Abstract
Purpose
The purpose of this study was to evaluate the tensile strength of surgical synthetic absorbable sutures over a period of 14 days under simulated oral conditions.
Methods
Three suture materials (polyglycolic acid [PGA], polyglactin [PG] 910, and poly (glycolide-co-є-caprolactone) [PGC]) were used in 4-0 and 5-0 gauges. 210 suture samples (35 of each material and gauge) were used. All of the samples were tested preimmersion and 1 hour and 1, 3, 7, 10, and 14 days postimmersion. The tensile strength of each suture material and gauge was assessed. The point of breakage and the resorption pattern of the sutures were also assessed.
Results
During the first 24 hours of immersion, all 4-0 and 5-0 samples of PGA, PG 910, and PGC maintained their initial tensile strength. At baseline (preimmersion), there was a statistically significant (P<0.001) difference in the tensile strengths between the 4-0 and 5-0 gauge of PGA, PG 910, and PGC. PGA 4-0 showed the highest tensile strength until day 10. At 7 days, all the 4-0 sutures of the three materials had maintained their tensile strength with PGA 4-0 having significantly greater (P=0.003) tensile strength compared to PG.
Use of suture materials for wound closure is an ancient art that dates back to Egyptian scrolls of 3500 BC that describe the use of linen to close wounds. Use of animal hair, vegetable fibers, silk, leather, and gut have all been shown to be successfully used in wound closure [1].
A suture is a biomaterial device, either natural or synthetic, used to ligate blood vessels and approximate tissues together. The goals of wound closure include obliteration of dead space, even distribution of tension along deep suture lines, and maintenance of tensile strength across the wound until tissue tensile strength is adequate [2].
The healing of a surgical wound involves matrix formation that bridges the margins of the wound, supports cells and a regenerating vasculature, and restores the resistance of the tissues to functional stress [3].
Various factors that make suturing a different phenomenon in dentistry when compared to suturing of other parts of the body include the type of tissues involved, the constant presence of saliva, high tissue vascularization, and functions related to speech, mastication, and swallowing. Pertinent sutures require specific physical characteristics and properties, such as good tensile strength, dimensional stability, lack of memory, knot security, and sufficient flexibility to avoid damage to the oral mucosa. The strength and adherence of the sutured tissue increase over time, and investigators have noted that a significant increase in flap strength is achieved between 1 and 2 weeks. Deficiency in the strength of the suture material can result in untimely suture breakage, leading to poor adaptation of the surgical flaps and inducing the healing of tissues by secondary intention [4].
A variety of absorbable and nonabsorbable materials, ranging from animal derivatives to synthetic polymers, some of which can be fabricated in braided and/or monofilament form, are used for suturing. An advantage of absorbable sutures is that they generally do not require removal [5].
Commonly used bio-absorbable suture materials that have shown desirable properties in relation to tissue reactions are gut, polyglycolic acid (PGA), polyglactin (PG), and chromic gut (CG) [6]. Choosing the appropriate suture material should be based on the appropriate tensile strength, tissue biocompatibility, and resorption rates [7].
PGA is a polymer of glycolic acid that is often prepared by the polymerization of glycolide. PG is a synthetic, absorbable, braided suture consisting of 90% glycolide and 10% L-lactide [8]. Poliglecaprone 25 monofilament suture material, a copolymer of glycolide and e-caprolactone, is absorbed by hydrolysis in a period of 91 to 119 days, has excellent strength, minimal memory, and a great deal of malleability [9]. Studies have examined the tensile strength and resorption rates of different suture materials, and it has been found that the tensile strength of sutures decreased over time and was dependent on the rate of resorption [4].
Tensile strength is a measure of the time it takes for suturing material to lose 70% to 80% of its initial strength. Initial tensile strength is a measure of the amount of tension applied in a horizontal plane necessary to break the suturing material [10].
To the best of our knowledge, limited data focusing on the comparison of the tensile strengths of synthetic absorbable suture materials under simulated oral conditions is available. Hence this study compared the tensile strength of three different synthetic absorbable sutures.
This study evaluated three surgical synthetic absorbable suture materials: PGA, PG 910 and poly (glycolide-co-є-caprolactone) (PGC). Two gauges (4-0 and 5-0) of each type were used. Two hundred ten samples, 5 samples of each material and size, were selected based on their usage in periodontal surgery. The samples included were grouped as follows:
Solus (PGA, Lotus Surgicals Ltd., Dehradun, India): 35 samples of 4-0 and 35 samples of 5-0.
Solus 910 (PG 910, Lotus Surgicals Ltd.): 35 samples of 4-0 and 35 samples of 5-0. Monolus (PGC, Lotus Surgicals Ltd.): 35 samples of 4-0 and 35 samples of 5-0.
Each sample was tied with a square surgeon's knot around flexible rubber tubing to allow for a consistent loop size that would be practical during the mechanical analysis phase. Once tied, the sutures were carefully slid off the tubing for testing.
A biologic simulation of the oral environment was created in vitro by mixing 9 mL each of artificial saliva and sterile human serum (Human AB serum, Equitech-Bio, Kerrville, TX, USA) in a 1:1 ratio. This biologic solution was prepared and maintained at a pH of 7.4 to 8.1 in an incubator at 37℃. The samples were placed in a container containing the serum saliva mixture simulating the oral environment and maintained for a specified time in a nontensioned state.
Artificial saliva was prepared by mixing the following chemicals in one liter of distilled water:
1) Sodium azide: 0.75 g
2) Potassium monohydrogen phosphate: 0.804 g
3) Calcium chloride: 0.166 g
4) Magnesium chloride: 0.059 g
5) Sodium chloride: 1.02 g
The tensile strength of the suture samples was tested at specified points in time: preimmersion and 1 hour and 1, 3, 7, 10, and 14 days post immersion. The tensile strength was assessed using the Universal UltraTest machine (Mecmesin, Slinfold, UK). Each sample was taken from the container containing the saliva-serum solution and the suture was positioned with the knot pointed midway between both arms to allow for consistency in force distribution relative to the knot. Tensile strength assessment of the suture samples was done at a cross-head speed of 25 cm/min. Each specimen was stretched to failure and the maximum load was recorded in Newtons (N) and tabulated for analysis. The point of breakage for each sample of each material was assessed at a magnification of ×10 using a microscope (Labovision, Olympus Co., Tokyo, Japan) with an attached digital camera.
A descriptive statistical analysis was carried out in the present study. The results of continuous measurements are presented as mean±standard deviation (min-max) and results of categorical measurements are presented as number (%). Significance was assessed at a 5% level. A three-factor analysis of variance (ANOVA) was used to assess the tensile strength of materials at different thicknesses over time. Time was treated as a nonrepeated measures variable because the materials tested at each time frame were independent.
Statistical software, namely SAS 9.2 (SAS Institute Inc., Cary, NC, USA), SPSS ver. 15.0 (SPSS Inc., Chicago, IL, USA), Stata 10.1 (StataCorp LP., College Station, TX, USA), MedCalc 9.0.1 (MedCalc, Ostend, Belgium), Systat 12.0 (Systat Software Inc., Chicago, IL, USA) and R ver. 2.11.1 (R Foundation for Statistical Computing, Vienna, Austria) were used for the analysis of the data.
All suture loops were intact at the end of their respective soaking periods and were suitable for mechanical testing. Each suture demonstrated an obvious breaking point during mechanical testing on the Universal UltraTest Machine.
The tensile strength for three materials and two gauges at each point in time are listed in Table 1. The three-factor ANOVA showed a statistically significant (P<0.001, η2=0.263) three-way interaction among the materials over time for the two gauges (Table 1, Figs. 1 and 2).
Analyses were conducted to determine whether the tensile strength of the materials differed at baseline (under dry conditions).
At baseline (preimmersion), there was a statistically significant (P<0.001) difference in the tensile strengths between the 4-0 and 5-0 gauges of PGA, PG, and PGC.
The tensile strength of PGA, PG, and PGC 4-0 sutures was significantly greater than the 5-0 sutures until day 10. However, on day 14, both the gauges had negligible tensile strength in all three materials (Table 1). Both the 4-0 and 5-0 gauges of all three materials, PGA, PG, and PGC, maintained their tensile strength after 1 hour of immersion in the saliva serum mixture. Also, within the first 24 hours of immersion in the solution, the 4-0 and 5-0 samples of PGA, PG, and PGC maintained their initial levels of tensile strength.
The tensile strength for PGA, PG, and PGC 4-0 sutures was significantly greater (P<0.001) than the 5-0 sutures until day 10. However, on day 14 both the gauges had negligible tensile strength for the three materials (Table 1). Between the 4-0 gauge of the three materials, PGA had the maximum tensile strength (14.73 N) compared to PG and PGC at baseline. PGA had the maximum tensile strength, 13.03 N and 11.27 N, at day 7 and 10 respectively. There was almost no strength for all of the three materials at day 14.
For the 5-0 sutures, PGC showed maximum tensile strength at baseline. However, all of the three materials had a similar tensile strength until day 10. Day 14 showed a very negligible tensile strength for the three materials. Samples of PGA 4-0 showed 76.5% of the initial tensile strength at day 10 but only 3.1% on day 14. PG 4-0 showed 71.4% of the initial tensile strength at day 10 and 14.9% on day 14. PGC 4-0 samples had 56.2% of the initial tensile strength at day 10 but only 4% on day 14.
Pairwise comparison of the materials with the 4-0 gauge was carried out. Under dry conditions, even though PGA had higher strength, there was not much difference among the three materials.
After 1 hour of immersion, PGA showed a significantly higher (P<0.001) tensile strength than PG. PGA and PGC 4-0 sutures also showed a strongly significant difference (P<0.001) in their tensile strengths, with PGA having the higher strength. At day 1 postimmersion, the difference in the tensile strength of PGA and PG 4-0 sutures was strongly significant (P<0.001). The tensile strengths of PG and PGC 4-0 sutures also showed a strongly significant difference (P<0.001), but no significant difference in strengths between the PGA and PGC 4-0 sutures was found. Therefore, on day 1, both PGA and PGC had similar tensile strengths. At day 3 postimmersion, the tensile strengths of PGA and PG 4-0 sutures showed a strongly significant difference (P<0.001). PGA had a higher tensile strength than the PG. PGA had a higher tensile strength than PGC with a strongly significant difference (P<0.001). After 14 days of immersion, there was no statistical difference in the tensile strength among the three materials with the 4-0 gauge.
Pairwise comparison of the materials with the 5-0 gauge was carried out. Under dry conditions (preimmersion), PGA had a higher tensile strength than PG while PGC had a higher tensile strength compared to PG and PGA. After 1 hour of immersion, all three sutures had similar tensile strength. After 3 days, PGC 5-0 had a higher tensile strength than PG 5-0 and PGA 5-0. PGC 5-0 had a higher tensile strength than PGA 5-0 with a significant difference (P<0.001). PGC 5-0 showed the highest tensile strength at the end of day 3. At 7 days postimmersion, PGC 5-0 still maintained the highest tensile strength among the three sutures. After 10 days of immersion, there was no significant difference in the tensile strength among the three sutures. After 14 days of immersion, there was no statistical difference in the tensile strength among the three materials with a 5-0 gauge.
The samples were evaluated microscopically for the type of breakage following tensile testing. PGA, PG, and PGC all showed consistently even breakage. The samples were also evaluated for evidence of resorption throughout the length of the suture. PGA showed evidence of such resorption. PG showed splitting of the suture strand at day 14. PGC showed irregularity of the suture strand at day 14. All of the samples appeared to be translucent with time.
Synthetic absorbable sutures share a single indication, providing temporary and mechanical support until the natural tissue heals and regains strength. As the natural tissue heals, the degradable suture gradually weakens so that a gradual stress transfer occurs. Therefore, adjusting the rate of degradation of an absorbable suture to enable proper healing of the surrounding tissue is a major challenge in designing a temporary support. Clinically, most absorbable sutures are made of biodegradable linear aliphatic polyesters, of which PGA is the most important because most other biodegradable polymers are derived from PGA [11].
In this study, sutures made of PGA and other degradable polymers were investigated. PGA is absorbed by the body through a mechanism of biodegradation [12]. PG is absorbed by the process of hydrolysis. Another new material that was tested in the study was PGC. PGC is a good example of a material that exhibit two-stage degradation [11].
Owing to the fact that no study has been conducted, in vitro, to evaluate the tensile strength of PGC in comparison with PGA and PG 910, we chose this material to compare its tensile strength with the two commonly used synthetic absorbable sutures in periodontology.
The tying of knotsdy is as important as any other aspect of surgery [13]. When using synthetic absorbable sutures, it is recommended that the surgeon's knot be used to prevent knot untying [4]. Our study used the surgeon's knot for all of the samples for this reason, and it has been shown that knot-holding capacity reaches breaking force with all materials after the addition of a maximum of two throws to any of the starting knots [13].
Although various combinations of saliva and serum have been reported in the literature, a 1:1 ratio was used in our stusto simulate oral conditions [14]. Serum within the oral cavity is present in the form of gingival crevicular fluid. The ratio was selected based on the theory that suture material tied under the gingiva will come in contact with serum on the inside and saliva on the outside of the gingival tissues. Also, when used in maxillofacial surgery in the face and around the oral cavity, saliva may, in rare cases, come in contact with the sutures, and hence, the 1:1 ratio was selected to best mimic possible periodontal and maxillofacial surgical scenarios [4].
The pH of the medium is another important factor that gains more importance in the resorption of sutures. pH levels were found to influence the performance of absorbable more than nonabsorbable sutures [15]. Only alkaline conditions accelerate the degradation of synthetic, or "man-made," absorbable sutures [10]. The pH of our medium was maintained between 7.4 and 8.1 by checking it daily and replenishing the solution every 2 days when changes in the pH were noted.
The duration of our study and the selection of testing points were based on clinical relevance; the study was conducted for only 2 weeks because the sutures for most periodontal procedures are removed at that time.
Of the three sutures used in the study, the PGA suture had the highest tensile strength at baseline. In the saliva serum solution, the PGA sutures maintained their tensile strength for the first 3 days but a significant reduction in the tensile strength was noted until day 14. However, in another study using Ringer's lactate solution, PGA showed no significant change in strength for 0 to 13 days [12]. The reason could be that in our study a saliva serum solution was used and the sutures were placed in the incubator at 37℃, which was not done in the other study. Thus temperature and type of solution could have an effect on the tensile properties of sutures.
PG sutures had the least tensile strength at baseline but they maintained their tensile strength until day 7 and 10, and had minimal strength by day 14. This was in agreement with another study, which reported reduced tensile strength of Vicryl when soaked in saliva compared to other liquids like soy, saline, or milk [8]. Saliva appears to enhance the degradation of the sutures, which results in the reduction in tensile strength.
PGC sutures had the second greatest tensile strength at baseline among the three sutures. The tensile strength of PGC was maintained until day 7 and decreased henceforth to almost 0.56 N on day 14. However, another study suggested that PGC can provide sufficient strength to allow wound healing during the initial stage [10]. PGC was suggested to be the appropriate material for intraoral surgical procedures like microdebrider-assisted extended uvuloplasty because it maintains high tensile strength until postoperative day seven but degrades rapidly by postoperative day 14 [10]. The reason could be that since PGC is a monofilament suture, it can maintain its tensile strength better than other multifilament sutures. However, PGA is one exception that shows a better tensile strength than a PGC suture.
In an evaluation of the tensile strength and knot stability of surgical sutures, bioabsorbable sutures were immersed in Hank's balanced salt solution for 14 days and a surgeon's knot was then attempted [16]. It was observed that the tensile strength following linear loading decreased significantly for the CG suture after 14 days. Unlike in that study, the sutures in our investigation were initially tied in tension and immersed in the solution in an attempt to mimic the clinical situation [16]. This difference in the approach could explain the variation in tensile strength between the studies.
The tensile strength of PGA was found to be superior to PG at baseline and until day 10, which was similar to that reported in another study [4] that carried out the same procedure as in our study. This confirms that the difference in the approach towards the strength examination can result in different tensile strength values.
Previous investigations [17,18] of PGA sutures showed excellent handling properties, high initial tensile strength, and less tissue reaction. PGA suture possesses some properties superior to other materials, in that it does not produce much tissue reaction and has a greater tensile strength than other materials with a similar diameter. It lasts longer than catgut, and disintegration can be precipitated because it is hydrophilic. It is easier to handle and easier to tie than silk and catgut and does not produce stitch marks like those materials [19]. PGC is a monofilament material and the risk of wicking is minimized, causing less tissue reaction, which gives it an edge over the other multifilament suture materials.
The present study is in agreement with study carried out in vitro [8] and shows a reduction in the tensile strength of PG sutures under simulated oral conditions; however, that study evaluated the tensile strength for a period of 35 days. In another study [20], PGC demonstrated a decrease in tensile strength from baseline until two weeks, which was in agreement with the present study. The use of various biological fluids was the difference between the two studies.
The study demonstrated that the 4-0 sutures were stronger and had greater tensile strength than the 5-0 sutures for all of the three materials. Among the 4-0 sutures, PGA showed the highest tensile strength at the end of day 10 and is a desirable suture if tensile strength is required after 10 days. All of the sutures lost their tensile strength by day 14. Microscopic evaluation suggested that all the three materials had even breakage.
Although the results of this in vitro study favor the use of synthetic absorbable sutures, suture selection in the oral cavity should be predicated upon the demands of the repair and the surgeon's preference.
Figures and Tables
Figure 1
Line graph showing a comparison of the decrease in tensile strength in three materials of the same gauge (4-0). PGA: polyglycolic acid, PG: polyglactin, PGC: poly (glycolide-co-є-caprolactone).
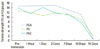
References
1. Parell GJ, Becker GD. Comparison of absorbable with nonabsorbable sutures in closure of facial skin wounds. Arch Facial Plast Surg. 2003; 5:488–490.


2. Pillai CK, Sharma CP. Review paper: absorbable polymeric surgical sutures: chemistry, production, properties, biodegradability, and performance. J Biomater Appl. 2010; 25:291–366.


3. Wikesjo UM, Nilveus RE, Selvig KA. Significance of early healing events on periodontal repair: a review. J Periodontol. 1992; 63:158–165.


4. Vasanthan A, Satheesh K, Hoopes W, Lucaci P, Williams K, Rapley J. Comparing suture strengths for clinical applications: a novel in vitro study. J Periodontol. 2009; 80:618–624.


5. Yaltirik M, Dedeoglu K, Bilgic B, Koray M, Ersev H, Issever H, et al. Comparison of four different suture materials in soft tissues of rats. Oral Dis. 2003; 9:284–286.


6. Karaca E, Hockenberger AS, Yildiz H. Investigating changes in mechanical properties and tissue reaction of silk, polyester, polyamide, and polypropylene sutures in vivo. Text Res J. 2005; 75:297–303.


7. Moore RL, Hill M. Suturing techniques for periodontal plastic surgery. Periodontol 2000. 1996; 11:103–111.


8. Ferguson RE Jr, Schuler K, Thornton BP, Vasconez HC, Rinker B. The effect of saliva and oral intake on the tensile properties of sutures: an experimental study. Ann Plast Surg. 2007; 58:268–272.


9. Nary Filho H, Matsumoto MA, Batista AC, Lopes LC, de Goes FC, Consolaro A. Comparative study of tissue response to polyglecaprone 25, polyglactin 910 and polytetrafluorethylene suture materials in rats. Braz Dent J. 2002; 13:86–91.


10. van Heerden J. Comparison of inflammatory response to polyglytone 6211 and polyglecaprone 25 in a rat model. S Afr Med J. 2005; 95:972–974.
11. Huang TW, Cheng PW, Chan YH, Wang CT, Fang KM, Young TH. Clinical and biomechanical analyses to select a suture material for uvulopalatopharyngeal surgery. Otolaryngol Head Neck Surg. 2010; 143:655–661.


12. Moser JB, Lautenschlager EP, Horbal BJ. Mechanical properties of polyglycolic acid sutures in oral surgery. J Dent Res. 1974; 53:804–808.


14. Zoller GO, Zentner A. Initial attachment of human gingival fibroblast-like cells in vitro to titanium surfaces pretreated with saliva and serum. Clin Oral Implants Res. 1996; 7:311–315.
15. Chu CC, Moncrief G. An in vitro evaluation of the stability of mechanical properties of surgical suture materials in various pH conditions. Ann Surg. 1983; 198:223–228.


16. Kim JC, Lee YK, Lim BS, Rhee SH, Yang HC. Comparison of tensile and knot security properties of surgical sutures. J Mater Sci Mater Med. 2007; 18:2363–2369.


17. Pavan A, Bosio M, Longo T. A comparative study of poly (glycolic acid) and catgut as suture materials: histomorphology and mechanical properties. J Biomed Mater Res. 1979; 13:477–496.


18. von Fraunhofer JA, Storey RJ, Masterson BJ. Tensile properties of suture materials. Biomaterials. 1988; 9:324–327.


19. Shetty PC, Dicksheet S, Scalea TM. Emergency department repair of hand lacerations using absorbable vicryl sutures. J Emerg Med. 1997; 15:673–674.


20. Tiyek I, Gemci R, Turkoglu S. Comparison of physical properties of sutures in medical liquids. Int J Phys Sci. 2011; 6:2158–2168.