Abstract
Purpose
Nitric oxide (NO) has been known as an important regulator of osteoblasts and periodontal ligament cell activity. This study was performed to investigate the relationship between NO-mediated cell death of human periodontal ligament fibroblasts (PDLFs) and N-methyl-D-aspartic acid (NMDA) receptor antagonist (+)-5-methyl-10, 11-dihydro-5H-dibenzo[a,d]cyclohepten-5, 10-imine hydrogen maleate (MK801).
Methods
Human PDLFs were treated with various concentrations (0 to 4 mM) of sodium nitroprusside (SNP) with or without 200 µM MK801 in culture media for 16 hours and the cell medium was then removed and replaced by fresh medium containing MTS reagent for cell proliferation assay. Western blot analysis was performed to investigate the effects of SNP on the expression of Bax, cytochrome c, and caspase-3 proteins. The differences for each value among the sample groups were compared using analysis of variance with 95% confidence intervals.
Results
In the case of SNP treatment, as a NO donor, cell viability was significantly decreased in a concentration-dependent manner. In addition, a synergistic effect was shown when both SNP and NMDA receptor antagonist was added to the medium. SNP treated PDLFs exhibited a round shape in culture conditions and were dramatically reduced in cell number. SNP treatment also increased levels of apoptotic marker protein, such as Bax and cytochrome c, and reduced caspase-3 in PDLFs. Mitogen-activated protein kinase signaling was activated by treatment of SNP and NMDA receptor antagonist.
Nitric oxide (NO), a free radical synthesized from L-arginine by NO synthases (NOS), can modulate various tissue and cell activities, including vasodilation, neurotransmission, immune responses, and death control [1,2]. NO is also involved in the regulation of bone metabolism through the several biological process [3]. Bone forming osteoblasts constitutively produce a small amount of NO which regulates proliferation and differentiation of osteoblasts [4,5]. Overproduction of NO, which is stimulated by inflammatory cytokines or mechanical stress of osteoblasts, may lead to cell damage and initiate and regulate apoptosis [4,6,7]. In inflammation-induced osteoporosis, elevated levels of NO have been shown to cause apoptosis and to increase bone-resorption by osteoclasts, resulting in decreased bone mineral density [8].
In the central nervous system, NO production is closely associated with N-methyl-D-aspartic acid (NMDA) receptors [9]. NMDA receptors, as glutamate receptors residing in presynaptic and postsynaptic neurons, have been known to control synaptic plasticity and memory formation in the central nervous system [10]. Upon stimulation of NMDA receptors by an external signal, Ca2+ enters the cell through the activated ionotropic NMDA receptor and then NO is produced by NOS which translocates to the membrane and is activated by Ca2+ [11]. An increase in NO induces excitotoxic cell death or apoptotic events in afflicted neurons.
Previous studies have revealed that glutamate NMDA receptors in osteoblasts may be involved in regulation of bone formation [12,13]. Recently, we have also reported that NMDA receptors are involved in periodontal ligament fibroblast (PDLF) differentiation but not their proliferation [14]. PDL cells form the connective tissue between the tooth cementum and alveolar bone to anchor teeth and maintain structural integrity. In addition, PDLFs have the potential to differentiate into cementoblasts and osteoblasts needed for cementum and alveolar bone formation, respectively [15-17].
Mechanical stress has been shown to promote an increase in NO production in cultured human PDL cells [18]. Constitutive NO production arising from chewing or other mechanical-stress of teeth may play several roles in periodontal tissues, such as serving to maintain structural integrity of PDL [19] and regulating PDL response to various stimuli like proinflammatory cytokines, mechanical strain, and sex hormones [6,20,21]. However, excessively produced NO may lead to cell damage in periodontal tissues similar to that in endothelial cells and osteoblasts [22,23]. For example, lipoplysaccharide (LPS)-induced nitrite synthesis in PDLFs and osteoblasts led to cell apoptosis and increased pro-apoptotic responses to cytokines [24,25]. This has been shown in the cell types such as human monocytes, endothelial cells, macrophages, and gingival fibroblasts, which produce inflammatory cytokines via stimuli of bacteria or bacterial components like LPS [26].
However, the function of NMDA receptor in NO-mediated PDLF cell damage has not been well characterized. We investigated the relationship between the effect of excessively produced NO using sodium nitroprusside (SNP) and NMDA receptor antagonist.
(+)-5-methyl-10, 11-dihydro-5H-dibenzo[a,d]cyclohepten-5, 10-imine hydrogen maleate (MK801) and SNP were purchased from Sigma Aldrich (St. Louis, MO, USA). 3-(4,5-dimethylthiazol-2-yl)-5-(3-carboxymethoxyphenyl)-2-(4-sulfophenyl)-2H-tetrazolium, inner salt (MTS) was purchased from Promega (Madison, WI, USA ). Cell culture reagents were obtained from Hyclone (Logan, UT, USA) and Gibco (Paisley, Scotland). Protease inhibitor cocktail was purchased from Roche (Rotkreuz, Switzerland). Primary antibodies of caspase-3, Bax, cytochrome c, c-Jun N-terminal kinase/stress-activated protein kinase (JNK/SARK), mitogen-activated protein kinases (p38) and anti-rabbit, anti-mouse second antibodies were obtained from Santa Cruz Biotechnology (Santa Cruz, CA, USA). Anti-phosphorylated extracellular signal-regulated kinases (pERK), ERK rabbit polyclonal antibodies were prepared by Cell Signaling Technology (Beverly, MA, USA). β-actin mouse monoclonal antibody was purchased from Sigma Aldrich.
Human PDLFs were purchased from ScienCell (San Diego, CA, USA). They were cultured in α-MEM supplemented with 10% fetal bovine serum, 100 U/mL penicillin and 100 µg/mL streptomycin at 37℃ in a 5% CO2 humidified atmosphere. SNP was dissolved in phosphate buffered saline (PBS) buffer to 1 M as stock solution, stored frozen at -20℃ and protected from light for use in related experiments. PDLFs were treated with various concentrations (0, 0.5, 1, 1.5, 2, 2.5, 3, 3.5, and 4 mM) of SNP with or without 200 µM MK801 in culture media for 16 hours and PDLF morphologies were observed and photographed. All of the media were changed every 3 days.
After the cells were treated with SNP at the various concentrations for 16 hours, the cell medium was removed and then replaced by fresh medium containing MTS reagent. The cells were incubated at 37℃ in a 5% CO2 for 3 hours, and then the absorbance at 490 nm was measured on a microplate reader (Molecular Devices, Sunnyvale, CA, USA).
Human PDLFs were washed with PBS and collected in radioimmunoprecipitation assay buffer (150 mM sodium chloride, 1% Triton X-100, 1% sodium deoxycholate, 0.1% sodium dodecyl sulfate [SDS], 50 mM Tris-HCl, pH 7.5, and 2 mM ethylenediaminetetraacetic acid) with protease inhibitor cocktail (Roche, Rotkreuz, Switzerland). Equal amounts of proteins were separated by SDS-polyacrylamide gel electrophoresis and transferred to polyvinylidine fluoride membrane. The membrane was incubated with blocking buffer containing 5% skim milk in tris buffered saline for 2 hours. After blocking, the membranes were incubated for 2 hours with primary antibody, 1:1,000 anti-pERK, anti-ERK, anti-caspase-3 rabbit polyclonal antibodies, and anti-Bax, anti-cytochrome c, anti-β-actin mouse monoclonal antibodies, followed by incubation for 1 hour with 1:5,000 anti-rabbit and anti-mouse secondary antibodies, respectively. The membrane was detected with the aid of electrochemiluminescence detection reagents (Takara, Kyoto, Japan) using a fluorescence imaging system (Fuji Film, Tokyo, Japan).
A statistical software package (SPSS Inc., Chicago, IL, USA) was used to evaluate the data on cell proliferation. The differences for each value among the sample groups were compared using analysis of variance. The statistical significance of the results was analyzed using 95% confidence intervals.
To determine the effect of SNP on the PDLFs, we first observed morphological changes. PDLF morphologies were observed and photographed following treatments of 0 to 4 mM SNP with or without 200 µM MK801, the NMDA receptor antagonist, for 16 hours. There was alteration of PDLF cell morphology in a SNP concentration-dependent manner. The 3 mM SNP treated PDLFs led to a dramatic reduction in the numbers of flat, spread, and spindle-shaped fibroblasts and loss of cell-cell contact, and increased cellular debris from apparently dead/dying cells (Fig. 1A). The PDLF morphologies were largely unaltered when they were cultured with 0 to 2 mM SNP. Interestingly, the PDLF morphology and viability were more sensitive to SNP in the presence of MK801, the blocker of NMDA receptors; the rounded and floating cells, and large loss of flat and spread PDLFs were observed at 2 mM SNP (Fig. 1A). We determined cell viability by MTS assay after SNP and MK801 exposure. Exposure of PDLFs to 0 and 0.5 mM SNP for 16 hours did not affect cell viability. In the 2.5 mM SNP treated PDLFs, significantly decreased optical density (A490) was determined by MTS assay, and in the presence of MK801, 1 mM SNP treated PDLFs showed similarly significant reduction compared to the untreated control (Fig. 1B), suggesting that PDLF viability is sensitive to NO and MK801.
Fig. 2 shows the effects of SNP on Bax, cytochrome c, and caspase-3 proteins. Administration of SNP in PDLFs increased Bax and cytochrome c production. However caspase-3 protein was decreased. Bax protein production was increased in 2 mM SNP treated PDLFs, and was highly expressed at 1 mM SNP treated cells in the presence of MK801. Cytochrome c protein represented the high expression in 2 mM SNP treated PDLFs. In MK801 treated PDLFs showed that cytochrome c was highly expressed at 1 mM SNP treatment. Caspase-3 protein was decreased in 3 mM SNP treated PDLFs and 2 mM SNP treated PDLFs in the presence of MK801. These results suggest that PDLFs reacted more sensitively in the presence of MK801 compared to the group treated with SNP
alone.
SNP treated PDLFs increased the phosphorylation of mitogen-activated protein kinase (MAPK) pathway proteins such as JNK/SARK, ERK, and p38 (Fig. 3). Western blot results revealed that phosphorylation of JNK/SARK and ERK proteins were induced from 3 mM SNP treated PDLFs. In the presence of MK801, increased phosphorylation of these proteins was shown in 2 mM SNP treated PDLFs. Phosphorylation of p38 was detected in 4 mM SNP treated PDLFs and revealed with 3 mM SNP in the presence of MK801. Notably, pERK was significantly increased in the presence of SNP and MK801.
Many of the pathogenic effects of bacteria in periodontitis involving alveolar bone destruction are mediated by bacterial products including endotoxin or LPS [27,28]. LPS can directly induce cell death or apoptosis in many cell types, and by stimulating NO production in macrophages, epithelial cells, and fibroblasts [29-31]. The production of high levels of NO in various cells is associated with autocytotoxicity, suppression of carcinogenesis, and inhibition of metastasis that result partially from induction of apoptosis [32].
In central neurons, NO is produced by elevated cytosolic Ca2+ which constitutively activates neuronal NOS (nNOS) via the activation of glutamate NMDA receptors [9]. There are two NOS subtypes: inducible NOS (iNOS) found in fibroblasts and macrophages and endothelial NOS (eNOS) in blood vessel walls [33]. PDL cells express both eNOS and iNOS, and NO products by eNOS, and iNOS modulates the function of PDL cells [19] and by occlusal stimuli in the rat PDL promotes PDL healing in transplanted teeth [34]. In this study we focused on the relation of NO and NMDA receptors in PDLFs.
NO released by SNP, an extracellular NO donor, has similar cytotoxic effects on PDLFs to induction of intracellular NO which is produced by NOS. A high level of NO induces oxidative stress in cells that may lead to apoptosis accompanied by changes in gene expression, and cell dysfunction and damage. As shown in Fig. 1, SNP treated PDLFs are associated with cell viability as evidenced by changes in their morphology into shrunken and round from spindle-shaped, and by the decrease in cell number and reduction in response to MTS assay. When PDLFs were treated with NMDA receptor antagonist, cell death of PDLFs was promoted at a lower concentration than only administration of SNP. In addition, our results showed that the oxidative stress by SNP-induced NO increased expression of apoptotic marker proteins such as Bax and cytochrome c. Interestingly, MK801 treatment of SNP stimulated PDLFs further enhanced the apoptotic responses of these cells in morphology and apoptosis marker protein expression than SNP treatment alone. Our results suggest that SNP, as a nitric donor, promotes apoptotic protein-induced PDLF cell death.
De novo synthesis of Bax protein by SNP has been shown to be proapoptotic in NO-induced osteoblast death [35], suggesting that increased Bax protein levels may be a critical trigger of apoptosis induced by oxidative stress in osteoblasts [36]. Cytochrome c is another well-known marker protein of apoptosis that may be released from mitochondria to the cytoplasm in osteoblasts under oxidative stress and associated depolarization of the mitochondrial membrane potential [37]. Decreased levels of caspase-3 expression in SNP treated PDLFs are also consistent with conversion and activation from the inactive form of caspase-3, which is specifically labeled as anti-caspase-3 antibody in the immunoblot analysis shown in Fig. 2. Activation of caspase-3 can cause the digestion of key cellular proteins and induce DNA fragmentation and cell apoptosis [38,39]. Possibly, similar proapoptotic alterations in Bax levels, cytochrome c-mediated activation of caspase-3 activity, and DNA fragmentation may have occurred in PDLFs treated with SNP in our study.
In most cells, NO-mediated apoptosis typically involves activation by phosphorylation of the JNK/SARK, ERK, and p38 groups of MAPKs [40]. We monitored the phosphorylation of ERK, p38, and JNK following treatment with SNP and SNP/MK801 to determine whether these kinases were involved in the signaling pathway of NO-mediated apoptosis in PDLFs. In NO induced PDLFs, phosphorylation of JNK/SARK, ERK and p38 was increased compared to the control PDLFs. In PDLFs treated with SNP and MK801, the phosphorylation of the MAPK panel occurred at lower concentrations of SNP that also produced morphologic and biochemical differences as shown in Fig. 1. Overall, these results indicate that in PDLFs, NO overproduction may induce an apoptosis signal via the MAPK pathway, and suggest that activated glutamate NMDA receptors in PDLFs may inhibit cell death and apoptosis, which are induced by conditions that stimulate excessive NO production, such as periodontal bacterial infections.
Figures and Tables
Figure 1
Effect of sodium nitroprusside (SNP) on the viability of periodontal ligament fibroblasts (PDLFs). PDLFs showed morphological changes following treatment with SNP and SNP+200 µM (+)-5-methyl-10, 11-dihydro-5H-dibenzo[a,d]cyclohepten-5, 10-imine hydrogen maleate (MK801) for 16 hours (A). PDLFs were treated with SNP at indicated concentrations for 16 hours and the cell viability was determined by MTS assay (B). Magnification ×100. a)Indicates significantly different from non-treated cells (P<0.05). OD: optical density.
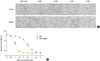
Figure 2
Sodium nitroprusside (SNP) altered the expression of apoptosis marker proteins. Periodontal ligament fibroblasts were treated with SNP and (+)-5-methyl-10, 11-dihydro-5H-dibenzo[a,d] cyclohepten-5, 10-imine hydrogen maleate (MK801) for 16 hours. The cell lysates were performed to Western blot analysis using anti-Bax, anti-cytochrome c, anti-caspase-3, and anti-β-actin antibodies. β-actin was used as internal control.
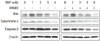
Figure 3
Sodium nitroprusside (SNP) induced phosphorylation of mitogen-activated protein kinases in periodontal ligament fibroblasts (PDLFs). PDLFs were treated with SNP and (+)-5-methyl-10, 11-dihydro-5H-dibenzo[a,d]cyclohepten-5, 10-imine hydrogen maleate (MK801) for 16 hours. The cell lysates were performed to Western blot analysis using anti-c-Jun N-terminal kinase/stress-activated protein kinase (JNK/SARK), anti-extracellular-signal-regulated kinase (ERK), anti-mitogen-activated protein kinases (p38), anti-phosphorylated JNK/SARK, anti-phosphorylated ERK or anti-phosphorylated p38 antibodies.
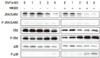
ACKNOWLEDGMENTS
This work was supported by a Korea Research Foundation (KRF) grant funded by the Korean government (MEST) (No. 2009-0077792) and a grant of the Korean Health Technology R&D Project (A101768), Ministry for Health, Welfare & Family Affairs, Republic of Korea.
References
1. Chang H, Tsai SY, Chang Y, Chen TL, Chen RM. Therapeutic concentrations of propofol protects mouse macrophages from nitric oxide-induced cell death and apoptosis. Can J Anaesth. 2002; 49:477–480.


2. Moncada S, Palmer RM, Higgs EA. Nitric oxide: physiology, pathophysiology, and pharmacology. Pharmacol Rev. 1991; 43:109–142.
3. van't Hof RJ, Ralston SH. Nitric oxide and bone. Immunology. 2001; 103:255–261.
4. Mancini L, Moradi-Bidhendi N, Becherini L, Martineti V, MacIntyre I. The biphasic effects of nitric oxide in primary rat osteoblasts are cGMP dependent. Biochem Biophys Res Commun. 2000; 274:477–481.


5. Koyama A, Otsuka E, Inoue A, Hirose S, Hagiwara H. Nitric oxide accelerates the ascorbic acid-induced osteoblastic differentiation of mouse stromal ST2 cells by stimulating the production of prostaglandin E(2). Eur J Pharmacol. 2000; 391:225–231.


6. Ralston SH, Todd D, Helfrich M, Benjamin N, Grabowski PS. Human osteoblast-like cells produce nitric oxide and express inducible nitric oxide synthase. Endocrinology. 1994; 135:330–336.


7. Damoulis PD, Hauschka PV. Cytokines induce nitric oxide production in mouse osteoblasts. Biochem Biophys Res Commun. 1994; 201:924–931.


8. Armour KE, Van'T Hof RJ, Grabowski PS, Reid DM, Ralston SH. Evidence for a pathogenic role of nitric oxide in inflammation-induced osteoporosis. J Bone Miner Res. 1999; 14:2137–2142.


9. Brunetti L. Nitric oxide: a gas as a modulator of neuroendocrine secretions. Clin Ter. 1994; 144:147–153.
10. Contestabile A. Roles of NMDA receptor activity and nitric oxide production in brain development. Brain Res Brain Res Rev. 2000; 32:476–509.


11. Arundine M, Sanelli T, Ping He B, Strong MJ. NMDA induces NOS 1 translocation to the cell membrane in NGF-differentiated PC 12 cells. Brain Res. 2003; 976:149–158.


12. Hinoi E, Fujimori S, Yoneda Y. Modulation of cellular differentiation by N-methyl-D-aspartate receptors in osteoblasts. FASEB J. 2003; 17:1532–1534.
13. Taylor AF. Osteoblastic glutamate receptor function regulates bone formation and resorption. J Musculoskelet Neuronal Interact. 2002; 2:285–290.
14. Yu JH, Lee SP, Kim TI, Jang JH. Identification of N-methyl-D-aspartate receptor subunit in human periodontal ligament fibroblasts: potential role in regulating differentiation. J Periodontol. 2009; 80:338–346.


15. Tomokiyo A, Maeda H, Fujii S, Wada N, Shima K, Akamine A. Development of a multipotent clonal human periodontal ligament cell line. Differentiation. 2008; 76:337–347.


16. Basdra EK, Komposch G. Osteoblast-like properties of human periodontal ligament cells: an in vitro analysis. Eur J Orthod. 1997; 19:615–621.


17. Seo BM, Miura M, Gronthos S, Bartold PM, Batouli S, Brahim J, et al. Investigation of multipotent postnatal stem cells from human periodontal ligament. Lancet. 2004; 364:149–155.


18. Kikuiri T, Hasegawa T, Yoshimura Y, Shirakawa T, Oguchi H. Cyclic tension force activates nitric oxide production in cultured human periodontal ligament cells. J Periodontol. 2000; 71:533–539.


19. Watarai H, Warita H, Soma K. Effect of nitric oxide on the recovery of the hypofunctional periodontal ligament. J Dent Res. 2004; 83:338–342.


20. Armour KE, Ralston SH. Estrogen upregulates endothelial constitutive nitric oxide synthase expression in human osteoblast-like cells. Endocrinology. 1998; 139:799–802.


21. Klein-Nulend J, Helfrich MH, Sterck JG, MacPherson H, Joldersma M, Ralston SH, et al. Nitric oxide response to shear stress by human bone cell cultures is endothelial nitric oxide synthase dependent. Biochem Biophys Res Commun. 1998; 250:108–114.


22. Chen RM, Chen TL, Chiu WT, Chang CC. Molecular mechanism of nitric oxide-induced osteoblast apoptosis. J Orthop Res. 2005; 23:462–468.


23. Palmer RM, Bridge L, Foxwell NA, Moncada S. The role of nitric oxide in endothelial cell damage and its inhibition by glucocorticoids. Br J Pharmacol. 1992; 105:11–12.


24. Lin SK, Kok SH, Lin LD, Wang CC, Kuo MY, Lin CT, et al. Nitric oxide promotes the progression of periapical lesion via inducing macrophage and osteoblast apoptosis. Oral Microbiol Immunol. 2007; 22:24–29.


25. Thammasitboon K, Goldring SR, Boch JA. Role of macrophages in LPS-induced osteoblast and PDL cell apoptosis. Bone. 2006; 38:845–852.


26. Alexander MB, Damoulis PD. The role of cytokines in the pathogenesis of periodontal disease. Curr Opin Periodontol. 1994; 39–53.
27. Rogers JE, Li F, Coatney DD, Rossa C, Bronson P, Krieder JM, et al. Actinobacillus actinomycetemcomitans lipopolysaccharide-mediated experimental bone loss model for aggressive periodontitis. J Periodontol. 2007; 78:550–558.


28. Reddi K, Meghji S, Wilson M, Henderson B. Comparison of the osteolytic activity of surface-associated proteins of bacteria implicated in periodontal disease. Oral Dis. 1995; 1:26–31.


29. Sosroseno W, Bird PS, Seymour GJ. Nitric oxide production by a human osteoblast cell line stimulated with Aggregatibacter actinomycetemcomitans lipopolysaccharide. Oral Microbiol Immunol. 2009; 24:50–55.


30. Albina JE, Cui S, Mateo RB, Reichner JS. Nitric oxide-mediated apoptosis in murine peritoneal macrophages. J Immunol. 1993; 150:5080–5085.
31. Tiwari MM, Messer KJ, Mayeux PR. Inducible nitric oxide synthase and apoptosis in murine proximal tubule epithelial cells. Toxicol Sci. 2006; 91:493–500.


32. Xie K, Huang S, Dong Z, Juang SH, Wang Y, Fidler IJ. Destruction of bystander cells by tumor cells transfected with inducible nitric oxide (NO) synthase gene. J Natl Cancer Inst. 1997; 89:421–427.


34. Chen CC, Kanno Z, Soma K. Occlusal forces promote periodontal healing of transplanted teeth with enhanced nitric oxide synthesis. J Med Dent Sci. 2005; 52:59–64.
35. Chen RM, Liu HC, Lin YL, Jean WC, Chen JS, Wang JH. Nitric oxide induces osteoblast apoptosis through the de novo synthesis of Bax protein. J Orthop Res. 2002; 20:295–302.


36. Srivastava RK, Sollott SJ, Khan L, Hansford R, Lakatta EG, Longo DL. Bcl-2 and Bcl-X(L) block thapsigargin-induced nitric oxide generation, c-Jun NH(2)-terminal kinase activity, and apoptosis. Mol Cell Biol. 1999; 19:5659–5674.


37. Ho WP, Chen TL, Chiu WT, Tai YT, Chen RM. Nitric oxide induces osteoblast apoptosis through a mitochondria-dependent pathway. Ann N Y Acad Sci. 2005; 1042:460–470.


38. Earnshaw WC, Martins LM, Kaufmann SH. Mammalian caspases: structure, activation, substrates, and functions during apoptosis. Annu Rev Biochem. 1999; 68:383–424.

