Abstract
Purpose
Curcumin is known to exert numerous biological effects including anti-inflammatory activity. In this study, we investigated the effects of curcumin on the production of interleukin-6 (IL-6) by murine macrophage-like RAW 264.7 cells stimulated with lipopolysaccharide (LPS) from Prevotella intermedia, a major cause of inflammatory periodontal disease, and sought to determine the underlying mechanisms of action.
Methods
LPS was prepared from lyophilized P. intermedia ATCC 25611 cells by the standard hot phenol-water method. Culture supernatants were collected and assayed for IL-6. We used real-time polymerase chain reaction to detect IL-6 mRNA expression. IκB-α degradation, nuclear translocation of NF-κB subunits, and STAT1 phosphorylation were characterized via immunoblotting. DNA-binding of NF-κB was also analyzed.
Results
Curcumin strongly suppressed the production of IL-6 at both gene transcription and translation levels in P. intermedia LPS-activated RAW 264.7 cells. Curcumin did not inhibit the degradation of IκB-α induced by P. intermedia LPS. Curcumin blocked NF-κB signaling through the inhibition of nuclear translocation of NF-κB p50 subunit. Curcumin also attenuated DNA binding activity of p50 and p65 subunits and suppressed STAT1 phosphorylation.
Curcumin is a major phenolic compound found in the spice turmeric [1]. This compound has been shown to possess a wide spectrum of potential biological benefits, including antioxidant, anti-tumor, cardio-protective, antibacterial, antiviral, and anti-inflammatory properties [2-5]. Thus, curcumin has a potential therapeutic value against various chronic diseases, including allergies, rheumatoid arthritis, diabetes, cardiovascular diseases, cancer, and other chronic illnesses.
Periodontal disease is a chronic inflammatory process accompanied by destruction of surrounding connective tissue and alveolar bone, and sometimes loss of teeth [6]. The primary causative agents of periodontal disease are particular gram-negative anaerobic bacteria that accumulate in the gingival sulcus. Prevotella intermedia is a major periodontal pathogen that is dominant in the subgingival flora of patients with adult periodontitis [7,8], acute necrotizing ulcerative gingivitis [9], and pregnancy gingivitis [10].
Lipopolysaccharide (LPS) is a major component of the outer membrane of gram-negative bacteria, including P. intermedia. It can trigger a number of host cells to produce and release a wide variety of proinflammatory cytokines, including tumor necrosis factor alpha (TNF-α), IL-1β, IL-6, and IL-8 [11]. LPS preparations extracted from oral black-pigmented bacteria including P. intermedia have been reported to possess unique chemical and immunobiological properties quite different from those of the classical LPSs from the family Enterobacteriaceae such as Escherichia coli and Salmonella species [12-14].
Host responses to the specific causative bacteria and their metabolic products are a major determinant of disease pathogenesis. Recent work has demonstrated, in addition to bacterial control, that modulation of the host inflammatory response is a plausible therapeutic strategy for periodontal disease [15,16]. Therefore, host modulatory agents directed at inhibiting specific cytokines appear to be beneficial in terms of attenuating periodontal disease progression and potentially enhancing therapeutic responses. Therefore, in the present study we investigated the effects of curcumin on the production of IL-6 by macrophages stimulated with LPS from P. intermedia and sought to determine the underlying mechanisms of action.
Curcumin was obtained from Sigma-Aldrich (St. Louis, MO, USA). Antibodies against IκB-α, STAT1 and phospho-STAT1 were obtained from Cell Signaling Technology (Beverly, MA, USA), while antibodies against NF-κB p65, NF-κB p50, and PARP-1 were purchased from Santa Cruz Biotechnology (Santa Cruz, CA, USA).
P. intermedia ATCC 25611 was used throughout. It was grown anaerobically on the surface of enriched Trypticase soy agar containing 5% (v/v) sheep blood, or in general anaerobic medium broth (Nissui, Tokyo, Japan) supplemented with 1 µg/mL menadione and 5 µg/mL hemin. Culture purity was assessed by gram staining and plating on solid medium.
LPS was prepared from lyophilized P. intermedia ATCC 25611 cells by the standard hot phenol-water method as described previously [17]. The protein content of the purified LPS was less than 0.1%. Coomassie blue staining of overloaded sodium dodecyl sulfate (SDS)-polyacrylamide gels did not reveal any visible protein bands in the purified LPS, confirming the purity of the preparation (data not shown).
The murine macrophage cell line RAW 264.7 (American Type Culture Collection, Rockville, MD, USA) was in Dulbecco's modified Eagle's medium as described previously [17]. The cells were seeded into 24-well culture plates at a density of 5×105 cells/well and incubated for at least 12 hours to allow them to adhere to the plates. After washing three times with medium, various concentrations of P. intermedia LPS and curcumin were added and the cells were cultured for 24 hours, after which culture supernatants were collected and assayed for IL-6.
The cellular toxicity of curcumin was assessed by the MTT assay. Cells were incubated with various concentrations of P. intermedia LPS and curcumin for 24 hours, and MTT was added to the cultures to a final concentration of 0.5 mg/mL. After incubation at 37℃ in 5% CO2 for 2 hours, the supernatant was removed and the cells were solubilized in dimethyl sulfoxide. The extent of reduction of MTT to formazan within the cells was quantified by measuring absorbance at 570 nm with a Spectra Max 250 ELISA Reader (Molecular Devices Inc., Sunnyvale, CA, USA).
The amount of IL-6 secreted into the culture medium was determined by enzyme-linked immunosorbent assay (ELISA) using a commercially available kit (OptEIA, BD Biosciences Pharmingen, San Diego, CA, USA) according to protocols recommended by the manufacturer.
Cells were plated in 100 mm tissue culture dishes at a density of 1×107 cells/dish and treated with various concentrations of P. intermedia LPS and curcumin for 24 hours. Total RNA was isolated with an RNeasy Mini Kit (Qiagen, Valencia, CA, USA) according to the manufacturer's instructions. cDNA was prepared from 1 µg of the total RNA using iScript cDNA Synthesis Kit (Bio-Rad Laboratories Inc., Hercules, CA, USA). A real-time PCR was performed using the CFX96 real-time PCR detection system (Bio-Rad Laboratories Inc.) with a specific primer for mouse IL-6. As an endogenous control, β-actin primer was used. PCR was conducted with SsoFast EvaGreen Supermix (Bio-Rad Laboratories Inc.) according to the manufacturer's instruction. Thermal cycler conditions were as follows: After denaturing at 98℃ for 30 seconds, PCR was performed for 45 cycles, each of which consisted of denaturing at 95℃ for 1 second, annealing/extending at 60℃ for 5 seconds. The following PCR primers for IL-6 (162 bp), and β-actin (149 bp) were used: IL-6 sense, 5'-GCCAGAGTCCTTCAGAGAGATACAG-3' and antisense, 5'-GAATTGGATGGTCTTGGTC-CTTAGC-3'; β-actin sense, 5'-TGAGAGGGAAATCGTGCGTGAC-3' and antisense, 5'-GCTCGTTGCCAATAGTGATGACC-3'. Each assay was normalized to β-actin mRNA.
Cells were plated in 60-mm tissue culture dishes, at a density of 4×106 cells per dish, and treated with various concentrations of P. intermedia LPS and curcumin for the indicated periods of time. Cell lysates were prepared as described previously [17]. The nuclear fraction was prepared from cells using the ActiveMotif nuclear extract kit (Active Motif, Carlsbad, CA, USA) according to the manufacturer's instructions. Protein concentrations were determined with the bicinchoninic acid protein assay reagents (Pierce, Rockford, IL, USA) according to the manufacturer's instructions. The same amount of protein (30 µg) was then subjected to SDS-polyacrylamide gel electrophoresis on 10 to 12% acrylamide gels with 3% stacking gels. The resolved proteins were transferred to a nitrocellulose membrane by electroblotting, and the blots were blocked for 1 hour in PBST (Phosphate Buffered Saline with 0.1% Tween-20) containing 3% nonfat dry milk, followed by incubation with specific primary antibodies. They were then washed three times for 10 minutes each with PBST, incubated with horseradish peroxidase-conjugated secondary antibodies at room temperature for 1 hour and visualized by enhanced chemiluminescence (Cell Signaling Technology) as recommended.
Cells were plated in 60-mm tissue culture dishes, at a density of 4×106 cells per dish, and incubated with curcumin (20 µM) in the absence or presence of P. intermedia LPS (10 µg/mL) for the indicated periods of time. After extracting the nuclear protein as described above, the DNA-binding activity of NF-κB in nuclear extract was assayed by using a TransAM NF-κB p65/NF-κB p50 transcription factor assay kits (Active Motif) according to the manufacturer's recommended procedures. Oligonucleotide with the NF-κB consensus binding site (5'-GGGACTTTCC-3'), to which the activated NF-κB contained in nuclear extracts specifically binds, has been immobilized on a 96-strip well plate. The activated NF-κB p65 and p50 specifically bound to this oligonucleotide was detected using specific antibodies to NF-κB p65 and p50, respectively. A Spectra Max 250 ELISA Reader was used to read the sample absorbance, with results expressed as optical density emitted at 450 nm.
Stimulation of RAW 264.7 cells with P. intermedia LPS induced significant increases in IL-6 (Fig. 1). Curcumin inhibited IL-6 production dose-dependently in P. intermedia LPS-treated cells. Treatment of cells with 20 µM curcumin reduced IL-6 production by 83%. Curcumin did not affect the cell viability as determined by MTT assay (data not shown), indicating that inhibitory effects of curcumin on IL-6 production was unrelated to its cytotoxicity. As shown in Fig. 2, curcumin also reduced P. intermedia LPS-induced IL-6 mRNA expression in a dose-dependent manner.
The previous study [17] showed that the NF-κB and JAK2/STAT1 pathways are involved in IL-6 production induced by P. intermedia LPS. First, the effect of curcumin on P. intermedia LPS-induced degradation of IκB-α, upstream signaling pathway of NF-κB, was determined by immunoblotting. The degradation of IκB-α induced by LPS was not inhibited when cells were co-treated with curcumin (Fig. 3). Next, the effects of curcumin on the nuclear translocation of NF-κB subunits were examined. Nuclear fractions were immunoblotted with antibodies against NF-κB p65 and p50. As shown in Fig. 4A, curcumin did not affect P. intermedia LPS-induced p65 nuclear translocation. Instead, translocation of p50 subunit was dose-dependently hampered in the presence of curcumin (Fig. 4A). Finally, DNA-binding activity of NF-κB in nuclear extract was analyzed by using the ELISA-based NF-κB p65/NF-κB p50 transcription factor assay kits. DNA-binding activities of NF-κB p65 and p50 subunits induced by P. intermedia LPS were attenuated by treatment with curcumin (Fig. 4B).
It was examined whether curcumin regulates P. intermedia LPS-induced IL-6 production through inhibiting the STAT1 pathway. STAT1 phosphorylation induced by P. intermedia LPS was dose-dependently inhibited by curcumin (Fig. 5).
It is well known that LPSs from periodontal pathogens induce excess production of inflammatory mediators, such as NO, TNF-α, and IL-6 in immune cells [17-20]. These proinflammatory mediators are potential targets for the development of new therapeutic approaches to the treatment of periodontitis, because these molecules play a significant role in the development of periodontal destruction.
The results of the present study indicate that curcumin suppresses the production of IL-6 at both gene transcription and translation levels in P. intermedia LPS-activated RAW 264.7 cells. IL-6 is important in the pathogenesis of periodontal disease. Clinically, IL-6 levels in sites with periodontal disease are higher than those in healthy sites and closely related to the severity of periodontal disease [21,22]. Moreover, it has been well demonstrated that IL-6 is a potent bone resorptive agent, induces osteoclastogenesis, and hence plays important roles in alveolar bone resorption in periodontal disease [23,24]. Blockade of IL-6, therefore, could be a highly efficient tool for blocking the development and progression of inflammatory periodontal disease.
It is generally accepted that multiple signal transduction pathways participate in LPS-induced activation of macrophages and resultant production of proinflammatory cytokines, and NF-κB pathways play critical roles. NF-κB is a transcription factor that plays a critical role in the expressions of proinflammatory cytokines and other mediators [25-28]. In this study, curcumin did not inhibit NF-κB transcriptional activity at the level of IκB-α degradation. Curcumin blocked NF-κB signaling through inhibition of nuclear translocation of NF-κB p50 subunit induced with P. intermedia LPS. In addition, curcumin also attenuated DNA-binding activities of NF-κB p65 and p50 subunits. p50 usually forms a heterodimer with other NF-κB subunits and participates in target gene transcription [29,30]. Inhibition of NF-κB activity by curcumin would be useful in the treatment of inflammatory periodontal disease.
The STAT signaling pathway plays an essential role in the regulation of inflammatory responses [31]. The seven STAT family members are identified in mammals, and each one binds to a different DNA sequence [32]. The STAT family of transcription factors are activated by JAKs [33,34]. STAT1, downstream of JAK2, appears to be an important transcription factor for LPS-induced gene expressions in macrophages [35]. In this study, curcumin down-regulaed P. intermedia LPS-induced IL-6 production via regulating the STAT1 pathway. Thus, the STAT1 signaling pathway could be a potential molecular target for treating periodontal disease.
In summary, this study shows that curcumin strongly suppresses IL-6 production induced by LPS from P. intermedia in macrophages. The underlying mechanisms of curcumin involve the inhibition of NF-κB and STAT1 pathways. Although further study is required to explore the detailed mechanism of action, curcumin may contribute to blockade of the host-destructive processes mediated by IL-6 and appears to have potential therapeutic values in the treatment of inflammatory periodontal disease.
Figures and Tables
Figure 1
Effects of curcumin on Prevotella intermedia lipopolysaccharide (LPS)-induced production of interleukin (IL)-6 in RAW 264.7 cells. Cells were incubated with different doses of curcumin (0, 1, 5, 10, and 20 µM) in the absence or presence of P. intermedia LPS (10 µg/mL). Supernatants were removed after 24 hours and assayed for IL-6. The results are means±standard deviation of three independent experiments. a)P<0.01 versus P. intermedia LPS alone.
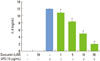
Figure 2
Effects of curcumin on Prevotella intermedia lipopolysaccharide (LPS)-induced interleukin (IL)-6 mRNA expression in RAW 264.7 cells. Cells were incubated with different doses of curcumin (0, 1, 5, 10, and 20 µM) in the absence or presence of P. intermedia LPS (10 µg/mL) for 24 hours. Real-time polymerase chain reaction was performed with EvaGreen Supermix, β-actin being used as an endogenous control. Data are presented as percentage of P. intermedia LPS alone. The results are means±standard deviation of three independent experiments. a)P<0.05. b)P<0.01 vs. P. intermedia LPS alone.
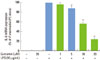
Figure 3
Effects of curcumin on Prevotella intermedia lipopolysaccharide (LPS)-induced IκB-α degradation in RAW 264.7 cells. Cells were incubated with different doses of curcumin (0, 1, 5, 10, and 20 µM) in the absence or presence of P. intermedia LPS (10 µg/mL). After 30 minutes of incubation, IκB-α degradation was determined by immunoblot analysis of cell lysates using antibody against IκB-α. A representative immunoblot from two separate experiments with similar results is shown.
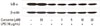
Figure 4
Effects of curcumin on nuclear translocation (A) and DNA binding activity (B) of NF-κB subunits induced with Prevotella intermedia lipopolysaccharide (LPS) in RAW 264.7 cells. (A) Cells were incubated with different doses of curcumin (0, 1, 5, 10, and 20 µM) in the absence or presence of P. intermedia LPS (10 µg/mL). After 30 minutes (for NF-κB p65) or 8 hours (for NF-κB p50) of incubation, the nuclear fraction was isolated from cells. Nuclear translocation of NF-κB subunits was assessed by immunoblot analysis using antibodies against NF-κB p65 and p50. A representative immunoblot from two separate experiments with similar results is shown. (B) Cells were incubated with different doses of curcumin (0 and 20 µM) in the absence or presence of P. intermedia LPS (10 µg/mL). After 30 minutes (for NF-κB p65) or 8 hours (for NF-κB p50) of incubation, DNA-binding activity of NF-κB in nuclear extracts was assessed by using the enzyme-linked immunosorbent assay-based NF-κB p65/NF-κB p50 transcription factor assay kits. The results are means±standard deviation of two independent experiments. a)P<0.05 vs. P. intermedia LPS alone. OD: optical density.
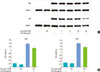
Figure 5
Effects of curcumin on Prevotella intermedia lipopolysaccharide (LPS)-induced phosphorylation of STAT1 in RAW 264.7 cells. Cells were incubated with different doses of curcumin (0, 1, 5, 10, and 20 µM) in the absence or presence of P. intermedia LPS (10 µg/mL) for 4 hours. Expression of phospho-STAT1 was measured by immunoblot analysis of cell lysates. Total-STAT1 was used as an internal control. A representative immunoblot from two separate experiments with similar results is shown.
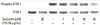
References
1. Surh Y. Molecular mechanisms of chemopreventive effects of selected dietary and medicinal phenolic substances. Mutat Res. 1999. 428:305–327.


2. Huang HC, Jan TR, Yeh SF. Inhibitory effect of curcumin, an anti-inflammatory agent, on vascular smooth muscle cell proliferation. Eur J Pharmacol. 1992. 221:381–384.


3. Joe B, Lokesh BR. Role of capsaicin, curcumin and dietary n-3 fatty acids in lowering the generation of reactive oxygen species in rat peritoneal macrophages. Biochim Biophys Acta. 1994. 1224:255–263.


4. Ruby AJ, Kuttan G, Babu KD, Rajasekharan KN, Kuttan R. Anti-tumour and antioxidant activity of natural curcuminoids. Cancer Lett. 1995. 94:79–83.


5. Rao CV, Rivenson A, Simi B, Reddy BS. Chemoprevention of colon carcinogenesis by dietary curcumin, a naturally occurring plant phenolic compound. Cancer Res. 1995. 55:259–266.
7. Slots J, Bragd L, Wikström M, Dahlén G. The occurrence of Actinobacillus actinomycetemcomitans, Bacteroides gingivalis and Bacteroides intermedius in destructive periodontal disease in adults. J Clin Periodontol. 1986. 13:570–577.


8. Tanner AC, Haffer C, Bratthall GT, Visconti RA, Socransky SS. A study of the bacteria associated with advancing periodontitis in man. J Clin Periodontol. 1979. 6:278–307.


9. Chung CP, Nisengard RJ, Slots J, Genco RJ. Bacterial IgG and IgM antibody titers in acute necrotizing ulcerative gingivitis. J Periodontol. 1983. 54:557–562.


10. Kornman KS, Loesche WJ. The subgingival microbial flora during pregnancy. J Periodontal Res. 1980. 15:111–122.


12. Hamada S, Takada H, Ogawa T, Fujiwara T, Mihara J. Lipopolysaccharides of oral anaerobes associated with chronic inflammation: chemical and immunomodulating properties. Int Rev Immunol. 1990. 6:247–261.


13. Kirikae T, Nitta T, Kirikae F, Suda Y, Kusumoto S, Qureshi N, et al. Lipopolysaccharides (LPS) of oral black-pigmented bacteria induce tumor necrosis factor production by LPS-refractory C3H/HeJ macrophages in a way different from that of Salmonella LPS. Infect Immun. 1999. 67:1736–1742.


14. Hashimoto M, Asai Y, Tamai R, Jinno T, Umatani K, Ogawa T. Chemical structure and immunobiological activity of lipid A from Prevotella intermedia ATCC 25611 lipopolysaccharide. FEBS Lett. 2003. 543:98–102.


16. Reddy MS, Geurs NC, Gunsolley JC. Periodontal host modulation with antiproteinase, anti-inflammatory, and bone-sparing agents. A systematic review. Ann Periodontol. 2003. 8:12–37.


17. Kim SJ, Ha MS, Choi EY, Choi JI, Choi IS. Prevotella intermedia lipopolysaccharide stimulates release of nitric oxide by inducing expression of inducible nitric oxide synthase. J Periodontal Res. 2004. 39:424–431.


18. Kim SJ, Ha MS, Choi EY, Choi JI, Choi IS. Nitric oxide production and inducible nitric oxide synthase expression induced by Prevotella nigrescens lipopolysaccharide. FEMS Immunol Med Microbiol. 2005. 43:51–58.


19. Kim SJ, Choi EY, Kim EG, Shin SH, Lee JY, Choi JI, et al. Prevotella intermedia lipopolysaccharide stimulates release of tumor necrosis factor-alpha through mitogen-activated protein kinase signaling pathways in monocyte-derived macrophages. FEMS Immunol Med Microbiol. 2007. 51:407–413.


20. Choi EY, Jin JY, Lee JY, Choi JI, Choi IS, Kim SJ. Melatonin inhibits Prevotella intermedia lipopolysaccharide-induced production of nitric oxide and interleukin-6 in murine macrophages by suppressing NF-κB and STAT1 activity. J Pineal Res. 2011. 50:197–206.


21. Geivelis M, Turner DW, Pederson ED, Lamberts BL. Measurements of interleukin-6 in gingival crevicular fluid from adults with destructive periodontal disease. J Periodontol. 1993. 64:980–983.


22. Mogi M, Otogoto J, Ota N, Inagaki H, Minami M, Kojima K. Interleukin 1 beta, interleukin 6, beta 2-microglobulin, and transforming growth factor-alpha in gingival crevicular fluid from human periodontal disease. Arch Oral Biol. 1999. 44:535–539.


23. Tamura T, Udagawa N, Takahashi N, Miyaura C, Tanaka S, Yamada Y, et al. Soluble interleukin-6 receptor triggers osteoclast formation by interleukin 6. Proc Natl Acad Sci U S A. 1993. 90:11924–11928.


24. Liu XH, Kirschenbaum A, Yao S, Levine AC. Cross-talk between the interleukin-6 and prostaglandin E(2) signaling systems results in enhancement of osteoclastogenesis through effects on the osteoprotegerin/receptor activator of nuclear factor-{kappa}B (RANK) ligand/RANK system. Endocrinology. 2005. 146:1991–1998.


25. Baeuerle PA, Henkel T. Function and activation of NF-kappa B in the immune system. Annu Rev Immunol. 1994. 12:141–179.
26. Rothwarf DM, Karin M. The NF-kappa B activation pathway: a paradigm in information transfer from membrane to nucleus. Sci STKE. 1999. 1999:RE1.
27. Karin M, Ben-Neriah Y. Phosphorylation meets ubiquitination: the control of NF-[kappa]B activity. Annu Rev Immunol. 2000. 18:621–663.
28. Covert MW, Leung TH, Gaston JE, Baltimore D. Achieving stability of lipopolysaccharide-induced NF-kappaB activation. Science. 2005. 309:1854–1857.


29. Li Q, Verma IM. NF-kappaB regulation in the immune system. Nat Rev Immunol. 2002. 2:725–734.
30. Ghosh S, May MJ, Kopp EB. NF-kappa B and Rel proteins: evolutionarily conserved mediators of immune responses. Annu Rev Immunol. 1998. 16:225–260.


31. Pfitzner E, Kliem S, Baus D, Litterst CM. The role of STATs in inflammation and inflammatory diseases. Curr Pharm Des. 2004. 10:2839–2850.


32. Schindler C, Darnell JE Jr. Transcriptional responses to polypeptide ligands: the JAK-STAT pathway. Annu Rev Biochem. 1995. 64:621–651.


33. Yamaoka K, Saharinen P, Pesu M, Holt VE 3rd, Silvennoinen O, O'Shea JJ. The Janus kinases (Jaks). Genome Biol. 2004. 5:253.


34. Schindler C, Levy DE, Decker T. JAK-STAT signaling: from interferons to cytokines. J Biol Chem. 2007. 282:20059–20063.


35. Gao JJ, Filla MB, Fultz MJ, Vogel SN, Russell SW, Murphy WJ. Autocrine/paracrine IFN-alphabeta mediates the lipopolysaccharide-induced activation of transcription factor Stat1alpha in mouse macrophages: pivotal role of Stat1alpha in induction of the inducible nitric oxide synthase gene. J Immunol. 1998. 161:4803–4810.