Abstract
The use of dental implants has become a mainstay of rehabilitative and restorative dentistry. With an impressive clinical success rate, there remain a few minor clinical issues with the use of implants such as peri-implant mucositis and peri-implantitis. The use of laser technology with implants has a fascinating breadth of applications, beginning from their precision manufacturing to clinical uses for surgical site preparation, reducing pain and inflammation, and promoting osseointegration and tissue regeneration. This latter aspect is the focus of this review, which outlines various studies of implants and laser therapy in animal models. The use of low level light therapy or photobiomodulation has demonstrated its efficacy in these studies. Besides more research studies to understand its molecular mechanisms, significant efforts are needed to standardize the clinical dosing and delivery protocols for laser therapy to ensure the maximal efficacy and safety of this potent clinical tool for photobiomodulation.
Low-level light therapy (LLLT) or photobiomodulation (PBM) has become a routine treatment modality in many dental clinical settings and has been demonstrated to be efficacious in wound healing and tissue regeneration [1]. The term 'level' in LLLT is a poor descriptor, as it usually misrepresents the power and total energy utilized. The preferred term, PBM, better encompasses the inhibitory and stimulatory biological responses of low power laser therapy, both of which have therapeutic benefits. An operational definition of PBM suggests there is no significant increase in target tissue temperature above normal tissue temperature (37℃) [2]. These nonthermal therapeutic PBM effects are distinct from the heat-mediated laser tissue ablation effects, utilized for photodynamic therapy (PDT) and high power surgical applications. PBM effects should also be clearly distinguished from the antibacterial-antifungicidal effects of light therapy based on either endogenous fluorophores (color) in microorganisms or, more often, pretreatment with an exogenous photosensitizer dye. These disinfectant light effects are more akin to PDT than PBM, but do contribute to the beneficial therapeutic healing response.
Lasers at various nonionizing wavelengths are popularly used for PBM applications such as visible (660 nm), near-infrared (810 nm and 940 nm), and less frequently, midinfrared (1,040 nm, 2,940 nm, 9,400 nm, or 10,400 nm). Light emitting diodes and, less often, broad light sources are also becoming more popular due to better quality and control offered by the newer devices. Although the precise molecular mechanisms for PBM remain to be fully elucidated, their clinical effects on alleviating pain, reducing inflammation, and stimulating wound healing are well established [3,4]. The most significant challenge to using PBM in the clinic today is defining effective clinical dose parameters, specifically, the wavelength, irradiance, fluence, and delivery protocols for its use in a specific biological scenario to alleviate pain and inflammation or modulate the immune response to promote wound healing and tissue regeneration [4,5].
A major advancement in restorative clinical dentistry has been the increasingly popular use of dental implants. Besides their remarkable aesthetic and functional utility, the remarkable success rate (97.99%) of dental implants has significantly increased the reliability of restorative dental clinical care [6]. A few persistent clinical issues with implants currently are peri-implant mucositis (in the soft tissue) and peri-implantitis (of the bone-implant interface) [7,8]. Peri-implantitis is an inflammatory infection defined by bone loss of the peri-implant bone, with bleeding or bleeding upon examination of the soft tissue around the implant area, as well as pocket formation [8,9]. Peri-implant mucositis is classified as an inflammatory infection of only the soft tissue surrounding the implant site, with mucosal lesions, and lacking bone loss of the peri-implant bone [7,9].
Lasers have a fascinating range of applications for implants, from their initial stages of fabrication (e.g., laser sintering) to generating surface-topological modifications to promote ideal metal-biological interfaces. Further, lasers are used in the clinical setting where they are rapidly becoming a popular surgical tool to prepare soft (mucosa) and hard (bone) tissues for implant placement, to decontaminate surgical sites, and finally, to reduce pain and inflammation and promote healing (Fig. 1) [3,8,10,11]. The latter procedures are within the scope of PBM and can be performed either at the time of implant placement or during follow-up visits [10]. It should be emphasized that the lasers used in all these applications vary significantly with the type, dose, and manner of use. This article focuses on the published studies evaluating the potential utility of low power laser therapy for PBM in promoting clinical implants' success.
A literature search was performed in PubMed using the keywords "implants", "lasers", and "tissue regeneration" with a publication date up to April 2013. This resulted in 61 hits (Fig. 2). A second PubMed search was conducted using more specific keywords: "dental implants", "low-level laser therapy", and "tissue regeneration", which yielded additional 10 results.
The search results were further screened for relevance by excluding articles if they met any of the following criteria: (1) use of a surgical laser to cut into soft tissue or surgical preparation of hard or soft tissue, (2) laser treatment to treat the surface of the bone/implant-this includes surface decontamination/debridement, and/or (3) in vitro models. This literature review only focused on in vivo implant studies with the sole goal of evaluating relevant studies addressing PBM effects that may improve implant stability. Of the 71 total hits from the two searches, we identified 7 articles for more detailed analyses. The full text papers were assessed, and results were summarized based on the experimental animal model, namely, rabbits, nonhuman primates, and rodents.
Guzzardella et al. [12] studied the effects of the low-power laser on osseointegration in a rabbit model. Hydroxyapatite implants were placed into both femurs of the rabbits; the left femur was laser treated, and the right femur served as the internal control for each rabbit. The laser treatment was applied using a GaAlAs 780 nm for 10 minutes, equating to 300 J/cm2. The laser treatment was applied immediately after surgery and each day for five consecutive days. Histomorphometric and histologic analyses were conducted in the 4th and 8th week to assess osseointegration of the implant to the bone. The histologic and histomorphometric results showed that better osseointegration was achieved in the laser-treated group than the control in both 4th and 8th week (P<0.005 and P<0.001, respectively). In addition to improved osseointegration, the authors also observed that the laser-treated group demonstrated enhanced healing of the surgical site and reduced swelling as compared to the control group. In another study, Lopes et al. [13] assessed the bone quality and healing of rabbit tibial implants after laser treatments. Tibial implants were placed into fourteen rabbits; eight of the rabbits were laser treated, and the other six served as controls. Laser treatments were performed with an 830 nm laser. A total of seven treatments were performed every 48 hours for 15 days, resulting in total energy of 86 J per session. The rabbits were sacrificed at on the 15th, 30th, and 45th day, and bone healing was evaluated using Raman sectroscopy and scanning electron microscopy. The authors found that there was significantly higher discrepancy in calcium hydroxyapatite deposition on the 30th and 45th day between the irradiated and control group (P<0.001) [13]. The authors attributed these differences to the biostimulatory effects of laser treatments at the cellular level, as they increase adenosine triphosphate (ATP) production, osteoblast differentiation, and angiogenesis, potentially contributing towards improved bone mineral deposition. Another study by Campanha et al. [14] investigated the ability of PBM to improve implant stability. In this study, implants were inserted into the tibia of 30 rabbits and laser treatment was performed in 15 subjects. An 830 nm wavelength GaAlAs laser was used to treat four points around the implant with 21.5 J/cm2 per point for 51 seconds. The laser treatment was applied every 48 hours for seven treatments. The animals were sacrificed on the 15th, 30th, and 45th day. The tibia was removed and embedded onto an acrylic resin block, and the removal torque values (RTVs) of the implant were measured using an axial digital torquemeter. The RTV is the amount of force required to break the bone-implant union. The authors observed a statistically significant increase in the RTVs of laser-treated sites on the 15th day (P<0.013) and the 30th day (P<0.030), but not on the 45th day (P<0.215), compared to the controls.
The type and quality of the implant itself can have major implications for the integration of the implant into the biological system. An ideal implant would be biocompatible, could be placed with a minimally invasive procedure, and would have high clinical predictability with low postoperative risks [15]. In order to improve the latter implant characteristics, investigators have assessed the effects of various implant materials when combined with PBM.
Pinto et al. [16] assessed the effect of LLLT on the stability of two different orthodontic mini-implants in rabbits. Sixteen self-threading titanium fix implants and sixteen self-perforating implants were inserted into the right tibia of eight rabbits (two mini.implants per rabbit) and their left tibia served as the control. Laser treatment was performed every other day for 21 days after implantation, at two sites with a fluence of 90 J/cm2 for 25 seconds, with a total energy of 2.5 J and a total of 10 laser treatments. The stability of the implants was assessed by measuring the amount of force needed to remove the implant using a pull-out test from a device fabricated by the authors. This type of pull-out test is distinct from the RTV that is used in many implant studies to determine the amount of force required to remove an implant [17]. The mean pull-out force for the implants in the control group ranged from 108.58 N to 124.63 N, while the laser-treated groups had the mean pull-out force values ranging from 124.63 N to 177.39 N. Furthermore, their results indicate that there was a statistically significant difference (P<0.05) between the laser titaniumim plant group and all of the other groups, which the authors suggest is because of the increased contact of the surface of the titanium implant with bone due to its higher threadability. This study indicates that the choice of implant type, such as its shape and material, along with PBM therapy can have major clinical implications for successful integration and overall mechanical stability.
All four rabbit studies demonstrated improved implant performances following laser treatments. There was reduced swelling and better osseointegration of the implant-to-bone interface. These studies provide supportive evidence for the potential application of low-power laser therapy to improve implant healing, stability, and long-term success. However, the wavelength, dose, number of laser treatments, and duration of laser treatments vary substantially among these studies, indicating that there is a clear need to standardize the most efficacious treatment parameters to ensure optimal clinical therapeutic benefit.
The effect of low-power laser treatment on implant sites of hard tissue was evaluated by Dortbudak et al. [18] using a nonhuman primate model. The osteocyte count and viability were measured in the iliac crest of male baboons after implant insertion into each iliac crest of the baboon. The left iliac crest of the animal was treated for 1 minute with a 690 nm laser of 100 mW for 60 seconds for a total of 6 J. Five days after implantation, the bone and implant were removed, and the osteocyte viability and resorption rates were quantified using histomorphometric analysis. In the laser treated group, osteocyte viability was significantly higher (41.7%) than in the control group (34.4%, P<0.027). However, there were no differences in the bone resorption rates between the two groups. Although laser treatment did not appear to modulate the bone turnover rates, the authors suggested that the use of PBM may improve implant osseointegration based on the increased osteocyte viability they observed.
There have been many studies that demonstrate the biostimulatory effects of laser therapy to improve the bone-biomaterial interface and increased bone deposition after implantation. However, it is important to evaluate whether laser therapy can improve the functional and mechanical stability of implants. Maluf et al. [19] evaluated the mechanical stability of the bone-implant attachment after laser treatment in a mouse model. An implant was placed into one of the tibia in each of 24 mice. A 795 nm GaAlAs laser diode was used to treat four areas around the implant with 8 J/cm2 for a total of six treatment sessions every 48 hours. Both the control and irradiated groups were sacrificed on day 14, and the bones with implants were removed, fixed, and processed for hard tissue sectioning. The torque required to loosen the implant was measured using a digital torque machine. Results from this study revealed that the laser-treated groups had significantly higher torque removal values than the control (P<0.01). Based on this, the authors concluded that there is a significant increase in implant-to-bone attachment following laser treatments, suggesting that PBM improves bone repair and osseointegration.
Kim et al. [2] placed dental titanium implants in the tibia of 20 rats, divided into two groups. They measured the amount of receptor activator of nuclear factor kB (RANK), receptor activator of nuclear factor kB ligand (RANKL), and osteoprotegerin (OPG). The binding of RANKL (the ligand) to RANK (the receptor) potently stimulates osteoclast differentiation and resorptive activity [2]. OPG, on the other hand, is a cytokine that inhibits osteoclast activity by binding and sequestering RANK, hence reducing osteoclast differentiation. Laser treatments were performed with a GaAlAs diode laser with a wavelength of 808 nm. Six points were treated around the implant site with a duration of 10 seconds per point for a total of 960 mJ per point. Laser treatments were performed for 7 consecutive days after implant placement. The animals were sacrificed on the 1st, 3rd, 7th, 14th, and 21st day after treatment, and the decalcified sections were processed for routine immunohistochemical analysis to assess RANKL, OPG, and RANK expression. RANKL expression was present in both groups, but expression of RANKL appeared to be stronger and higher in the laser-treated group compared to the control group. Similar to RANKL, OPG was also expressed in both groups but at much higher levels in the laser-treated group. It is interesting to note that RANK expression was observed on the first day in the laser-treated group, while its expression was not detected until the 21st day in the control group. The bone densities of the two groups were also compared using histomorphometric analysis, and a significantly higher bone density was noted in the laser-treated group. It is also worth noting that the authors used scanning electron microscopy to assess the surface of the titanium implant and found that it was not damaged during laser treatment. This study indicates that laser therapy can modulate the specific molecular pathways involved in osteoclast proliferation and differentiation in vivo that could contribute to better osseous implant healing.
There appears to be some evidence for the use of PBM in improving implant stability in animal models. While lasers are extensively used in dentistry, their clinical applications in implant dentistry are currently largely limited to surface decontamination and surgical site preparation. PBM is becoming more recognized in the literature for its beneficial effects in enhancing implant stability, and therefore, more controlled human clinical studies should be pursued to validate its clinical efficacy in improving implant outcomes.
Animal models have been extensively used to improve our understanding of disease pathogenesis and have contributed immensely to discovering safe, effective therapeutic regimens for humans. A multitude of factors must be considered in designing and implementing animal studies. Appropriate animal models are chosen based on their physiological, anatomical, and genetic similarities to humans [20]. In animal implant studies, variables such as differences in bone density and composition, the rate of skeletal changes, and bone microstructures are additional factors that should be taken into consideration when designing an appropriate study [21].
In this review, we noted the use of mice, rats, baboons, and rabbit models to assess the effects of PBM on implant biology (Table 1). New Zealand white rabbits were the most common animals used to assess the stability of implants after treatment with lasers. Although Wang et al. [22] have observed that human fracture properties are different from those of rabbits, rabbits are still a useful intermediate model to use before testing in a larger animal [21]. Baboons and other nonhuman primates are ideal animal models due to their genetic and physiological similarity to humans, such as bone composition and bone fracture properties [23]. However, these primate models are expensive, not as readily available, difficult to house, and have greater ethical barriers for their use. Rodents have always been the most popular model organism for research, as they offer many advantages, such as ease of housing and maintenance, relatively homogenous genetic background in inbred strains; they offer a powerful mean to perform targeted gene studies. Advancements in genomic technologies have facilitated for the development of transgenic models allowing alteration of specific genes in a controlled spatio-temporal manner that has significantly contributed to our understanding of human diseases [24]. This capability to manipulate factors at the genetic level is key to our understanding of critical molecular players and to deriving causal relationships that can help develop targeted, efficacious clinical therapies.
Cell culture models have also immensely contributed to advancements in research and medicine. Despite the obvious limitation of being an isolated, simplified experimental model system, there are many advantages to using homotypic (single cell type) or heterotypic (multiple cell lineages) and in situ (graft and scaffold) models to study specific questions on cell behavior and function individually with minimal extraneous variables [25].
Implant success is dependent on both the health of the soft tissue collar (top) as well as proper interface of connective tissue to the implant surface [26]. Therefore, the effects of PBM on implants have been explored with regard to both the connective tissue cells, namely, the fibroblasts, as well as cells mediating osseointegration, namely, osteoblast-osteoclasts [12]. Khadra et al. [26] investigated the effect of laser therapy in the improvement of the attachment of human oral fibroblasts onto titanium surfaces, concluding that laser therapy can stimulate fibroblast activity and improve fibroblast attachment to a titanium implant surface, and suggesting that this may be a basis for the use of laser therapy to improve the implant-to-soft tissue interface. Besides soft tissue, cells that mediate osseointegration are also important. Many studies have looked at the use of laser treatment to stimulate osteoblast activity in vitro. For example, Dortbudak et al. [27] investigated the effect of low-level laser treatment on osteoblasts in vitro using bone marrow-derived mesenchymal stem cells, concluding that higher osteoblastic activity by laser treatment can contribute to improved implant osseointegration. The success and stability of an implant would be dependent on the ability of the implant to osseointegrate, indicated by the successful union of bone-to-implant material while maintaining structural and functional integrity of the host site [2,15,28]. PBM not only improves implant stability but it can also enhance healing around the surgical site through an increase in ATP synthesis and angiogenesis, reduce inflammation, and increase osteoblast proliferation [13,14,19].
Although this review noted the positive results of PBM used on implants in multiple animal studies, unfortunately, many negative studies are not published and rarely presented in professional forums. The gold standard to incorporate PBM in routine implant clinical management hinges on double-blinded, placebo-controlled, and multicentered randomized clinical trials. Given the popularity of implants in current dental practice and the increasing limitation in resources to perform such large clinical studies, innovative avenues to perform PBM research in implant dentistry via existing clinical networks such as the practice-based research network could be explored [29]. Implants are already an integral part of clinical dental practice with remarkable success rates. Modalities that promote these clinical successes to near perfection will not only improve patient satisfaction with esthetics and function, but bode well for the profession of dentistry.
Figures and Tables
Figure 1
Applications in implant dentistry including both manufacturing and clinical uses of lasers.
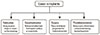
ACKNOWLEDGEMENTS
This work was funded by the Intramural Research Program, National Institute of Dental and Craniofacial Research, National Institutes of Health.
References
1. Peplow PV, Chung TY, Ryan B, Baxter GD. Laser photobiomodulation of gene expression and release of growth factors and cytokines from cells in culture: a review of human and animal studies. Photomed Laser Surg. 2011; 29:285–304.


2. Kim YD, Kim SS, Hwang DS, Kim SG, Kwon YH, Shin SH, et al. Effect of low-level laser treatment after installation of dental titanium implant-immunohistochemical study of RANKL, RANK, OPG: an experimental study in rats. Lasers Surg Med. 2007; 39:441–450.


3. Mester E, Mester AF, Mester A. The biomedical effects of laser application. Lasers Surg Med. 1985; 5:31–39.


4. Hahm E, Kulhari S, Arany PR. Targeting the pain, inflammation and immune (PII) axis: plausible rationale for LLLT. Photonics Lasers Med. 2012; 1:241–254.


5. Arany PR, Nayak RS, Hallikerimath S, Limaye AM, Kale AD, Kondaiah P. Activation of latent TGF-beta1 by low-power laser in vitro correlates with increased TGF-beta1 levels in laser-enhanced oral wound healing. Wound Repair Regen. 2007; 15:866–874.


6. Thalji G, Cooper LF. Molecular assessment of osseointegration in vivo: a review of the current literature. Int J Oral Maxillofac Implants. 2013; 28:e521–e534.


7. Peri-implant mucositis and peri-implantitis: a current understanding of their diagnoses and clinical implications. J Periodontol. 2013; 84:436–443.
8. Badran Z, Bories C, Struillou X, Saffarzadeh A, Verner C, Soueidan A. Er: YAG laser in the clinical management of severe peri-implantitis: a case report. J Oral Implantol. 2011; 37(Spec No):212–217.


9. Lang NP, Berglundh T. Working Group 4 of Seventh European Workshop on Periodontology. Periimplant diseases: where are we now? Consensus of the Seventh European Workshop on Periodontology. J Clin Periodontol. 2011; 38:Suppl 11. 178–181.


10. Arnabat-Dominguez J, Bragado-Novel M, Espana-Tost AJ, Berini-Aytes L, Gay-Escoda C. Advantages and esthetic results of erbium, chromium: yttrium-scandium-gallium-garnet laser application in second-stage implant surgery in patients with insufficient gingival attachment: a report of three cases. Lasers Med Sci. 2010; 25:459–464.


11. Botos S, Yousef H, Zweig B, Flinton R, Weiner S. The effects of laser microtexturing of the dental implant collar on crestal bone levels and peri-implant health. Int J Oral Maxillofac Implants. 2011; 26:492–498.
12. Guzzardella GA, Torricelli P, Nicoli Aldini N, Giardino R. Laser technology in orthopedics: preliminary study on low power laser therapy to improve the bone-biomaterial interface. Int J Artif Organs. 2001; 24:898–902.


13. Lopes CB, Pinheiro AL, Sathaiah S, Da Silva NS, Salgado MA. Infrared laser photobiomodulation (lambda 830 nm) on bone tissue around dental implants: a Raman spectroscopy and scanning electronic microscopy study in rabbits. Photomed Laser Surg. 2007; 25:96–101.


14. Campanha BP, Gallina C, Geremia T, Loro RC, Valiati R, Hubler R, et al. Low-level laser therapy for implants without initial stability. Photomed Laser Surg. 2010; 28:365–369.


15. Bengtson AL, Bengtson NG, Bengtson CR, Mendes FM, Pinheiro SR. Histological and radiographic evaluation of the muscle tissue of rats after implantation of bone morphogenic protein (rhBMP-2) in a scaffold of inorganic bone and after stimulation with low-power laser light. Indian J Dent Res. 2010; 21:420–424.


16. Pinto MR, dos Santos RL, Pithon MM, Araujo MT, Braga JP, Nojima LI. Influence of low-intensity laser therapy on the stability of orthodontic mini-implants: a study in rabbits. Oral Surg Oral Med Oral Pathol Oral Radiol. 2013; 115:e26–e30.
17. Favero LG, Pisoni A, Paganelli C. Removal torque of osseointegrated mini-implants: an in vivo evaluation. Eur J Orthod. 2007; 29:443–448.


18. Dortbudak O, Haas R, Mailath-Pokorny G. Effect of low-power laser irradiation on bony implant sites. Clin Oral Implants Res. 2002; 13:288–292.


19. Maluf AP, Maluf RP, Brito Cda R, Franca FM, de Brito RB Jr. Mechanical evaluation of the influence of low-level laser therapy in secondary stability of implants in mice shinbones. Lasers Med Sci. 2010; 25:693–698.


20. Simmons D. The use of animal models in studying genetic disease: transgenesis and induced mutation. Nature Educ. 2008; 1:70.
21. Mapara M, Thomas BS, Bhat KM. Rabbit as an animal model for experimental research. Dent Res J (Isfahan). 2012; 9:111–118.


22. Wang X, Mabrey JD, Agrawal CM. An interspecies comparison of bone fracture properties. Biomed Mater Eng. 1998; 8:1–9.
23. VandeBerg JL, Williams-Blangero S. Advantages and limitations of nonhuman primates as animal models in genetic research on complex diseases. J Med Primatol. 1997; 26:113–119.


24. Nguyen D, Xu T. The expanding role of mouse genetics for understanding human biology and disease. Dis Model Mech. 2008; 1:56–66.


25. Fell HB. Tissue culture and its contribution to biology and medicine. J Exp Biol. 1972; 57:1–13.


26. Khadra M, Kasem N, Lyngstadaas SP, Haanaes HR, Mustafa K. Laser therapy accelerates initial attachment and subsequent behaviour of human oral fibroblasts cultured on titanium implant material: a scanning electron microscope and histomorphometric analysis. Clin Oral Implants Res. 2005; 16:168–175.

