Abstract
Purpose
(-)-epigallocatechin-3-gallate (EGCG) has been reported to exert anti-inflammatory and antibacterial effects in periodontitis. However, its exact mechanism of action has yet to be determined. The present in vitro study evaluated the anti-inflammatory effects of EGCG on human periodontal ligament fibroblasts (hPDLFs) and human periodontal ligament stem cells (hPDLSCs) affected by bacterial lipopolysaccharide (LPS) extracted from Porphyromonas gingivalis.
Methods
hPDLFs and hPDLSCs were extracted from healthy young adults and were treated with EGCG and/or P. gingivalis LPS. After 1, 3, 5, and 7 days from treatment, cytotoxic and proliferative effects were evaluated using a 3-(4,5-dimethylthiazol-2-yl)-2,5-diphenyltetrazolium bromide assay and bromodeoxyuridine assay, respectively. And then, the gene expressions of hPDLFs and hPDLSCs were observed for interleukin (IL)-1β, IL-6, tumor necrosis factor (TNF)-α, osteoprotegerin (OPG), receptor activator of nuclear factor kappa-B ligand (RANKL), and RANKL/OPG using real-time polymerase chain reaction (PCR) at 0, 6, 24, and 48 hours after treatment. The experiments were performed with the following groups for hPDLFs and hPDLSCs; 1) No treat, 2) EGCG alone, 3) P. gingivalis LPS alone, 4) EGCG+P. gingivalis LPS.
Results
The 20 µM of EGCG and 20 µg/mL of P. gingivalis LPS had the lowest cytotoxic effects, so those concentrations were used for further experiments. The proliferations of hPDLFs and hPDLSCs increased in all groups, though the 'EGCG alone' showed less increase. In real-time PCR, the hPDLFs and hPDLSCs of 'EGCG alone' showed similar gene expressions to those cells of 'no treat'. The gene expressions of 'P. gingivalis LPS alone' in both hPDLFs and hPDLSCs were highly increased at 6 hours for IL-1β, IL-6, TNF-α, RANKL, and RANKL/OPG, except the RANKL/OPG in hPDLSCs. However, those increased gene expressions were down-regulated in 'EGCG+P. gingivalis LPS' by the additional treatment of EGCG.
Periodontitis is one of the most widespread infectious diseases, and the associated periodontal tissue destruction is caused directly from the presence of periodontal pathogenic bacteria. Periodontal pathogens are considered to chronically activate inflammatory cells and osteoclasts, leading to gingival attachment loss and alveolar bone destruction. Yamamoto et al. [1] reported that human periodontal ligament (hPDL) cells may play an important role in the production of inflammatory cytokines in periodontal diseases. Furthermore, periodontal ligament (PDL) stem cells are regarded to be highly proliferative and capable of regenerating cementum- and PDL-like tissues, and to have therapeutic potential for the reconstruction of tissues destroyed by periodontal diseases [2].
Porphyromonas gingivalis is a gram-negative, black-pigmented anaerobic bacterium that is found in periodontal pockets, and is thought to play a principal role in periodontitis [3]. Lipopolysaccharide (LPS), a bacterial membrane protein, is known to be able to induce the secretion of high levels of several cytokines and proteinases from host cells, which leads to periodontal tissue destruction [4]. LPS stimulates inflammatory cells, such as neutrophils, macrophages, and fibroblasts, to secrete interleukin (IL)-1, IL-6, and tumor necrosis factor-alpha (TNF-α) [5,6], and these mediators have been reported to potently accelerate formation of osteoclast and bone resorption both in vivo and in vitro [7,8]. Moreover, the receptor activator of nuclear factor kappa-B (NF-κB) ligand (RANKL) stimulates bone resorption, whereas osteoprotegerin (OPG) inhibits it, and this bimolecular system is involved in periodontal tissue destruction. P. gingivalis was reported to induce RANKL and reduce OPG mRNA expressions in hPDL cells and gingival fibroblasts, resulting in an increased RANKL/OPG expression ratio [9].
Several studies demonstrated that (-)-epigallocatechin-3-gallate (EGCG) suppresses LPS-induced bone resorption by inhibiting IL-1β production or by directly inhibiting osteoclastogenesis [10-12]. Furthermore, EGCG was found to inhibit RANKL-induced osteoclast differentiation via the suppression of NF-κB transcriptional activity [13]. Several studies have reported the biological effects of EGCG, one of the major constituents of green tea, including its cell-preserving cytostatic properties, antibacterial and anti-inflammatory effects, and antioxidant effects [14-17]. In the field of dentistry, EGCG was demonstrated to improve the host conditions in periodontitis and periapical lesions, and those effects are due to the bactericidal effect of EGCG against periodontal pathogens [13,18], and due to the inhibitory effect on the production of related cytokines and their inflammatory pathways of gingival fibroblasts or osteoblasts [16,19].
The present in vitro study evaluated the anti-inflammatory effects of EGCG on human periodontal ligament fibroblasts (hPDLFs) and human periodontal ligament stem cells (hPDLSCs) affected by a periodontal pathogen (P. gingivalis).
We isolated hPDLFs and hPDLSCs from the nondecayed healthy teeth of three young adults (aged 11 to 19 years), that had been extracted for orthodontic purposes. The experimental protocols in this study were approved by the Ethics Committee of the College of Dentistry at Yonsei University, and before enrollment, written and informed consent was obtained from each subject.
After washing the extracted teeth with α-modified Eagle's medium (α-MEM; GIBCO, Grand Island, NY, USA) including 100 U/mL penicillin and 100 µg/mL streptomycin (GIBCO), the PDL tissues of the root from the middle-third to the apex were scraped and collected. For hPDLFs, the collected PDL tissues were minced and attached to the bottom of culture plates (T75 cell culture flask, Nunclon Delta Surface, NUNC, Roskilde, Denmark) and cultured with α-MEM supplemented with 10% fetal calf serum (GIBCO), 100 U/mL penicillin and 100 µg/mL streptomycin at 37℃ in 5% CO2. On the other hand, hPDLSCs were prepared using the enzymatic method by Seo et al. [2]. The minced small pieces of PDL tissues were digested with a solution of enzymes containing α-MEM (GIBCO), 2 mg/mL collagenase type I (Waco Pure Chemical Industries, Tokyo, Japan), and 1 mg/mL dispase (GIBCO) for 1 hour at 37℃. Then, single cell suspensions were collected using a 70-µm strainer (BD Falcon, BD Biosciences, Bedford, MA, USA). Cells (5×105) were cultured in a T75 flask at 37℃ in 5% CO2 with α-MEM containing 15% fatal calf serum, 10 µmol/mL L-ascorbic acid 2-phosphate (Sigma-Aldrich Co., St. Louis, MO, USA), 200 µmol/mL L-glutamine (GIBCO) and 100 U/mL penicillin, and 100 µg/mL streptomycin.
hPDLFs outgrown from PDL tissues and colonies of hPDLSCs were observed after approximately 14 days and 10 days, respectively. On days 21 and 14, respectively, the cells (hPDLFs and hPDLSCs) were detached by treating them with 0.25% trypsin-ethylenediaminetetraacetic acid solution (GIBCO) for 3 minutes at 37℃. Culturing medium was exchanged every three to four days, and the third or fourth passage of hPDLFs and hPDLSCs were used in this experiment.
The extraction of P. gingivalis LPS was performed as follows. Any bioactive extracellular material was removed by suspending P. gingivalis in saline, stirring the suspension gently for 1 hour at 4℃, and then harvesting them by centrifugation. After repeating this surface-washing process, primary extraction of the endotoxin was performed by using butanol as reported by Morrison and Leive [20]. In brief, the bacteria were suspended in 0.15 M NaCl (Sigma-Aldrich Co.) and an equal volume of butanol (Sigma-Aldrich Co.) was mixed in thoroughly at 4℃ for 10 minutes. After centrifuging the mixture at 35,000×g for 20 minutes, the aqueous supernatant was removed and saved. Then, the same volume of butanol was put into the insoluble residue, and approximately half the volume of saline was added. This butanol extraction was performed in tricplicate. To eliminate any insoluble residues, the combined aqueous extracts were centrifuged and dialyzed with distilled water at 4℃ for 8 hours, and lyophilization was performed. The products, however, also contained lipid-A-associated proteins as well as LPS, so a subsequent purification was prepared. The crude products were resuspended in water and ultracentrifuged at 105,000×g for 3 hours, and the procedures were repeated one more time before lyophilizing the endotoxin. Pure LPS was then prepared through the standard hot phenol-water method [21]. In short, endotoxin was resuspended into pyrogen-free distilled water, 90% phenol (Sigma-Aldrich Co.) was added, and the mixture was extracted twice at 68℃ for 20 minutes. After cooling, the supernatants were centrifuged at 35,000×g for 15 minutes, dialyzed extensively with distilled water at 4℃, and centrifuged again at 105,000×g for 3 hours. After subsequent freeze-drying of the aqueous LPS solution, the mixture of DNase (25 µg/mL; Sigma-Aldrich Co.) and RNase (25 µg/mL; Sigma-Aldrich Co.) in 0.1 M Tris-HCl (pH 8.0; Sigma-Aldrich Co.) was treated overnight at 37℃ to remove nucleic acids. Any contaminating protein was then hydrolyzed with proteinase K (50 µg/mL; Sigma-Aldrich Co.), followed by heating at 60℃ for 1 hour and incubation overnight at 37℃.
The cytotoxic activities of EGCG and P. gingivalis LPS on hPDLFs or hPDLSCs were confirmed through 3-(4,5-dimethylthiazol-2-yl)-2,5-diphenyltetrazolium bromide (MTT) assay. hPDLFs or hPDLSCs were seeded onto 96-well plates and then treated with EGCG (at 0, 0.1, 1, 10, 20, 50, or 100 µM) and/or P. gingivalis LPS (at 0, 0.1, 0.5, 1, 5, 10, 20, or 50 µg/mL) for 1, 3, 5, and 7 days. MTT reagent (Amresco, Solon, OH, USA) was put into each well at a final concentration of 0.5 mg/mL, and after a 3-hour incubation at 37℃, the supernatant of each well was replaced with the same volume of dimethyl sulfoxide (Amresco) and further incubated for 30 minutes. The optical density was measured at a wavelength of 570 nm with a microplate reader (enzyme-linked immunosorbent assay [ELISA]; Bio-Rad Laboratories Inc., Hercules, CA, USA).
Using a bromodeoxyuridine (BrdU) cell proliferation kit (Roche Diagnostics, Mannheim, Germany), the proliferation of hPDLFs and hPDLSCs was measured. Cells were seeded onto 96-well plates, and after 6 hours they were treated with EGCG or/and P. gingivalis LPS at the concentrations that were selected on the basis of the aforementioned MTT cytotoxicity assays as follows: 1) No treatment, 2) EGCG alone (20 µM), 3) P. gingivalis LPS alone (20 µg/mL), and 4) EGCG (20 µM) + P. gingivalis LPS (20 µg/mL). After 24 hours, the assay was performed according to the manufacturer's instructions. In brief, BrdU-labeling solution was added and cells were incubated for 2 hours at room temperature. Then, cells were fixed for 30 minutes and reincubated at room temperature with anti-BrdU-peroxidase antibody in the kit. After 90 minutes, cells were rinsed with phosphate-buffered saline and substrate solution was added until sufficient color had developed. The absorbance of the BrdU incorporation was measured by a microplate spectrophotometer (ELISA; Bio-Rad Laboratories Inc.) at 370 nm.
The mRNA of several cytokines was detected in order to analyze the changes in secretions of inflammatory factors under the influence of EGCG. The following representative inflammatory cytokines and osteoclastogenic factors involved in periodontitis were sought: IL-1β, IL-6, TNF-α, RANKL, and OPG [9,1,22,23]. Real-time PCR analysis was performed, and mRNA expressions of IL-1β, IL-6, TNF-α, RANKL, and OPG were detected. hPDLSCs and hPDLFs were seeded onto 6-well plates, and were treated with EGCG (20 µM) and/or P. gingivalis LPS (20 µg/mL) when they reached 80% confluence. At 6, 12, and 48 hours after treatment, the mRNA expressions of mediators were detected according to the manufacturer's instructions for each product. Using Trizol (Invitrogen, Carlsbad, CA, USA), cells were harvested and total RNA was prepared. For synthesis of cDNA, isolated RNA templates were mixed with Oligo dT (Maxime RT Premix, iNtRON Biotechnology Inc., Seongnam, Korea). The subsequent real-time PCR amplificatory reactions were performed using a SYBR real-time PCR kit (Premix Ex Taq, Takara Bio, Otsu, Japan), an ABI 7300 real-time PCR machine and software (Applied Biosystems, Foster City, CA, USA), and specific primers (Table 1).
The quantification of each mRNA was calculated relative to the results of an internal standard (β-actin). Each data production was performed in triplicate with the same total RNA.
The statistical analysis was performed with IBM SPSS ver. 19 (IBM Co., Armonk, NY, USA). The data in the present study was analyzed using a Wilcoxon rank-sum test, Kruskal-Wallis test, and Tukey's post hoc multiple comparison test, and the level of significance was set as 0.1 or 0.05 (P<0.1 or P<0.05).
The cell toxicities of EGCG and P. gingivalis LPS were tested on hPDLFs and hPDLSCs. The additional treatments of EGCG or P. gingivalis LPS produced significantly different cell viabilities of hPDLFs and hPDLSCs, especially at concentrations of 50 and 100 µM for EGCG, and 50 µg/mL for P. gingivalis LPS. For EGCG treatment, the cellular activities of hPDLFs and hPDLSCs were gradually increased until day 5 or 7, except the concentrations of 50 and 100 µM EGCG (Fig. 1A, B). The groups of 50 and 100 µM EGCG showed significantly low cell viabilities, while the cells of most concentrations (0, 0.1, 1, 10, and 20 µM EGCG) were comparable at each day. On day 7, the proliferations of hPDLSCs in 0.1, 1, 10, and 20 µM EGCG were less extensive than those of the untreated cells (0 µM EGCG), but there was no significant difference (P<0.1). On the other hand, the additional treatment of P. gingivalis LPS also produced a gradual increase in cell proliferation of hPDLFs and hPDLSCs, and they were similar to EGCG treatment. Comparing with other concentrations in hPDLFs, the only group of 50 µg/mL showed a significant decrease in cell viability at day 7 (Fig. 1C). However, the additional treatment of P. gingivalis LPS (0.1, 0.5, 1, 5, 10, 20, and 50 µg/mL) exerted effects on hPDLSCs, showing less activities at day 7 than untreated hPDLSCs (0 µg/mL) (Fig. 1D). Although there was a significant decrease in cell viabilities, the lower concentrations of P. gingivalis LPS (0.1, 0.5, 1, 5, 10, and 20 µg/mL) were relatively less influential than 50 µg/mL (P<0.05). Therefore, the less cytotoxic concentrations, 20 µM for EGCG and 20 µg/mL for P. gingivalis LPS, were used in the subsequent studies.
The effects of EGCG and/or P. gingivalis LPS on cell characteristics were determined by performing a cell-proliferation assay over 7 days with BrdU (Fig. 2). hPDLSCs and hPDLFs generally proliferated continuously during the experimental period, with an exponential increase occurring from days 5 to 7. More importantly, the population of hPDLSCs was significantly greater than that of hPDLFs. However, for both hPDLFs and hPDLSCs, the degree of conjugation with BrdU was significantly lower in the EGCG-treated groups ('EGCG alone' and 'EGCG+P. gingivalis LPS') than in the EGCG-untreated groups ('No treat' and 'P. gingivalis LPS alone'); BrdU conjugation was unaffected by the presence of P. gingivalis LPS (P<0.05). This suggests that the reduction in hPDLSC and hPDLF proliferation observed in the EGCG-treated groups is attributable to the presence of EGCG.
The inflamed condition of PDL cells was simulated by irritating hPDLFs and hPDLSCs with P. gingivalis LPS, and the anti-inflammatory and osteoclastogenesis-related effects of EGCG was tested by comparing cells treated with EGCG and those left untreated. Analysis was conducted using real-time PCR, and the presented data is the representative of separate experiments in triplicate. For inflammatory genes (IL-1β, IL-6, and TNF-α) in general, hPDLFs and hPDLSCs in 'EGCG alone' showed similar or lower gene expressions than those cells of 'No treat', but the gene expressions of 'P. gingivalis LPS alone' were highly increased at 6 hours (Fig. 3). The increased gene expressions were notably higher in IL-1β and IL-6 for hPDLFs and in IL-6 and TNF-α for hPDLSCs. Unlike 'P. gingivalis LPS alone', the 'EGCG+P. gingivalis LPS' group of cells showed similar gene expressions with the cells of 'no treat'. The increased gene expressions of 'P. gingivalis LPS alone' at 6 hours were down-regulated by the additional treatment with EGCG, though there was an increase in the IL-1β gene expression of hPDLSCs after 48 hours. Based on the RANKL and OPG gene expressions, the osteclastogenesis of the groups was evaluated with the ratio of RANKL/OPG (Fig. 4). The groups of 'no treat' and 'EGCG alone' showed similar and rarely increased gene expressions for both types of cells, hPDLFs and hPDLSCs. In the groups of 'P. gingivalis LPS alone', however, the gene expression of hPDLFs was up-regulated at 6 hours in RANKL, so the ratio of RANKL/OPG was higher than the other groups. On the other hand, the 'EGCG+P. gingivalis LPS' group showed much lower gene expression at 6 hours in RANKL and RANKL/OPG. For hPDLSCs, the 'P. gingivalis LPS alone' group showed much higher gene expressions at 6 hours in both RANKL and OPG. Although the RANKL gene was strongly expressed, the relative gene expressions of RANKL/OPG were not increased since that of the OPG gene was also strongly observed. However, those up-regulated gene expressions of RANKL and OPG were not observed in the 'EGCG+P. gingivalis LPS' group, and there was an increase in OPG gene expression at 48 hours.
The PDL fibroblast, which is the major constitutive cell in the PDL, is known to participate with other immunogenic cells in immune responses to resist the harmful action of oral bacteria and toxins. However, since multipotent mesenchymal stem cells have recently been found in PDL tissues, so it has been suggested that the hPDLSCs may play an important role in biologic reactions in the periodontium. Few studies have investigated the immune reaction of hPDLSCs against periodontal pathogens.
It was reported recently that EGCG can improve the periodontal condition in periodontitis and periapical lesions because of its bactericidal effects against periodontal pathogens [13,24]. With the aim of understanding the anti-inflammatory mechanisms of EGCG, several studies have been performed on various cells, such as human chondrocytes, intestinal epithelial cells, and gingival fibroblasts [19,25,26]. However, the effect of EGCG on PDL cells has yet to be established, and there are no reports on the effect of EGCG on the gene expressions of inflammatory cytokines in PDL cells. We therefore examined the effect of EGCG on the release of inflammatory cytokines from P. gingivalis-stimulated hPDLFs and hPDLSCs. We also determined the effect of EGCG on the proliferation of hPDLFs and hPDLSCs.
The cytotoxicities of P. gingivalis LPS and EGCG were tested using the MTT assay. Among the various concentrations of EGCG (0, 0.1, 1, 10, 20, 50, and 100 µM) and P. gingivalis LPS (0, 0.1, 0.5, 1, 5, 10, 20, and 50 µg/mL) tested, only 50 and 100 µM EGCG and 50 µg/mL P. gingivalis LPS exerted cytotoxic effects on the cell viability of hPDLFs and hPDLSCs. We tested the effects of both P. gingivalis LPS and EGCG on cell prolif-eration using the BrdU assay with the maximum concentrations that showed no cytotoxicity (i.e., 20 µg/mL and 20 µM, respectively).
The BrdU assay enables a more precise detection of changes in proliferation; the synthetic nucleoside BrdU can be incorporated into the newly synthesized DNA of replicating cells, and application of anti-BrdU can thus detect the newly proliferated cells. Although the number of both types of cells increased during the entire experimental period, the proliferation of hPDLSCs was greater than that of hPDLFs. This higher proliferation by hPDLSCs may be due to their potential for self-renewal, which is a typical property of stem cells.
On the other hand, the addition of EGCG to the culture medium reduced the cellular proliferation regardless of treatment with P. gingivalis LPS. Such an inhibitory effect of EGCG on cell proliferation might be attributable to its cytostatic nature. Previous studies have supported that EGCG pauses cell growth due to its ampiphathic property, which makes EGCG readily combine with cellular proteins, thus making possible the storage of intact cells [27-29]. Another study, however, has reported that EGCG suppresses tumorigenesis by interfering with signal transducer and transcription activator protein [30]. In chronic peridontitis, PDL cells including PDLF are involved in the breakdown of periodontal tissues by secreating inflammatory cytokines [4]. If the inflamed PDL cells that are releas-ing destructive inflammatory mediators could be decreased or at least limited in their rate increase during periodontitis, less destruction and better healing of periodontal tissues would be possible.
In the real-time PCR analysis, we observed the changes in gene expressions of hPDLFs and hPDLSCs, which were IL-1β, IL-6, TNF-α, RANKL, OPG, and the RANKL/OPG ratio. In previous reports, significantly large quantities of IL-1β, IL-6, and TNF-α were reportedly found at sites of periodontal disease, where they play a central role in the breakdown of periodontal tissues [22,31] by stimulating monocytes and fibroblasts to release extracellular matrix-degradable proteins [32], helping the maturation of B cells into immunoglobulin-producing plasma cells [33]. Therefore, those P. gingivalis LPS changes in gene expression induced by P. gingivalis LPS in this study may be the inflammatory reactions of hPDLFs and hPDLSCs. However, there was unexpected result, in that an exponential increase of IL-1β gene expression at 48 hours was observed in hPDLSCs of the 'EGCG + P. gingivalis LPS' group. We were not able to find related or similar results in other papers. Therefore, it further experiments are needed to understand the therapeutic mechanism by EGCG more thoroughly.
In addition, osteoclastogenesis, a differentiating and maturing procedure of osteoclasts, is the main mechanism underlying the break down of periodontal tissue [34] and IL-1β, IL-6, and TNF-α were demonstrated to be associated in tissue destruction by promoting the differentiation of osteoclast precursors and subsequently activating osteoclasts [35]. For these reasons, changes in osteoclastogenic mediators, RANKL and OPG, should also be detected as periodontal inflammatory reactors. RANKL, a surface-bond molecule, is a key factor in the differentiation and activation of osteoclasts, while OPG, a decoy receptor for RANKL, blocks RANKL by binding RANKL-RANK ligands of osteoclast precursors and osteoclasts [36], so the ratio of RANKL/OPG is a value to consider as a possible marker of osteoclastogenesis.
We therefore investigated the gene expressions of IL-1β, IL-6, TNF-α, RANKL, and OPG in hPDL cells stimulated by P. gingivalis LPS. At 6 hours after P. gingivalis LPS stimulation, the challenged hPDLFs exhibited up-regulated gene expressions of IL-1β, IL-6, TNF-α, and RANKL, and an increased RANKL/OPG ratio, suggesting a bone-destruction response. These results are consistent with those of other studies: Imatani et al. [37] found that P. gingivalis induced the secretion of IL-1β, IL-6, IL-8, and TNF-α by human gingival fibroblasts, and Yamamoto et al. [1] found that stimulation with P. gingivalis induced mRNA expressions of IL-1β, IL-6, IL-8, TNF-α, and RANKL in hPDL cells. Similar research has revealed that P. gingivalis-challenged PDL cells up-regulate RANKL and down-regulate OPG, resulting in a significantly higher RANKL/OPG ratio, and indicating a transient increase in their osteoclastogenic potential [9]. However, the gene expression levels of TNF-α, RANKL, and OPG in P. gingivalis-LPS-stimulated hPDLSCs were higher than those of IL-1β and IL-6. Moreover, although the RANKL gene was strongly expressed in P. gingivalis-LPS-stimulated hPDLSCs, the expression level of the OPG gene was also similarly high, indicating that the RANKL/OPG ratio was not changed markedly by P. gingivalis LPS treatment. These findings show that the main inflammatory reaction by hPDLSCs was due to increased TNF-α expression rather than IL-1β and IL-6 expressions, which is different from the responses in hPDLFs. Moreover, the osteoclastogenesis induced by hPDLSCs would be down-regulated in comparison to hPDLFs, since the compensative OPG was expressed more strongly in P. gingivalis-stimulated hPDLSCs. This is thought to be due to cellular differences between fibroblastic hPDLFs and stem-cell-like hPDLSCs.
It is known that mesenchymal stem cells have immune-modulating effects, and several studies have found that PDLSCs also have immune-suppressing potential by inhibiting the proliferation of monocytes and T cells [38]. Therefore, we assumed that the down-regulated osteoclastogenic RANKL/OPG ratio of hPDLSCs would be associated with the immune-modulating effect of stem cells, but further in-depth studies are required to investigate this relationship. The findings of such studies would be helpful for understanding the anti-inflammatory mechanisms of EGCG in periodontal inflammation.
Additional treatment with EGCG caused a down-regulation of the P. gingivalis-LPS-induced increase in the gene expressions of IL-1β, IL-6, TNF-α, RANKL, and OPG in both hPDLFs and hPDLSCs. The anti-inflammatory effects of EGCG have been reported mainly in fields other than dentistry, although a few studies have recently investigated this in the dental field. Nakanishi et al. [39] confirmed that treatment with EGCG causes down-regulation of the secretion of IL-6 by human dental pulp cells simulated by Escherichia coli LPS, and Hosokawa et al. [40] showed that EGCG can reduce IL-6 production by human gingival fibroblasts stimulated by TNF-14. In a clinical study, Hirasawa et al. [24] found significant reductions in markers of gingivitis after the slow-release application of EGCG over an 8-week period.
In conclusion, we treated hPDLFs and hPDLSCs with a major green tea extract, EGCG, and P. gingivalis LPS. The findings in the present study show that although EGCG treatment induces the down-regulation of cell proliferative activities, the hPDLFs and hPDLSCs showed up-regulated gene expression against P. gingivalis LPS stimulation, while EGCG decreased those up-regulated gene expressions of IL-1β, IL-6, TNF-α, as well as the osteoclastogenic gene expression of RANKL. Our results demonstrated that EGCG may exert an anti-inflammatory and antiosteoclastogenic effect in hPDLFs and hPDLSCs against a major pathogen of periodontits, P. gingivalis LPS.
Figures and Tables
Figure 1
Effects of (-)-epigallocatechin-3-gallate (EGCG) and Porphyromonas gingivalis lipopolysaccharide (LPS) on the viability of human periodontal ligament fibroblasts (hPDLFs) and human periodontal ligament stem cells (hPDLSCs). Most of the hPDLFs (A) and hPDLSCs (B) exhibited comparable cell viability in the EGCG-containing media (0, 0.1, 1, 10, and 20 µM) at days 3 to 7, except in 50 and 100 µM (P<0.1). The proliferation of hPDLFs (C) and hPDLSCs (D) treated with 50 µg/mL P. gingivalis LPS exhibited the most significant down-regulation at day 7, while remained unaffected for hPDLFs and less affected for hPDLSCs in other concentrations (i.e., 0, 0.1, 0.5, 1, 5, 10, and 20 µg/mL) (P<0.05). MTT: 3-(4,5-Dimethylthiazol-2-yl)-2,5-diphenyltetrazolium bromide, OD: optical density. a), b), or c)There is a statistically significant difference; a) vs. b), a) vs. c), and b) vs. c) (P<0.1). d), e), or f)There is a statistically significant difference; d) vs. e), d) vs. f), and e) vs. f) (P<0.1).
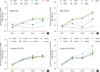
Figure 2
Proliferative changes of human periodontal ligament fibroblasts (hPDLFs) and human periodontal ligament stem cells (hPDLSCs) treated with Porphyromonas gingivalis lipopolysaccharide (LPS) and (-)-epigallocatechin-3-gallate (EGCG). In general, hPDLFs (A) and hPDLSCs (B) proliferated continuously during the experimental period, with an exponential increase in cell growth being observed from days 5 to 7. The cellular proliferation was generally higher in hPDLSCs than hPDLFs. However, for both hPDLFs and hPDLSCs, the degree of conjugation with bromodeoxyuridine (BrdU) was significantly lower in EGCG-treated groups than in EGCG-untreated groups. Conjugation with BrdU was not affected by treatment with P. gingivalis LPS (P<0.05). OD: optical density. a), b), or c)There is a statistically significant difference; a) vs. b), a) vs. c), and b) vs. c) (P<0.05).
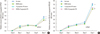
Figure 3
Effects of Porphyromonas gingivalis lipopolysaccharide (LPS) and/or (-)-epigallocatechin-3-gallate (EGCG) on inflammatory mRNA expressions. EGCG-treated cells (human periodontal ligament fibroblasts [hPDLFs] and human periodontal ligament stem cells [hPDLSCs]) showed similar or lower gene expressions than those cells of 'No treat'. The gene expressions of 'P. gingivalis LPS' alone were highly increased at 6 hours, but those increased gene expressions were down-regulated in 'EGCG+P. ginvialis LPS' group by the additional treatment of EGCG. IL-1β: interleukin 1β, IL-6: interleukin 6, TNF-β: tumor necrosis factor-α.
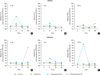
Figure 4
Effects of Porphyromonas gingivalis lipopolysaccharide (LPS) and (-)-epigallocatechin-3-gallate (EGCG) on osteoclastogenesis-related mRNA expression. (A, B, and C) The gene expression of 'P. gingivalis LPS alone' in human periodontal ligament fibroblasts (hPDLFs) was increased at 6 hours in receptor activator of nuclear factor kappa-B ligand (RANKL) and RANKL/osteoprotegerin (OPG), but it was down-regulated in 'EGCG+P. gingivalis LPS' group. (D, E, and F) The 'P. gingivali LPS alone' of human periodontal ligament stem cells (hPDLSCs) showed much higher gene expressions at 6 hours in both of RANKL and OPG, but those up-regulated gene expressions of RANKL and OPG were not increased in 'EGCG+P. gingivalis LPS' group.
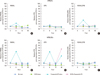
ACKNOWLEDGEMENTS
This study was supported by a grant of the Korea Healthcare Technology R&D Project, Ministry for Health, Welfare & Family Affairs, Republic of Korea (A101578).
References
1. Yamamoto T, Kita M, Oseko F, Nakamura T, Imanishi J, Kanamura N. Cytokine production in human periodontal ligament cells stimulated with Porphyromonas gingivalis. J Periodontal Res. 2006. 41:554–559.


2. Seo BM, Miura M, Gronthos S, Bartold PM, Batouli S, Brahim J, et al. Investigation of multipotent postnatal stem cells from human periodontal ligament. Lancet. 2004. 364:149–155.


3. Slots J, Bragd L, Wikstrom M, Dahlen G. The occurrence of Actinobacillus actinomycetemcomitans, Bacteroides gingivalis and Bacteroides intermedius in destructive periodontal disease in adults. J Clin Periodontol. 1986. 13:570–577.


4. Birkedal-Hansen H. Role of cytokines and inflammatory mediators in tissue destruction. J Periodontal Res. 1993. 28:500–510.


5. Nair SP, Meghji S, Wilson M, Reddi K, White P, Henderson B. Bacterially induced bone destruction: mechanisms and misconceptions. Infect Immun. 1996. 64:2371–2380.


6. Aznar C, Fitting C, Cavaillon JM. Lipopolysaccharide-induced production of cytokines by bone marrow-derived macrophages: dissociation between intracellular interleukin 1 production and interleukin 1 release. Cytokine. 1990. 2:259–265.


7. Boyce BF, Aufdemorte TB, Garrett IR, Yates AJ, Mundy GR. Effects of interleukin-1 on bone turnover in normal mice. Endocrinology. 1989. 125:1142–1150.


8. Gowen M, Wood DD, Ihrie EJ, McGuire MK, Russell RG. An interleukin 1 like factor stimulates bone resorption in vitro. Nature. 1983. 306:378–380.


9. Belibasakis GN, Bostanci N, Hashim A, Johansson A, Aduse-Opoku J, Curtis MA, et al. Regulation of RANKL and OPG gene expression in human gingival fibroblasts and periodontal ligament cells by Porphyromonas gingivalis: a putative role of the Arg-gingipains. Microb Pathog. 2007. 43:46–53.


10. Yun JH, Pang EK, Kim CS, Yoo YJ, Cho KS, Chai JK, et al. Inhibitory effects of green tea polyphenol (-)-epigallocatechin gallate on the expression of matrix metalloproteinase-9 and on the formation of osteoclasts. J Periodontal Res. 2004. 39:300–307.


11. Rogers J, Perkins I, van Olphen A, Burdash N, Klein TW, Friedman H. Epigallocatechin gallate modulates cytokine production by bone marrow-derived dendritic cells stimulated with lipopolysaccharide or muramyldipeptide, or infected with Legionella pneumophila. Exp Biol Med (Maywood). 2005. 230:645–651.


12. Yun JH, Kim CS, Cho KS, Chai JK, Kim CK, Choi SH. (-)-Epigallocatechin gallate induces apoptosis, via caspase activation, in osteoclasts differentiated from RAW 264.7 cells. J Periodontal Res. 2007. 42:212–218.


13. Lee YL, Hong CY, Kok SH, Hou KL, Lin YT, Chen MH, et al. An extract of green tea, epigallocatechin-3-gallate, reduces periapical lesions by inhibiting cysteine-rich 61 expression in osteoblasts. J Endod. 2009. 35:206–211.


14. Cabrera C, Artacho R, Gimenez R. Beneficial effects of green tea: a review. J Am Coll Nutr. 2006. 25:79–99.
15. Crespy V, Williamson G. A review of the health effects of green tea catechins in in vivo animal models. J Nutr. 2004. 134:3431S–3440S.


16. Higdon JV, Frei B. Tea catechins and polyphenols: health effects, metabolism, and antioxidant functions. Crit Rev Food Sci Nutr. 2003. 43:89–143.


17. Jung IH, Yun JH, Cho AR, Kim CS, Chung WG, Choi SH. Effect of (-)-epigallocatechin-3-gallate on maintaining the periodontal ligament cell viability of avulsed teeth: a preliminary study. J Periodontal Implant Sci. 2011. 41:10–16.


18. Sakanaka S, Aizawa M, Kim M, Yamamoto T. Inhibitory effects of green tea polyphenols on growth and cellular adherence of an oral bacterium, Porphyromonas gingivalis. Biosci Biotechnol Biochem. 1996. 60:745–749.


19. Hosokawa Y, Hosokawa I, Ozaki K, Nakanishi T, Nakae H, Matsuo T. Catechins inhibit CCL20 production in IL-17A-stimulated human gingival fibroblasts. Cell Physiol Biochem. 2009. 24:391–396.


20. Morrison DC, Leive L. Fractions of lipopolysaccharide from Escherichia coli O111:B4 prepared by two extraction procedures. J Biol Chem. 1975. 250:2911–2919.


21. Apicella MA. Isolation and characterization of lipopolysaccharides. Methods Mol Biol. 2008. 431:3–13.


22. Okada H, Murakami S. Cytokine expression in periodontal health and disease. Crit Rev Oral Biol Med. 1998. 9:248–266.


23. Wada N, Maeda H, Yoshimine Y, Akamine A. Lipopolysaccharide stimulates expression of osteoprotegerin and receptor activator of NF-kappa B ligand in periodontal ligament fibroblasts through the induction of interleukin-1 beta and tumor necrosis factor-alpha. Bone. 2004. 35:629–635.


24. Hirasawa M, Takada K, Makimura M, Otake S. Improvement of periodontal status by green tea catechin using a local delivery system: a clinical pilot study. J Periodontal Res. 2002. 37:433–438.


25. Ahmed S, Wang N, Lalonde M, Goldberg VM, Haqqi TM. Green tea polyphenol epigallocatechin-3-gallate (EGCG) differentially inhibits interleukin-1 beta-induced expression of matrix metalloproteinase-1 and -13 in human chondrocytes. J Pharmacol Exp Ther. 2004. 308:767–773.


26. Yang F, Oz HS, Barve S, de Villiers WJ, McClain CJ, Varilek GW. The green tea polyphenol (-)-epigallocatechin-3-gallate blocks nuclear factor-kappa B activation by inhibiting I kappa B kinase activity in the intestinal epithelial cell line IEC-6. Mol Pharmacol. 2001. 60:528–533.
27. Liao S, Kao YH, Hiipakka RA. Green tea: biochemical and biological basis for health benefits. Vitam Horm. 2001. 62:1–94.


28. Bae JY, Kanamune J, Han DW, Matsumura K, Hyon SH. Reversible regulation of cell cycle-related genes by epigallocatechin gallate for hibernation of neonatal human tarsal fibroblasts. Cell Transplant. 2009. 18:459–469.


29. Han DW, Matsumura K, Kim B, Hyon SH. Time-dependent intracellular trafficking of FITC-conjugated epigallocatechin-3-O-gallate in L-929 cells. Bioorg Med Chem. 2008. 16:9652–9659.


30. Tang SN, Fu J, Shankar S, Srivastava RK. EGCG enhances the therapeutic potential of gemcitabine and CP690550 by inhibiting STAT3 signaling pathway in human pancreatic cancer. PLoS One. 2012. 7:e31067.


31. Pathirana RD, O'Brien-Simpson NM, Reynolds EC. Host immune responses to Porphyromonas gingivalis antigens. Periodontol 2000. 2010. 52:218–237.


32. Graves DT, Cochran D. The contribution of interleukin-1 and tumor necrosis factor to periodontal tissue destruction. J Periodontol. 2003. 74:391–401.


33. Ishimi Y, Miyaura C, Jin CH, Akatsu T, Abe E, Nakamura Y, et al. IL-6 is produced by osteoblasts and induces bone resorption. J Immunol. 1990. 145:3297–3303.
34. Hasegawa T, Yoshimura Y, Kikuiri T, Yawaka Y, Takeyama S, Matsumoto A, et al. Expression of receptor activator of NF-kappa B ligand and osteoprotegerin in culture of human periodontal ligament cells. J Periodontal Res. 2002. 37:405–411.


35. Pfeilschifter J, Chenu C, Bird A, Mundy GR, Roodman GD. Interleukin-1 and tumor necrosis factor stimulate the formation of human osteoclastlike cells in vitro. J Bone Miner Res. 1989. 4:113–118.


36. Perez-Sayans M, Somoza-Martin JM, Barros-Angueira F, Rey JM, Garcia-Garcia A. RANK/RANKL/OPG role in distraction osteogenesis. Oral Surg Oral Med Oral Pathol Oral Radiol Endod. 2010. 109:679–686.


37. Imatani T, Kato T, Okuda K. Production of inflammatory cytokines by human gingival fibroblasts stimulated by cell-surface preparations of Porphyromonas gingivalis. Oral Microbiol Immunol. 2001. 16:65–72.


38. Kim HS, Kim KH, Kim SH, Kim YS, Koo KT, Kim TI, et al. Immunomodulatory effect of canine periodontal ligament stem cells on allogenic and xenogenic peripheral blood mononuclear cells. J Periodontal Implant Sci. 2010. 40:265–270.

