Abstract
Implants have been widely used in restorative treatment for patients who have undergone head and neck cancer surgery. With the development of combination treatment of head and neck cancer, radiotherapy has been a common means of therapy. However, it could induce various changes in hard and soft tissues and reduce the success and survival rate of the implants. Some research, using either animal models or clinical studies, have shown that certain strategies could be used for improving the survival rate of implants. In this review, we discussed the changes in both hard and soft tissues, which may reduce the survival rate of the implants, and the proposed methods for improving the survival rate of patients after radiotherapy.
Combination therapy is always performed on patients with head and neck cancer. Approximately 60%-80% of patients receive radiotherapy after surgery [1]. To increase their quality of life, restorative treatment is necessary. Among the different restorative methods, implants are widely chosen for their good functional recovery and aesthetic effects [2]. However, radiotherapy leads to a considerable number of difficulties in implant treatment. For example, the tissue in the irradiated area usually leads to a reduction of the vascular portion and cells and to hypoxia [3-5]. These changes lead to the destruction of osteoblasts and impaired bone modelling and remodelling which even cause osteoradionecrosis (ORN) [6,7]. Meanwhile, the radiotherapy may cause reduced saliva secretion and an imbalance of the peri-implant flora [8]. All these factors increase the risk of implant failure. To solve this problem, many strategies have been used in clinical practice, and some have achieved ideal effects. This review mainly focuses on the success rate of implants, tissue changes after radiotherapy, and effective adjuvant therapy methods, including hyperbaric oxygen (HBO), osteogenic growth peptide (OGP), and bone morphogenetic protein (BMP). The aim is to provide a useful guide to improve the success rate of implants in head and neck cancer patients after radiotherapy.
Radiotherapy is an important factor linked to implant failure. Studies from both animal subjects and human patients indicate that an irradiated bone has a greater risk of implant failure than a nonirradiated bone. This increase in risk may be up to 12 times greater [9,10]. There is much variation in the success rate reported amongst these data. A success rate as high as 99% in the mandible was reported by Keller et al. [11] in patients who had received radiotherapy, whereas rates as low as 70% were reported by Babin et al. [12]. A similar variation is seen in implants placed in the maxilla. The reported success rates vary from as high as 100% to as low as 40%. Buddula et al. [13] analyzed 48 patients who had prior head and neck radiation and had 271 dental implants, and reported that the 3-year survival rate was 238 implants (87.8%). Similar research was performed by Mancha et al. [14]; according to them, the overall 5-year survival rate in irradiated patients was 92.6%. Irradiated patients had a marginally significantly higher implant loss than nonirradiated patients. More importantly, they reported that the 5-year survival rate in the ORN group was only 48.3%. Recently, Linsen et al. [15] showed long-term results of implants following radical head and neck cancer surgery with adjuvant radiation therapy, and the overall 1-, 5-, and 10-year survival rates of all implants were 96.6%, 96.6%, and 86.9%, respectively. Based on the results of the research mentioned above, it was concluded that radiotherapy was the essential cause of implant failure. Irradiation can produce both early and late tissue changes. Early effects include those on the salivary glands, skin, and oral mucosa, whereas later effects involve bone changes leading to demineralization, fibrosis, increased susceptibility to infection, and finally, avascular necrosis [16-18]. Thus, the treatments against the changes in the soft and hard tissues after radiotherapy are meaningful for the success of dental implants in patients with head and neck cancer.
Both hard and soft tissues changed when the patients received radiotherapy. These changes are harmful to the restoration and the survival of implants. The radiotherapy could directly injure the remodeling system of the bone, including osteocytes, osteoblasts, and osteoclasts [19]. Meanwhile, it could also cause irradiation-induced vascular injury. Radiation injury to the fine vasculature of bone and its surrounding tissues first leads to hyperemia, followed by endarteritis, thrombosis, and a progressive occlusion and obliteration of small vessels, which would lead to a further cell number reduction and progressive fibrosis in bones [20,21]. All these changes further result in a significant loss of active osteoblasts and osteoclasts as well as periosteal fibrosis and the loss of remodeling elements.
The most severe potential complication of bone irradiation is ORN, which is considered to be an infectious process with compromised vascularity and minimal regenerative capabilities [22]. It usually occurs in the mandible and causes chronic pain and surface ulceration. The incidence of ORN of the mandible varies from 5% to 15%, and the incidence of ORN of the maxilla is much lower. ORN is closely associated with the implantation failure rate [23]. Mancha et al. [14] studied 225 implants placed in 30 patients who had received radiotherapy, and reported that irradiated patients had a marginally significantly higher implant loss than nonirradiated patients. The 5-year survival rate in the ORN group was 48.3% and that in the non-ORN group was 92.3%, with a statistically significant difference between both groups.
Besides the bones, the condition of the periodontium is directly related to the success and survival rate of the implants [24]. The cells and the vascular portion of the periodontal membrane decreased and the periodontal space widened after the patients received irradiation. The radiation-related changes in the cementum and the periodontal ligament may induce infection and increase the risk of hyposalivation, plaque accumulation, and the shift of oral microflora [25,26]. The effects of radiotherapy on the periodontium result in an increased risk of periodontal attachment loss as well as an increased risk of ORN development [27,28].
The change in salivary glands is another important factor that affects the survival rate of the implants. Radiotherapy could lead to a chronic salivary gland dysfunction, which is characterized by a reduced salivary flow and changes in the saliva composition [29]. The affected patients suffered from xerostomia, oral mucositis, difficulty in speaking, increase in oral pathologies, difficulty in chewing and swallowing food, and malnutrition due to a loss of the salivary flow [30,31]. Overall, these changes would destroy the environment of the oral cavity and ultimately, decrease the survival rate of the implants.
Radiotherapy causes different levels of damage to oral health. In addition to the changes in the hard and soft tissues in the oral cavity mentioned above, the damage of the oral mucosa, radiation caries, periodontal disease, dysfunction of muscles and joints, and imbalance of the nutritional status would all increase the risk of implant failure [32-35]. Thus, prevention and treatment considerations for irradiated patients are essential to improve the implant survival rate.
HBO therapy is a treatment modality in which a person breathes 100% O2 while exposed to increased atmospheric pressure [36]. It is the most studied and the most widely used therapy for improving the implant survival rate after radiotherapy adjuvant therapy. HBO therapy has been successfully used in the treatment of several disorders caused by tissue hypoxia, due to the extraoxygen supply to the tissues that it enables. For the bones after radiotherapy, HBO can cooperate with the basic fibroblast growth factor (bFGF) and protect the bone and bone marrow [37]. For the tissue damage after radiotherapy, HBO can improve the implant survival rate and achieve the ideal efficacy [38]. Granstrom et al. [39] retrospectively evaluated the implant survival of 631 implants installed in irradiated cancer patients over a 25-year period. The implant failure rate in HBO-treated patients was 8.5%, compared with the 40.2% of the non-HBO-treated group. In mouse models, it was found that HBO had significant effects on the treatment of irradiated tissues [40]. Through the study of histology and morphology, they found that HBO treatment could increase the formation of bone trabecular and bone exposure. In the irradiation region, HBO could significantly increase the velocity of blood flow. In addition, HBO is a good application for the treatment of ORN, hypo-salivation, radiation caries, and pain initiated by radiotherapy. Thus, HBO therapy can be considered an effective therapy for the prevention or treatment of the long-term complications of radiation therapy [41,42].
However, it has been insisted that HBO is not necessary in improving the survival rate of dental implants placed in irradiated bone because the incidence of ORN was very low (approximately 5%) and ideal survival rate of the implants could be achieved with prolonged healing period in previous studies. More importantly, HBO has some contraindications, including optic neuritis, pulmonary disease, claustrophobia, and epilepsy [43,44]. Correspondingly, the use of HBO can cause some complications, such as dysfunction of the eustachian tube, periosteal rupture, seizures, and decompression sickness [45,46]. In summary, HBO therapy has the potential to improve the implantation survival rate of patients received radiotherapy; however, the timing and indications for the use of HBO therapy should be in accord with specific conditions clinically.
BMPs are a group of growth factors known as cytokines and metabologens. Originally discovered by their ability to induce the formation of bone and cartilage, BMPs are now considered to constitute a group of pivotal morphogenetic signals, orchestrating tissue architecture throughout the body. These molecules primarily stimulate the differentiation of mesenchymal stem cells into chondroblasts and osteoblasts; they may contribute to the improvement in periodontal regenerative outcomes and are important for bone formation and renewal [47]. Wurzler et al. [48] reported that BMP had potential applications in reconstructive craninomaxillofacial surgery after irradiation for it could overcome the radiation-induced impairment of calvarial repair. Springer et al. [49] studied the application of BMP in the irradiated mandible and found that BMP could cooperate with bFGF and result in predictable bone generation. Other studies also identified that BMP could enhance the generation and repair of bones after radiotherapy [50,51].
In clinical applications, BMPs for clinical use are produced using recombinant DNA technology (recombinant human BMPs [rhBMPs]). rhBMP-2 and rhBMP-7 have been shown in clinical studies to be beneficial in the treatment of a variety of bone-related conditions, including spinal fusions and nonunions. In 2001, the U.S. Food and Drug Administration (FDA) approved rhBMP-7 (aka OP-1; Stryker Biotech, Hopkinton, MA, USA) for a humanitarian device exemption as an alternative to autograft in long bone nonunions. In 2004, the humanitarian device exemption was extended as an alternative to autograft for posterolateral fusion [52]. In 2002, rhBMP-2 (Infuse, Medtronic Inc., Minneapolis, MN, USA) was approved for anterior lumbar interbody fusions with a lumbar fusion device. In 2008, it was approved to repair posterolateral lumbar pseudarthrosis and open tibia shaft fractures with intramedullary nail fixation. In these products, BMPs are delivered to the site of the fracture by being incorporated into a bone implant and released gradually to allow bone formation, as the growth stimulation by BMPs must be localized and sustained for some weeks. The BMPs are eluted through a purified collagen matrix that is implanted in the site of the fracture. rhBMP-2 helps to grow bones better than any other rhBMP; therefore, it is considerably more widely used clinically. BMPs could promote and accelerate the new bone formation and maturation in the implant-bone interface [53,54]. Therefore, the use of the exogenous BMP bone tissue may improve the local content and the activity of BMP, and improve the implant survival rate after radiotherapy. Bovine BMP (bBMP) has been shown to increase the rate of osseointegration around cylindrical uncoated endosseous implants, as evidenced histomorphometrically four weeks after implantation in a dog model. The results revealed abundant lamellar bone formation around bBMP-coated implants. This bone was found adjacent to the implant threads and frequently entered the implant holes [55]. In a recent study, combined adenovirus-mediated human BMP-2 gene-modified bone marrow stromal cells with allograft enhanced the defect healing and improved the strength of implant fixation with osseointegration in a 3-mm bone defect around a titanium alloy implant [56].
The OGP is a 14-mer bone cell mitogen that increases bone formation and trabecular bone density and stimulates fracture healing. The overexpression of OGP has a markedly increased peak bone mass [57]. OGP is present in mammalian serum in micromolar concentrations mainly complexed to 2-macroglobulin. Upon its dissociation from the complex, it is proteolytically activated yielding the mitogenic C-terminal pentapeptide OGP [58]. In addition to stimulating bone formation, OGP potently enhances hematopoiesis. We have reported recently that the mitogenic action of OGP involves the activation of a Gi protein-MAP kinase signaling pathway [59]. Gurevitch et al. [60] reported that OGP could increase blood and bone marrow cellularity and enhance the engraftment of bone marrow transplants in irradiated mice. Other animal experiments showed that OGP could induce the transformation of cartilage callus into bone callus at the fracture site, promote the differentiation and maturation of the bone cells around implants, and accelerate the process of osseointegration [61,62]. However, at present, the research on OGP is still limited in terms of animal experiments, and the clinical applications of OGP need further study.
For patients with head and neck cancers, both combination therapy for the tumor and the follow-up implantation for functional reconstruction are necessary. Although radiotherapy is commonly applied to the cancerous tumor as an important means of therapy, it can cause various changes in both hard and soft tissues. All these changes are harmful to implant survival. Based on recent studies, some strategies, such as the use of HBO, BMPs, and OGP, might improve the implant survival rate (Fig. 1). However, every method has its limitations, and the applications and risks of different methods should be discussed further. Thus, the exploration of suitable ways to improve the retention of implants after radiotherapy needs further study.
Figures and Tables
Figure 1
Radiotherapy could induce various types of damage in both hard and soft tissues, and cause a considerable number of diseases and dysfunctions, such as osteoradionecrosis (ORN), radiation caries, changes in peri-implant flora, oral mucosa disease, periodontal disease, periodontium disease, salivary glands disease, dysfunction of muscles and joints, and imbalance of nutritional status. Some strategies, like the use of hyperbaric oxygen (HBO), bone morphogenetic protein (BMP), and osteogenic growth peptide (OGP), have been proposed to improve the implant survival rate after radiotherapy.
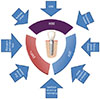
ACKNOWLEDGEMENTS
This work was supported by National Natural Science Foundation of China grants (No.: 81072215, 81001210, and 81172580), by the Fundamental Research Funds of the Central Universities of China (2011).
References
1. Harding SA, Hodder SC, Courtney DJ, Bryson PJ. Impact of perioperative hyperbaric oxygen therapy on the quality of life of maxillofacial patients who undergo surgery in irradiated fields. Int J Oral Maxillofac Surg. 2008; 37:617–624.


2. Tanaka TI, Chan HL, Tindle DI, Maceachern M, Oh TJ. Updated clinical considerations for dental implant therapy in irradiated head and neck cancer patients. J Prosthodont. 2013; 22:432–438.


4. Thavarajah N, Wong K, Zhang L, Bedard G, Wong E, Tsao M, et al. Continued success in providing timely palliative radiation therapy at the Rapid Response Radiotherapy Program: a review of 2008-2012. Curr Oncol. 2013; 20:e206–e211.


5. Ohri N, Shen X, Dicker AP, Doyle LA, Harrison AS, Showalter TN. Radiotherapy protocol deviations and clinical outcomes: a meta-analysis of cooperative group clinical trials. J Natl Cancer Inst. 2013; 105:387–393.


6. Koga DH, Salvajoli JV, Alves FA. Dental extractions and radiotherapy in head and neck oncology: review of the literature. Oral Dis. 2008; 14:40–44.


7. O'Dell K, Sinha U. Osteoradionecrosis. Oral Maxillofac Surg Clin North Am. 2011; 23:455–464.
8. Nagy K, Urban E, Fazekas O, Thurzo L, Nagy E. Controlled study of lactoperoxidase gel on oral flora and saliva in irradiated patients with oral cancer. J Craniofac Surg. 2007; 18:1157–1164.


9. Ihde S, Kopp S, Gundlach K, Konstantinovic VS. Effects of radiation therapy on craniofacial and dental implants: a review of the literature. Oral Surg Oral Med Oral Pathol Oral Radiol Endod. 2009; 107:56–65.


10. Yerit KC, Posch M, Seemann M, Hainich S, Dortbudak O, Turhani D, et al. Implant survival in mandibles of irradiated oral cancer patients. Clin Oral Implants Res. 2006; 17:337–344.


11. Keller EE, Tolman DE, Zuck SL, Eckert SE. Mandibular endosseous implants and autogenous bone grafting in irradiated tissue: a 10-year retrospective study. Int J Oral Maxillofac Implants. 1997; 12:800–813.
12. Babin RW, Ryu JH, Gantz BJ, Maynard JA. Survival of implanted irradiated cartilage. Otolaryngol Head Neck Surg. 1982; 90:75–80.


13. Buddula A, Assad DA, Salinas TJ, Garces YI. Survival of dental implants in native and grafted bone in irradiated head and neck cancer patients: a retrospective analysis. Indian J Dent Res. 2011; 22:644–648.


14. Mancha de la Plata M, Gias LN, Diez PM, Munoz-Guerra M, Gonzalez-Garcia R, Lee GY, et al. Osseointegrated implant rehabilitation of irradiated oral cancer patients. J Oral Maxillofac Surg. 2012; 70:1052–1063.


15. Linsen SS, Martini M, Stark H. Long-term results of endosteal implants following radical oral cancer surgery with and without adjuvant radiation therapy. Clin Implant Dent Relat Res. 2012; 14:250–258.


16. Colley HE, Eves PC, Pinnock A, Thornhill MH, Murdoch C. Tissue-engineered oral mucosa to study radiotherapy-induced oral mucositis. Int J Radiat Biol. 2013; 89:907–914.


17. Cheng SC, Wu VW, Kwong DL, Ying MT. Assessment of post-radiotherapy salivary glands. Br J Radiol. 2011; 84:393–402.


18. Khojastepour L, Bronoosh P, Zeinalzade M. Mandibular bone changes induced by head and neck radiotherapy. Indian J Dent Res. 2012; 23:774–777.


19. Silverman S Jr, Chierici G. Radiation therapy of oral carcinoma. I. Effects on oral tissues and management of the periodontium. J Periodontol. 1965; 36:478–484.


20. Mariano FV, Gondak RO, Santos-Silva AR, Correa MB, Almeida OP, Lopes MA. Reactive post-radiotherapy bone formation in the maxilla. J Craniofac Surg. 2013; 24:e43–e45.


21. Georgiou KR, Hui SK, Xian CJ. Regulatory pathways associated with bone loss and bone marrow adiposity caused by aging, chemotherapy, glucocorticoid therapy and radiotherapy. Am J Stem Cells. 2012; 1:205–224.
22. Lambade PN, Lambade D, Goel M. Osteoradionecrosis of the mandible: a review. Oral Maxillofac Surg. 2013; 17:243–249.


23. Wu G, Chen L, Zhu G, Wang Y. Low-intensity ultrasound accelerates mandibular implant bone integration in dogs with mandibular osteoradionecrosis. J Surg Res. 2013; 182:55–61.


24. Sugerman PB, Barber MT. Patient selection for endosseous dental implants: oral and systemic considerations. Int J Oral Maxillofac Implants. 2002; 17:191–201.
25. Marques MA, Dib LL. Periodontal changes in patients undergoing radiotherapy. J Periodontol. 2004; 75:1178–1187.


26. Colella G, Vuolo G, Siniscalchi G, Moscariello A, Itro A. Radiotherapy for maxillo-facial hemangiomas in children: dental and periodontal long term effects. Minerva Stomatol. 2005; 54:509–516.
27. Leung WK, Jin LJ, Samaranayake LP, Chiu GK. Subgingival microbiota of shallow periodontal pockets in individuals after head and neck irradiation. Oral Microbiol Immunol. 1998; 13:1–10.


28. Vissink A, Jansma J, Spijkervet FK, Burlage FR, Coppes RP. Oral sequelae of head and neck radiotherapy. Crit Rev Oral Biol Med. 2003; 14:199–212.
29. Blaha PJ, Reeve CM. Periodontal treatment for patients with cancer. Curr Opin Periodontol. 1994; 64–70.
30. Fiorentino A, Caivano R, Metallo V, Chiumento C, Cozzolino M, Califano G, et al. Parotid gland volumetric changes during intensity-modulated radiotherapy in head and neck cancer. Br J Radiol. 2012; 85:1415–1419.


31. Chen WC, Lai CH, Lee TF, Hung CH, Liu KC, Tsai MF, et al. Scintigraphic assessment of salivary function after intensity-modulated radiotherapy for head and neck cancer: correlations with parotid dose and quality of life. Oral Oncol. 2013; 49:42–48.


32. Şimşek G, Gurocak S, Karadag N, Karabulut AB, Demirtas E, Karatas E, et al. Protective effects of resveratrol on salivary gland damage induced by total body irradiation in rats. Laryngoscope. 2012; 122:2743–2748.


33. Santos-Silva AR, Rosa GB, Eduardo CP, Dias RB, Brandao TB. Increased risk for radiation-related caries in cancer patients using topical honey for the prevention of oral mucositis. Int J Oral Maxillofac Surg. 2011; 40:1335–1336.


34. Hong CH, Napenas JJ, Hodgson BD, Stokman MA, Mathers-Stauffer V, Elting LS, et al. A systematic review of dental disease in patients undergoing cancer therapy. Support Care Cancer. 2010; 18:1007–1021.


35. Sammartino G, Marenzi G, Cioffi I, Tete S, Mortellaro C. Implant therapy in irradiated patients. J Craniofac Surg. 2011; 22:443–445.


36. Carney AY. Hyperbaric oxygen therapy: an introduction. Crit Care Nurs Q. 2013; 36:274–279.
37. Wang X, Ding I, Xie H, Wu T, Wersto N, Huang K, et al. Hyperbaric oxygen and basic fibroblast growth factor promote growth of irradiated bone. Int J Radiat Oncol Biol Phys. 1998; 40:189–196.


38. Anderson L, Meraw S, Al-Hezaimi K, Wang HL. The influence of radiation therapy on dental implantology. Implant Dent. 2013; 22:31–38.


39. Granström G, Jacobsson M, Tjellstrom A. Titanium implants in irradiated tissue: benefits from hyperbaric oxygen. Int J Oral Maxillofac Implants. 1992; 7:15–25.
40. Chen X, Matsui Y, Ohno K, Michi K. Histomorphometric evaluation of the effect of hyperbaric oxygen treatment on healing around hydroxyapatite implants in irradiated rat bone. Int J Oral Maxillofac Implants. 1999; 14:61–68.
41. Marx RE, Ehler WJ, Tayapongsak P, Pierce LW. Relationship of oxygen dose to angiogenesis induction in irradiated tissue. Am J Surg. 1990; 160:519–524.
42. Johnsson AA, Sawaii T, Jacobsson M, Granstrom G, Turesson I. A histomorphometric and biomechanical study of the effect of delayed titanium implant placement in irradiated rabbit bone. Clin Implant Dent Relat Res. 2000; 2:42–49.


43. Donoff RB. Treatment of the irradiated patient with dental implants: the case against hyperbaric oxygen treatment. J Oral Maxillofac Surg. 2006; 64:819–822.
44. Wagner W, Esser E, Ostkamp K. Osseointegration of dental implants in patients with and without radiotherapy. Acta Oncol. 1998; 37:693–696.
45. Granström G. Placement of dental implants in irradiated bone: the case for using hyperbaric oxygen. J Oral Maxillofac Surg. 2006; 64:812–818.


46. Granström G. Osseointegration in irradiated cancer patients: an analysis with respect to implant failures. J Oral Maxillofac Surg. 2005; 63:579–585.
47. McGovern SC, Fong W, Wang JC. Can bone morphogenetic protein binding peptide increase efficiency of bone formation? Spine (Phila Pa 1976). 2010; 35:1655–1659.
48. Würzler KK, DeWeese TL, Sebald W, Reddi AH. Radiation-induced impairment of bone healing can be overcome by recombinant human bone morphogenetic protein-2. J Craniofac Surg. 1998; 9:131–137.


49. Springer IN, Niehoff P, Acil Y, Marget M, Lange A, Warnke PH, et al. BMP-2 and bFGF in an irradiated bone model. J Craniomaxillofac Surg. 2008; 36:210–217.


50. Zhang WB, Zheng LW, Chua DT, Cheung LK. Expression of bone morphogenetic protein, vascular endothelial growth factor, and basic fibroblast growth factor in irradiated mandibles during distraction osteogenesis. J Oral Maxillofac Surg. 2011; 69:2860–2871.


51. Iyomasa MM, Issa JP, de Queiroz Tavares ML, Pereira YC, Stuani MB, Mishima F, et al. Influence of low-level laser associated with osteogenic proteins recombinant human BMP-2 and Hevea brasiliensis on bone repair in Wistar rats. Microsc Res Tech. 2012; 75:117–125.


52. Ong KL, Villarraga ML, Lau E, Carreon LY, Kurtz SM, Glassman SD. Off-label use of bone morphogenetic proteins in the United States using administrative data. Spine (Phila Pa 1976). 2010; 35:1794–1800.


53. Razzouk S, Sarkis R. BMP-2: biological challenges to its clinical use. N Y State Dent J. 2012; 78:37–39.
54. Lauzon MA, Bergeron E, Marcos B, Faucheux N. Bone repair: new developments in growth factor delivery systems and their mathematical modeling. J Control Release. 2012; 162:502–520.


55. Johnson EE, Urist MR, Schmalzried TP, Chotivichit A, Huang HK, Finerman GA. Autogeneic cancellous bone grafts in extensive segmental ulnar defects in dogs. Effects of xenogeneic bovine bone morphogenetic protein without and with interposition of soft tissues and interruption of blood supply. Clin Orthop Relat Res. 1989; (243):254–265.
56. Yan MN, Dai KR, Tang TT, Zhu ZA, Lou JR. Reconstruction of peri-implant bone defects using impacted bone allograft and BMP-2 gene-modified bone marrow stromal cells. J Biomed Mater Res A. 2010; 93:304–313.


57. Bab IA. Regulatory role of osteogenic growth peptide in proliferation, osteogenesis, and hemopoiesis. Clin Orthop Relat Res. 1995; (313):64–68.
58. Chen Z, Chang M, Peng Y, Zhao L, Zhan Y, Wang L, et al. Regulation of endochondral ossification by osteogenic growth peptide C-terminal pentapeptide [OGP(10-14)]. Protein Pept Lett. 2009; 16:1074–1080.


59. Gabarin N, Gavish H, Muhlrad A, Chen YC, Namdar-Attar M, Nissenson RA, et al. Mitogenic G(i) protein-MAP kinase signaling cascade in MC3T3-E1 osteogenic cells: activation by C-terminal pentapeptide of osteogenic growth peptide [OGP(10-14)] and attenuation of activation by cAMP. J Cell Biochem. 2001; 81:594–603.


60. Gurevitch O, Slavin S, Muhlrad A, Shteyer A, Gazit D, Chorev M, et al. Osteogenic growth peptide increases blood and bone marrow cellularity and enhances engraftment of bone marrow transplants in mice. Blood. 1996; 88:4719–4724.

