Abstract
Purpose
This study evaluated the surface characteristics and bond strength produced using a novel technique for coating hydroxyapatite (HA) onto titanium implants.
Methods
HA was coated on the titanium implant surface using a super-high-speed (SHS) blasting method with highly purified HA. The coating was performed at a low temperature, unlike conventional HA coating methods. Coating thickness was measured. The novel HA-coated disc was fabricated. X-ray diffraction analysis was performed directly on the disc to evaluate crystallinity. Four novel HA-coated discs and four resorbable blast medium (RBM) discs were prepared. Their surface roughnesses and areas were measured. Five puretitanium, RBM-treated, and novel HA-coated discs were prepared. Contact angle was measured. Two-way analysis of variance and the post-hoc Scheffe's test were used to analyze differences between the groups, with those with a probability of P<0.05 considered to be statistically significant. To evaluate exfoliation of the coating layer, 7 sites on the mandibles from 7 mongrel dogs were used. Other sites were used for another research project. In total, seven novel HA-coated implants were placed 2 months after extraction of premolars according to the manufacturer's instructions. The dogs were sacrificed 8 weeks after implant surgery. Implants were removed using a ratchet driver. The surface of the retrieved implants was evaluated microscopically.
Surface modification of dental implants plays an important role in achieving rapid secondary stability and enhancing the bone-to-implant contact [1-4]. It also reduces the total treatment time required for the rehabilitation of missing teeth, which is beneficial both to dentists and their patients. Various techniques can be used to optimize the implant surface characteristics, including blasting, etching, titanium plasma spaying, and oxidizing.
Special interest has recently been paid to coating dental implants with hydroxyapatite (HA). HA has biocompatibility with and compositional similarities to natural bone [5-8]. HA-coated implants show higher survival rates and an earlier bone response than commercial pure-titanium and titanium-alloy implants [9].
A plasma-spraying method has been previously used to apply HA to the surface of titanium implants [10-13]. Despite the simplicity and versatility of this method, it has several inherent problems: easy exfoliation of the coating layer, vulnerability to bacterial infection, non-uniform coating thickness, and easy degradation in the human body. These inherent problems result in the rapid breakdown of osseointegration [14,15]. Various methods-including ion sputtering, ion plating, ion implantation, and ion-beam-associated deposition of the HA coating-have been introduced in attempt to overcome these drawbacks [16-19].
HA coating using a super-high-speed (SHS) blasting process is a novel method for increasing the bond strength between the coating layer and the substratum so as to prevent exfoliation of unstably coated HA from the titanium surface. A uniformly coated micron-thick HA layer could maintain the original microtexture of the implant surface. The aim of this study was to elucidate the surface characteristics of implants that are coated with HA using a novel technique.
Resorbable blast medium (RBM) surfaced dental implants (Dentis Co., Daegu, Korea) were prepared. HA was coated on the titanium implant surface using an SHS blasting method with highly purified HA (Sulzer Metco, Wohlen, Switzerland). The coating was performed at a low temperature, unlike conventional HA coating methods, with a thin layer of HA (0.1 to 5 µm) applied by blasting fine HA particles (0.1 to 50 µm) onto the surface. Carrier gas (nitrogen) was passed through a hopper containing the HA particles, and the particles and gas flowed into a vacuum chamber (at 10-1 torr) before blasting was performed onto the titanium implant surface at a high speed (100 m/sec). This process resulted in finer HA particles due to their fragmentation. The surface morphology of the implants was observed using field-emission scanning electron microscopy (FE-SEM; S-4300, Hitachi Ltd, Tokyo, Japan). A line profile analysis and surface-element mapping were performed using an energy-dispersive detector (Horiba, Kyoto, Japan).
X-ray diffraction analysis was performed directly on the novel HA-coated disc, which was mentioned above. A disc was placed in a special apparatus and aligned with the focal plane of a multipurpose X-ray diffractometer (MP-XRD; X'pert PRO XRD, PANalytical B.V., Almelo, The Netherlands). The scanning was made in the range of 20°<2θ<60° in 0.04° steps, with a counting time of 6 seconds per step. The percentages of crystalline HA, titanium, amorphous calcium phosphate (ACP), β-tricalcium phosphate (β-TCP), α-TCP, and tetracalcium phosphate (TTCP) were calculated based on the integrated areas under the curves that fitted the maximum peaks for each phase.
Four novel HA-coated discs and four RBM discs were prepared. Their surface roughnesses and areas were measured using optical profilometry (Wyko NT8000, Veeco, Tucson, AZ, USA).
Five pure-titanium, RBM-treated, and novel HA-coated discs were prepared. Saline at 36.5℃ was dropped onto each of the discs, and after 5 seconds the contact angles were measured using an automated contact-angle-measuring device (OCA 15 Plus, DataPhysics Instruments GmbH, Filderstadt, Germany), which included a video camera. Two-way analysis of variance and the post-hoc Scheffe's test were used to analyze differences between the groups; those with a probability of P<0.05 were considered to be statistically significant.
Seven male mongrel dogs, 18 to 24 months old and weighing about 30 kg each, were chosen. The dogs had intact dentition with no inflammation in the periodontium. The management, preparation, and surgical protocols applied to the animals followed the standard protocols approved by the Animal Care and Use Committee, Yonsei Medical Center, Seoul, Korea.
Novel HA-coated titanium implants (Dentis Co.) with micron-thick HA coatings were used. All premolars and the first molar on both sides of the mandible were extracted under general anesthesia and sterile conditions. Atropine (0.05 mg/kg) was injected subcutaneously, and xylazine (2 mg/kg; Rompun, Bayer Korea Ltd, Seoul, Korea) and ketamine hydrochloride (10 mg/kg; Ketalar, Yuhan, Seoul, Korea) were administered intravenously. Each dog was lying on a heating pad during surgery. An endotracheal tube was used for intubation, and 2% enflurane was administered. The physiological condition of each dog was monitored with an electrocardiogram.
In total, seven implants were placed on edentulous mandibles after a 2-month healing period. Other sites were used for another research project. The animals were anesthetized as described above. A crestal incision was made and a full mucoperiosteal flap was raised. Surgical preparation was performed according to the manufacturer's instructions. All HA-coated implants were inserted with a maximum force of over 30 N. The postoperative care was similar to that provided after tooth extraction. The sutures were removed after 7 to 10 days, and the dogs were fed a soft diet for 2 weeks. The dogs were sacrificed with an anesthesia overdose 8 weeks after surgery. The implants were removed using a ratchet driver. Following rinsing, the retrieved implants were observed in a top-down approach using FE-SEM and scanning transmission electron microscopy (HD-2300, Hitachi Ltd).
The thickness of the coating layer was within the range of 1 to 2 µm in all of the specimens (Fig. 1). The thin HA layer had formed evenly on the RBM surface (Fig. 2A), and the roughness of the implant surface was preserved (Fig. 2B). The spectrum data from the analysis of the surface elements revealed a calcium-to-phosphate ratio of 1.66, which is essentially the same as the stoichiometric value for HA (i.e., 1.67).
The crystallinity of HA on the specimen disc was 95% in the MP-XRD analysis (Fig. 3). The peak for titanium, which constituted the basic material of the implant, was found because the HA layer was very thin. The peaks for HA, α-TCP, β-TCP, TTCP, and ACP were not found.
The surface roughness was 1.83±0.06 µm (mean±standard deviation) for novel HA-coated titanium discs and 1.46±0.13 µm for RBM titanium discs, as revealed by optical-profile analysis. The surface areas of the HA-coated and RBM titanium discs were 277.80±76.28% and 60.24±4.15% greater, respectively, than the surface areas of the discs with a smooth titanium surface.
The contact angle was significantly lower for novel HA-coated titanium discs (28.70±5.66°) than for the RBM and pure-titanium discs (103.54±3.19° and 94.24±3.09°, respectively, P<0.0001; Fig. 4).
No exfoliated HA was found on the bony socket wall following the removal of the fixture. FE-SEM observations of the retrieved implants revealed that the HA-coated microstructure remained intact (Fig. 5).
Review articles have reported a positive correlation between surface roughness and osseointegration [1-4]. Albrektsson and Wennerberg [20] suggested the following classification based on the average surface roughness (Sa): smooth surface (Sa<0.5 µm), minimally rough surface (Sa=0.5-1 µm), moderately rough surface (Sa=1-2 µm), and rough surface (Sa>2 µm). The bone response was best for moderately rough surfaces. An appropriate surface roughness can be achieved using several methods, including HA coating. The advantages of HA-coated implants have been reported previously [5-8]. However, a thick or irregular coating layer may modify the surface roughness so as to produce an unwanted irregular texture. Therefore, it is crucial to ensure that the coating is both thin and uniform so that the original surface roughness is maintained.
The use of FE-SEM in the present study confirmed that the specimens had HA coating thicknesses within the range from 1 to 2 µm, and that there were no identifiable changes in the surface roughness. It has been reported that establishing a uniform micron-thick HA layer can prevent exfoliation of the coating layer more effectively than a thicker coating layer [21-23]. The present study found that implants with a micron-thick HA coating layer did not exhibit any HA exfoliation after they were removed from the bone. Thus, it can be assumed that a high bond strength between the coating layer and the titanium implant surface was obtained when using the novel HA coating technique employed in the present study. However, future studies should perform tensile strength testing in order to confirm these results.
Another purpose of roughening the implant surface is to increase the surface area that is in contact with bone. In the present study, the HA coating process resulted in a mean surface area gain of 277.8%, which could provide a better environment for biological molecules and bone-forming cells to attach to the implant surface.
A highly crystalline HA layer is also critical for the attachment of bone-forming cells in the initial healing phase [21]. The phase of ACP formation should be minimized because this hampers the proliferation and differentiation of osteoblast cells and weakens the bond strength. A previous study attempted to coat calcium phosphate using laser ablation and found that a thin and densely crystalline HA base layer was more resistant to dissolution than was a thick, plasma-sprayed base layer [22-24]. In the present study, the coating layer comprised densely crystalline HA without amorphous HA, and hence it can be assumed that our coating will be highly resistant to dissolution.
In the present study, the SHS blasting method was performed at room temperature. It is known that heat treatment during coating can produce microcracking and weakness of the coating layer due to differences in thermal expansion during the cooling process [23]. By performing the blasting at room temperature, we reduced the probability of thermal change, which could have contributed to the high crystallinity of the HA (95%) on the disc surface.
The surface charge and hydrophilicity of an implant have been known to influence osseointegration [25-27]. The pure-titanium and RBM surfaces in our study exhibited marked hydrophobicity, but application of an HA coating reduced the contact angle significantly (to 28.70±5.66°). Rapid hydration of the oxide layer could facilitate the adhesion of biomolecules [25] and enhance bone apposition in the early healing phase [26,27]. Therefore, the HA coating layer with enhanced wettability produced in this study is expected to accelerate osseointegration.
In conclusion, an SHS blasting method was used to apply a thin and uniform coating of highly crystalline HA over an RBM titanium surface with no deformation of the microtexture. The coating exhibited high bond strength and excellent wettability properties.
Figures and Tables
Figure 1
Thickness of the hydroxyapatite (HA) coating layer, as observed using field-emission scanning electron microscopy. The titanium substrate was coated with a thin HA layer whose thickness was within the range of 1 to 2 µm.
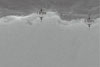
Figure 2
Surface topography of the implant, as observed using field-emission scanning electron microscopy: (A) Original magnification, ×50. (B) Original magnification, ×5,000.
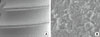
Figure 3
Surface hydroxyapatite (HA) crystallinity analysis. Multipurpose X-ray diffractometer analysis revealed that only HA was present on the disc surface.
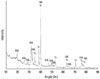
References
1. Chaffee NR, Cooper LF. Fixed partial dentures combining both resin-bonded and conventional retainers: a clinical report. J Prosthet Dent. 2000. 83:272–275.
2. Shalabi MM, Gortemaker A, Van't Hof MA, Jansen JA, Creugers NH. Implant surface roughness and bone healing: a systematic review. J Dent Res. 2006. 85:496–500.


3. Wennerberg A, Albrektsson T. Effects of titanium surface topography on bone integration: a systematic review. Clin Oral Implants Res. 2009. 20:Suppl 4. 172–184.


4. Wennerberg A, Albrektsson T. On implant surfaces: a review of current knowledge and opinions. Int J Oral Maxillofac Implants. 2010. 25:63–74.


5. de Groot K, Geesink R, Klein CP, Serekian P. Plasma sprayed coatings of hydroxylapatite. J Biomed Mater Res. 1987. 21:1375–1381.


6. Thomas KA, Kay JF, Cook SD, Jarcho M. The effect of surface macrotexture and hydroxylapatite coating on the mechanical strengths and histologic profiles of titanium implant materials. J Biomed Mater Res. 1987. 21:1395–1414.


7. Wang CY, Zhao BH, Ai HJ, Wang YW. Comparison of biological characteristics of mesenchymal stem cells grown on two different titanium implant surfaces. Biomed Mater. 2008. 3:015004.


8. Park YS, Yi KY, Lee IS, Han CH, Jung YC. The effects of ion beam-assisted deposition of hydroxyapatite on the grit-blasted surface of endosseous implants in rabbit tibiae. Int J Oral Maxillofac Implants. 2005. 20:31–38.


9. Gottlander M, Albrektsson T. Histomorphometric studies of hydroxylapatite-coated and uncoated CP titanium threaded implants in bone. Int J Oral Maxillofac Implants. 1991. 6:399–404.


10. Ji H, Marquis PM. Effect of heat treatment on the microstructure of plasma-sprayed hydroxyapatite coating. Biomaterials. 1993. 14:64–68.


11. Zyman Z, Weng J, Liu X, Zhang X, Ma Z. Amorphous phase and morphological structure of hydroxyapatite plasma coatings. Biomaterials. 1993. 14:225–228.


12. Chen J, Wolke JG, de Groot K. Microstructure and crystallinity in hydroxyapatite coatings. Biomaterials. 1994. 15:396–399.
13. Tsui YC, Doyle C, Clyne TW. Plasma sprayed hydroxyapatite coatings on titanium substrates. Part 2: optimisation of coating properties. Biomaterials. 1998. 19:2031–2043.
14. Biesbrock AR, Edgerton M. Evaluation of the clinical predictability of hydroxyapatite-coated endosseous dental implants: a review of the literature. Int J Oral Maxillofac Implants. 1995. 10:712–720.


15. Liao H, Fartash B, Li J. Stability of hydroxyapatite-coatings on titanium oral implants (IMZ). 2 retrieved cases. Clin Oral Implants Res. 1997. 8:68–72.


16. Ong JL, Lucas LC, Lacefield WR, Rigney ED. Structure, solubility and bond strength of thin calcium phosphate coatings produced by ion beam sputter deposition. Biomaterials. 1992. 13:249–254.


17. Yoshinari M, Ohtsuka Y, Derand T. Thin hydroxyapatite coating produced by the ion beam dynamic mixing method. Biomaterials. 1994. 15:529–535.


18. van Dijk K, Schaeken HG, Wolke JG, Jansen JA. Influence of annealing temperature on RF magnetron sputtered calcium phosphate coatings. Biomaterials. 1996. 17:405–410.


19. Singh RK, Qian F, Nagabushnam V, Damodaran R, Moudgil BM. Excimer laser deposition of hydroxyapatite thin films. Biomaterials. 1994. 15:522–528.


20. Albrektsson T, Wennerberg A. Oral implant surfaces: Part 1--review focusing on topographic and chemical properties of different surfaces and in vivo responses to them. Int J Prosthodont. 2004. 17:536–543.


21. Yang Y, Bumgardner JD, Cavin R, Carnes DL, Ong JL. Osteoblast precursor cell attachment on heat-treated calcium phosphate coatings. J Dent Res. 2003. 82:449–453.


22. Katto M, Ishibashi K, Kurosawa K, Yokotani A, Kubodera S, Kameyama A, et al. Crystallized hydroxyapatite coatings deposited by PLD with targets of different densities. J Phys: Conf Ser. 2007. 59:75–78.


23. Cleries L, Fernandez-Pradas JM, Sardin G, Morenza JL. Dissolution behaviour of calcium phosphate coatings obtained by laser ablation. Biomaterials. 1998. 19:1483–1487.


24. Arias JL, Garcia-Sanz FJ, Mayor MB, Chiussi S, Pou J, Leon B, et al. Physicochemical properties of calcium phosphate coatings produced by pulsed laser deposition at different water vapour pressures. Biomaterials. 1998. 19:883–888.


25. Rupp F, Scheideler L, Olshanska N, de Wild M, Wieland M, Geis-Gerstorfer J. Enhancing surface free energy and hydrophilicity through chemical modification of microstructured titanium implant surfaces. J Biomed Mater Res A. 2006. 76:323–334.

