed.
The mean plasma concentration–time release profile of vitamin C from vitamin C gel is shown in Figure 4. After applying vitamin C gel alone, the peak plasma concentration of vitamin C was increased to 66.9 µg/mL at 1 h, which gradually decreased to 6.9 µg/mL at 24 h. By contrast, when vitamin C gel was applied after pretreatment using vibrating microneedles, the peak plasma concentration of vitamin C was 96.2 µg/mL at 1 h, decreasing gradually to 12.9 µg/mL at 24 h. Also, when the vibrating microneedle pretreatment was used, AUC0–∞ was 1136.5 ± 340.0 µg·h/mL and AUC0–∞ was 843.4 ± 218.6 µg·h/mL in the absence of vibrating microneedle treatment. When the vibrating microneedles were applied, the AUC was increased 1.35 fold compared with the absence of vibrating microneedle pretreatment. Mean residence time (MRT) value with significance was 12.0 h in vitamin C gel after applying vibrating microneedles and longer than 9.8 h after applying vitamin C gel only, suggesting vitamin C could remain longer in plasma (Table 4).
A new concept, a microneedle system, was introduced to merge the advantages of transdermal delivery and parenteral delivery to increase the bioavailability of substances. However, in other scenarios, quick resealing of the pores is beneficial for safety reasons to avoid possible infection.[30] The kinetics of pore resealing after microneedle insertion have been studied in human subjects by electrical impedance measurements, which showed that pores formed by microneedles recovered their barrier properties within 2 h when the skin was not occluded, but took 3 to 40 h to recover when the skin was occluded, depending on the geometry of the microneedles.[3132] The kinetics of pore resealing were also studied in hairless rats using TEWL and tissue staining, and showed similar timeframes for pore resealing.[33] For the present vibrating microneedles, 5 min of application time was optimal for the transdermal delivery of vitamin C. Skin irritation scores for vitamin C gel were zero, which indicated the safety and acceptability of vibrating microneedles for transdermal administration of vitamin C gel.
In vivo pharmacokinetics in rats was determined based on the application conditions established in an in vitro skin permeation study. The stability of vitamin C in vitamin C gel should be considered. Vitamin C content in one aqueous solution of pure vitamin C decreased rapidly and down to <10% within 4 weeks. [34] The time-dependent stability of vitamin C in the plasma or various pH solutions was examined. Vitamin C was degraded 27% in plasma after 24 h incubation. However, vitamin C was stable in acidic solutions but degraded in alkaline solutions (data not shown).
This study addresses the use of vibrating microneedles to increase skin permeability by vitamin C. When applying vitamin C gel with vibrating microneedles, the optimized application conditions (level 3 of vibration intensity, 1,000 g of application power, and 5 min of application) showed the highest permeation by vitamin C. Considering these results, the vibrating microneedles appear to be useful in transdermal delivery of vitamin C.
Figures and Tables
Figure 2
In vitro cumulative amount of vitamin C permeated (µg/cm2) through excised rat skin up to 24 h according to the vibration intensity, application power and application time. (A), vibration intensity; (B), application power; (C), application time. Data was expressed as mean ± S.D (n = 3).
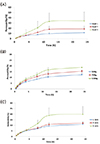
Figure 3
Cell viability according to the concentration of vitamin C solution, blank gel or vitamin C gel against Pam212 cells.
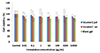
Figure 4
Mean plasma concentration–time profiles of vitamin C from vitamin C gel in the presence or absence of pretreatment by vibrating microneedles. Data was expressed as mean ± S.D (n = 3).
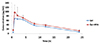
Table 1
Permeation parameters of vitamin C from the vitamin C gel according to the vibration intensity
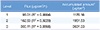
Level | Flux (µg/cm2/h) | Accumulated amounta (µg/cm2) |
---|---|---|
1 | 98.01 (R2 = 0.9864) | 1176.16 |
2 | 162.63 (R2 = 0.9229) | 1951.53 |
3 | 300.11 (R2 = 0.8868) | 3601.33 |
Table 2
Permeation parameters of vitamin C from the vitamin C gel according to the application power
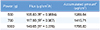
Power (g) | Flux (µg/cm2/h) | Accumulated amounta (µg/cm2) |
---|---|---|
500 | 105.80 (R2 = 0.9884) | 1269.64 |
700 | 117.98 (R2 = 0.987) | 1415.71 |
1000 | 149.65 (R2 = 0.976) | 1795.83 |
Table 3
Permeation parameters of vitamin C from the vitamin C gel according to the application time
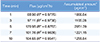
Table 4
Pharmacokinetic parameters of vitamin C after applying vitamin C gel and vitamin C gel with the vibrating microneedles (*p < 0.05)
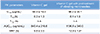
Acknowledgements
This study was supported by the Korea Institute of Industrial Technology (2013) and by the Priority Research Center Program (2009-0093815) through the National Research Foundation of Korea (NRF) funded by the Ministry of Education, Science and Technology, Republic of Korea.
References
1. Naik A, Kalia YN, Guy RH. Transdermal drug delivery: overcoming the skin's barrier function. Pharm Sci Technolo Today. 2000; 3:318–326.


3. Kirjavainen M, Mönkkönen J, Saukkosaari M, Valjakka-Koskela R, Kiesvaara J, Urtti A. Phospholipids affect stratum corneum lipid bilayer fluidity and drug partitioning into the bilayers. J Control Release. 1999; 58:207–214.


4. Parsaee S, Sarbolouki MN, Parnianpour M. In-vitro release of diclofenac diethylammonium from lipid-based formulations. Int J Pharm. 2002; 241:185–190.


5. Ho HO, Huang FC, Sokoloski TD, Sheu MT. The influence of cosolvents on the in-vitro percutaneous penetration of diclofenac sodium from a gel system. J Pharm Pharmacol. 1994; 46:636–642.


6. Shin SC, Kim HJ, Oh IJ, Cho CW, Yang KH. Development of tretinoin gels for enhanced transdermal delivery. Eur J Pharm Biopharm. 2005; 60:67–71.


7. Henry S, McAllister DV, Allen MG, Prausnitz MR. Microfabricated microneedles: a novel approach to transdermal drug delivery. J Pharm Sci. 1998; 87:922–925.


8. Gill HS, Prausnitz MR. Coated microneedles for transdermal delivery. J Control Release. 2007; 117:227–237.


9. Andrianov AK, DeCollibus DP, Gillis HA, Kha HH, Marin A, Prausnitz MR, et al. Poly [di (carboxylatophenoxy) phosphazene] is a potent adjuvant for intradermal immunization. Proc Natl Acad Sci USA. 2009; 106:18936–18941. DOI: 10.1073/pnas.0908842106.


10. Lee JW, Park JH, Prausnitz MR. Dissolving microneedles for transdermal drug delivery. Biomaterials. 2008; 29:2113–2124. DOI: 10.1016/j.biomaterials.2007.12.048.


11. Ito Y, Hagiwara E, Saeki A, Sugioka N, Takada K. Feasibility of microneedles for percutaneous absorption of insulin. Eur J Pharm Sci. 2006; 29:82–88.


12. Sivamani RK, Stoebe B, Wu GC, Zhai H, Liepmann D, Maibach H. Clinical microneedle injection of methyl nicotinate: stratum corneum penetration. Skin Res Technol. 2005; 11:152–156.


13. Gupta J, Felner EI, Prausnitz MR. Minimally invasive insulin delivery in subjects with type 1 diabetes using hollow microneedles. Diabetes Technol Ther. 2009; 11:329–337. DOI: 10.1089/dia.2008.0103.


14. Mikszta JA, Laurent PE. Cutaneous delivery of prophylactic and therapeutic vaccines: historical perspective and future outlook. Expert Rev Vaccines. 2008; 7:1329–1339. DOI: 10.1586/14760584.7.9.1329.


15. Machlin LJ. Handbook of Vitamins. 2nd Ed. Marcel Dekker, Inc;1991.
16. Bossi A, Piletsky SA, Piletska EV, Righetti PG, Turner AP. An assay for ascorbic acid based on polyaniline-coated microplates. Anal Chem. 2000; 72:4296–4300.


17. Yamamoto I, Tai A, Fujinami Y, Sasaki K, Okazaki S. Synthesis and characterization of a series of novel monoacylated ascorbic acid derivatives, 6-O-acyl-2-O-α-Dglucopyranosyl- l-ascorbic acids, as skin antioxidants. J Med Chem. 2002; 45:462–468.
18. Tsuchiya H, Bates CJ. Changes in collagen cross-link ratios in bone and urine of guinea pigs fed graded dietary vitamin C: A functional index of vitamin C status. J Nutr Biochem. 1998; 9:402–407.


19. Horino Y, Takahashi S, Miura T, Takahashi Y. Prolonged hypoxia accelerates the posttranscriptional process of collagen synthesis in cultured fibroblasts. Life Sci. 2002; 71:3031–3045.


20. Barry BW. Novel mechanisms and devices to enable successful transdermal drug delivery. Eur J Pharm Sci. 2001; 14:101–114.


21. Lombry C, Dujardin N, Préat V. Transdermal delivery of macromolecules using skin electroporation. Pharm Res. 2000; 17:32–37.
22. Gallarate M, Carlotti ME, Trotta M, Bovo S. On the stability of ascorbic acid in emulsified systems for topical and cosmetic use. Int J Pharm. 1999; 188:233–241.


23. Austria R, Semenzato A, Bettero A. Stability of vitamin C derivatives in solution and topical fornmlations. J Pharm Biomed Anal. 1997; 15:795–801.
24. Spiclin P, Gasperlin M, Kmetec V. Stability of ascorbyl palmitate in topical microemulsions. Int J Pharm. 2001; 222:271–279.


25. Semenzato A, Austria R, Dall'Aglio C, Bettero A. High-performance liquid chromatographic determination of ionic compounds in cosmetic emulsions: application to magnesium ascorbyl phosphate. J Chromatogr A. 1995; 705:385–389.


26. Alexander A, Dwivedi S, Ajazuddin , Giri TK, Saraf S, Saraf S, et al. Approaches for breaking the barriers of drug permeation through transdermal drug delivery. J Control Release. 2012; 164:26–40. DOI: 10.1016/j.jconrel.2012.09.017.


27. Yong Z, Matthew PS, Russell JM, Michael J. Transdermal prodrug delivery for radionuclide decorporation: nonaqueous gel formulation development and in vitro and in vivo assessment. Drug Dev Res. 2013; 74:322–331.


28. Cormier M, Johnson B, Ameri M, Nyam K, Libiran L, Zhang DD, et al. Transdermal delivery of desmopressin using a coated microneedle array patch system. J Control Release. 2004; 97:503–511.


29. Donnelly RF, Garland MJ, Morrow DI, Migalska K, Singh TR, Majithiya R, et al. Optical coherence tomography is a valuable tool in the study of the effects of microneedle geometry on skin penetration characteristics and in-skin dissolution. J Control Release. 2010; 147:333–341. DOI: 10.1016/j.jconrel.2010.08.008.


30. Kim YC, Park JH, Prausnitz MR. Microneedles for drug and vaccine delivery. Adv Drug Deliv Rev. 2012; 64:1547–1568. DOI: 10.1016/j.addr.2012.04.005.


31. Gupta J, Gill HS, Andrews SN, Prausnitz MR. Kinetics of skin resealing after insertion of microneedles in human subjects. J Control Release. 2011; 154:148–155. DOI: 10.1016/j.jconrel.2011.05.021.


32. Wermeling DP, Banks SL, Hudson DA, Gill HS, Gupta J, Prausnitz MR, et al. Microneedles permit transdermal delivery of a skin-impermeant medication to humans. Proc Natl Acad Sci USA. 2008; 105:2058–2063. DOI: 10.1073/pnas.0710355105.


33. Kalluri H, Kolli CS, Banga AK. Characterization of microchannels created by metal microneedles: formation and closure. AAPS J. 2011; 13:473–481. DOI: 10.1208/s12248-011-9288-3.

