Abstract
Purpose
Bone tissues for clinical application can be improved by studies on osteoblast differentiation. Runx2 is known to be an important transcription factor for osteoblast differentiation. However, bone morphogenetic protein (BMP)-2 treatment to stimulate Runx2 is not sufficient to acquire enough bone formation in osteoblasts. Therefore, it is necessary to find other regulatory factors which can improve the transcriptional activity of Runx2. The erythroblast transformation-specific (ETS) transcription factor family is reported to be involved in various aspects of cellular proliferation and differentiation.
Methods
We have noticed that the promoters of osteoblast differentiation markers such as alkaline phosphatase (Alp), osteopontin (Opn), and osteocalcin (Oc) contain Ets binding sequences which are also close to Runx2 binding elements. Luciferase assays were performed to measure the promoter activities of these osteoblast differentiation markers after the transfection of Runx2, myeloid Elf-1-like factor (MEF), and Runxs+MEF. Reverse-transcription polymerase chain reaction was also done to check the mRNA levels of Opn after Runx2 and MEF transfection into rat osteoblast (ROS) cells.
Results
We have found that MEF, an Ets transcription factor, increased the transcriptional activities of Alp, Opn, and Oc. The addition of Runx2 resulted in the 2- to 6-fold increase of the activities. This means that these two transcription factors have a synergistic effect on the osteoblast differentiation markers. Furthermore, early introduction of these two Runx2 and MEF factors significantly elevated the expression of the Opn mRNA levels in ROS cells. We also showed that Runx2 and MEF proteins physically interact with each other.
Conclusions
Runx2 interacts with MEF proteins and binds to the promoters of the osteoblast markers such as Opn nearby MEF to increase its transcriptional activity. Our results also imply that osteoblast differentiation and bone formation can be increased by activating MEF to elicit the synergistic effect of Runx2 and MEF.
Bone remodeling is a response process to the functional demands of mechanical loading, and controls the reshaping or replacement of bone during growth and microfractures that occur during normal activity. The equilibrium between bone formation by osteoblasts and bone resorption by osteoclasts maintains a stable level of bone mass [1-8]. As far as the regulation of bone remodeling is concerned, many factors exist. The regulation of bone remodeling is accomplished by both systemic and local processes.
A transcription factor is a protein that binds to specific DNA sequences and thereby controls the transcription of genetic information from DNA to mRNA. It functions alone or with other proteins in a complex by promoting or blocking the recruitment of the RNA polymerase to the specific genes. In bone remodeling, many transcription factors such as Runx2 and osterix are known to be involved, and the deficiency or overexpression of some of these factors generates severe metabolic disorders in bone [1,9-12].
The erythroblast transformation-specific (ETS) families of transcription factors control the expression of genes that are critical for cell proliferation, differentiation, and cell death. Some ETS transcription factors are also known to regulate bone development [13]. ETS-1, the founding member of the ETS family, which was originally from avian E26 retrovirus, is reported to be expressed in proliferating preosteoblastic cells, whereas ETS-2 is expressed in the differentiated and mature osteoblasts [13].
Myeloid Elf-1-like factor (MEF) was originally isolated from a human megakaryocytic leukemia cell line [14]. MEF contains a central ETS DNA binding domain and is expressed in a variety of myeloid leukemia cell lines and in normal adult hematopoietic tissues as well as in non-hematopoietic tissues [14]. MEF is a member of the Elf-1/E74 family of ETS proteins and has DNA binding properties similar to those of Elf-1. Eighty of the 85 amino acids in the ETS domain are identical in the MEF and Elf-1 proteins [15]. It has been reported that MEF transcriptionally activates genes expressed in lymphoid, myeloid, and epithelial cells, such as interleukin-8 and human beta-defensin 2 [16,17].
In this study, we analyzed the transcriptional function of MEF in the bone marker genes, osteopontin (Opn), osteocalcin (Oc), and alkaline phosphatase (Alp). Also, since these promoters have Runx binding sites close to potential ETS binding sites, we have hypothesized that MEF and Runx2 might have functional cooperativity on these promoters. This study provides a novel insight into the role of MEF transcription factor in osteogenesis.
Fetal bovine sera were from GIBCO-BRL (Invitrogen Co., Carlsbad, USA). The RevertAIDTM strand cDNA synthesis kit for reverse transcription was from Fermentas (Vilnius, Lithuania). Lipofectamin Plus was from Invitrogen (Carlsbad, USA) and Taq polymerase, the dNTP mixture and G418 were from Promega (Madison, USA).
DNA polymerase was from Invitrogen, Taq polymerase and the dNTP mixture were from Progmega.
Rat osteoblast ROS17/2.8 cells from a rat osteosarcoma were maintained in α-MEM. The cell maintenance protocol and the culture conditions used were as previously described [18]. COS7 cells were maintained in DMEM/high glucose (Hyclone, Logan, USA) with 10% FBS and 1% antibiotic-antimycotic. Lipofectamine Plus reagent was used for the transfection of the ROS17/2.8 and COS7 cells according to the manufacturer's protocol.
The plasmid containing Runx2 was constructed by cloning a Runx2 cDNA into the BamH I and Xho I sites of pcDNA3.1-FLAG or the Hind III and Xho I sites of pcDNA3.1-Myc. MEF was cloned into the BamH I and Xho I sites of pcDNA3.1-FLAG. The mouse Alp promoter (-1838 bp) in the pGL3 basic vector was kindly provided by Harada et al. [19] Oc promoter (1050-luciferase) was from Towler et al. [20,21]. The Opn promoter (1983 bp) was amplified by nested polymerase chain reaction (PCR) from mouse genomic DNA. The resulting fragment was ligated into the XhoI and HindIII sites of the pGL2-Basic firefly luciferase reporter vector (Promega).
The day before transfection, COS7 cells were plated on 6-well plates at a density of 1.2 × 105 cells/well. The cells were transfected with Lipofectamine Plus reagent (Invitrogen Co., Carlsbad, USA) according to the manufacturer's protocol. Each transfection for luciferase assay was performed with the same amount of ETS cDNA (in pCDNA3.1-Flag), Runx2 + CBFbeta cDNA, or pcDNA3.1 (Mock) and the Opn, Oc, or Alp gene promoters-luciferase reporters. Total DNA of 0.5 µg per well was used in each transfection for the luciferase assay. Twenty four hours after transfection, the cells were harvested for the luciferase assay. The supernatants from cell lysates were used for the luciferase assay system (Promega Co., Madison, USA). The results presented are representative data from at least two independent experiments with triplicate wells in each experiment.
Total cellular RNA was extracted from cells using TRIzol reagent and its concentration was measured by spectrophotometry. Total RNA was reverse-transcribed to produce cDNA using the Fermentas reverse transcription kit, according to the manufacturer's protocol. For reverse-transcription PCR (RT-PCR) analysis, mouse GAPDH specific primers were used as previously described [18]. To determine the Opn mRNA levels, RT-PCR was performed using the following primers: Opn forward 5'-TTCACAGCCACAAGGACAAG-3', Opn reverse 5'-TTACAACGGTGTTTGCATGA-3'.
Each luciferase assay and real-time PCR experiment was done in triplicate. Numerical data from representative investigations are expressed as mean ± SEM. The significance of the differences was determined using two-tailed Student's t-tests. A value of P < 0.05 was considered to indicate a significant difference.
We first investigated whether the promoters of the bone marker genes Opn, Oc, and Alp have an ETS responsive element (ERE) and Runx responsive element (RRE). More importantly, we focused on whether ERE and RRE are present close by each other, with about a 10.5 base pair distance, because the double helix DNA 1 turn is 10.5 base pairs and if the binding sites are this distance and multiples of this distance close by, it strongly suggests that the two transcription factors have a synergistic effect in their transactivity as long as the two proteins also have binding affinities. It is well known that Runx1 and MEF proteins have protein-protein interactions [22].
In Opn promoter, ETS responsive elements were observed in the -347 to -338 and -213 to -208 regions. RRE was found from -229 to -223 (Fig. 1A). Second ERE had a distance spanning from 10 to 21 base pairs with the RRE sites. This observation strongly suggests the cooperativity of the Runx and Ets proteins in this Opn promoter. In Oc promoter, Runx responsive elements were observed in the -634 to -629 and -599 to -594 regions. ERE was found in -576 to -563 (Fig. 2A). Second RRE had a distance spanning from 18 to 36 base pairs from the RRE sites. This observation also strongly suggests the cooperativity of the Runx and Ets proteins in this Oc promoter. In the Alp promoter, ETS responsive elements were found in the -1,140 to -1,130 and -701 to -689 regions. RRE was found in the -1,115 to -1,109 and -716 to -710 regions (Fig. 3A). The first ERE and first RRE exist at a distance of 15 to 31 base pairs from each other, and the second RRE and second ERE at 9 to 27 base pairs from each other. This observation also strongly suggests there are two cooperativities of the Runx and ETS proteins in the Alp promoter. However, in the bone sialoprotein (BSP) promoter, we could not find any RRE and ERE close by in the proximal promoter region.
We then tested whether the bone marker gene promoters respond to MEF and Runx2, and whether they show any functional cooperativities in the transactivating powers on these promoters. Cos7 cells were transfected with each luciferase construct and MEF cDNA with Runx2 +CBFbeta cDNA constructs.
In the Opn-luciferase (Opn-Luc) assay, we found that among the ETS transcription factors tested, MEF showed the highest luciferase activity (Fig. 1B). Furthermore, when Runx2 + CBFbeta was added, the activity was increased 4.5-fold, although this Opn promoter showed minimal transactivity to only Runx2 + CBFbeta transfection. Thus, MEF and Runx2 co-transfection resulted in a significant synergistic effect on the Opn-Luc promoter activity. In the Oc-luciferase assay, we found that among ETS transcription factors tested, ETV-1, ETS-2, and MEF showed high luciferase activity (Fig. 2B). Although EVT-1 and ETS-2 showed high transactivities on the Oc promoter, it did not show any further increase of the promoter activities upon Runx2 + CBFbeta addition. However, in MEF transfection, Runx2 + CBFbeta addition resulted in about a 2-fold increase, which is more than the additive amount of Runx2 + CBFbeta (grey bar in Oc-Luc graph) and MEF. Thus, MEF and Runx2 co-transfection also showed a significant synergistic effect on Oc-Luc promoter activity. In the Alp-luciferase assay, we found that Runx2 + CBFbeta cDNA transfection only resulted in relative luciferase activity (RLA) of about 20,000. MEF transfection showed an RLA of about 40,000. However, co-transfection of the expression vectors MEF and Runx2 + CBFbeta resulted in an RLA of 100,000 (Fig. 3B). Again, with the Alp promoter, MEF and Runx2 co-transfection had a significant synergistic effect on Alp-Luc promoter activity. However, in the BSP promoter, co-transfection of Runx2 + CBFbeta with ETS did not show any synergistic effect (Figs. 4A and B). This coincides well with the observation that there are no sequences with ERE and RRE close by in the proximal region of the BSP promoter.
We then tested whether MEF can induce endogenous Opn expression at early treatment of MEF (24 hours after transfection). ROS17/2.8 rat osteosarcoma cells were transfected with Mef cDNA alone, Runx2+CBF-beta cDNA, or MEF together with Runx2 + CBFbeta. MEF transfection resulted in higher Opn mRNA expression than Runx2+CBFbeta transfection. When the cells were transfected with MEF and Runx2 + CBFbeta together, the Opn mRNA expression was highly increased, suggesting again that MEF and Runx2 proteins might have a synergistic effect on Opn gene expression.
Our data showed that for the synergistic effect of MEF with Runx2 on the bone marker gene promoters, it required those two transcription factor responsive elements (RRE and ERE) existing close by on the proximal regions of the promoters. Thus, those promoters such as Opn, Oc, and Alp, having these two elements close by, showed a significant synergistic effect of the promoter activities whereas the BSP promoter which does not have RRE and ERE close by in the proximal regions of the promoter did not show any cooperative transactivity. It is interesting to note that the Opn, Oc, and Alp promoters have the RRE and ERE very close by in 10 to 40 bp ranges,and also that the binding sites seem to be 10.5, 21, or 31.5 distances, which is one, two or three rounds of the double-helix DNA structure.
Our data demonstrated that MEF and Runx2 have a functional synergistic effect on Opn, Oc, and Alp promoters. However, we only showed the direct effect of MEF and its cooperativity with Runx2 in the bone marker gene promoters. We still cannot tell what the long term effect of MEF is on these promoters. That is, if MEF can elevate a transcriptional repressor, the long term effect can be overcome and MEF itself might have even the opposite effect on bone marker gene expression. Thus, the long term effect of MEF needs to be further studied.
Opn, a sialic acid-rich phosphoprotein, is a major non-collagenous bone matrix protein and has been suggested to play an important role in ossification. Previously, the overlapping localization of Opn and Runx2 mRNA, and the marked decrease of Opn mRNA expression in Runx2 knockout mice have been reported, indicating that the transcription of the Opn gene was controlled by Runx2 [23]. It has been also reported that Opn promoter activity was markedly enhanced by Runx2 and ETS-1 in a synergistic manner [24]. The synergistic effect was diminished when either the Runx2 or ETS-1 binding site was mutated. The direct interaction between Runx2 and ETS-1 depended on protein-DNA binding. These results also suggest that the specific spatial arrangement of both sites and direct interaction between Runx2 and ETS-1 are essential for promoter function. These findings also support that Runx2 and ETS-1 cooperate to regulate expression of the Opn gene.
This promoter is also known to be regulated in carcinogensis. The same RRE and ERE region was also found to be bound by Runx2 and ETS-1, although these two factors decreased the Opn expression [25]. This difference might be due to the different cell context and ETS-1 and MEF characteristics. Also, this suppressive result might be due to the delayed time-dependant secondary effect.
In conclusion, our data showed that Runx2 and MEF bind nearby on the promoters of the osteoblast markers such as Opn, Oc, and Alp and to increase their transcriptional activities in synergistic manner. Our results also imply that osteoblast differentiation and bone formation can be increased by activating MEF to elicit the synergistic effect of Runx2 and MEF.
Figures and Tables
Figure 1
Synergistic effects of erythroblast transformation-specific (ETS) and Runx2 on the osteopontin (Opn) promoter. (A) Sequence analysis of the Opn promoter showed potential ETS and Runx2 binding elements. (B) Opn-luciferase (Opn-Luc) promoter construct (1,983 bp) was co transfected with ETS transcription factors as designated with or without Runx2 + CBFbeta into COS7 cells. Twenty-four hours after transfection the luciferase activity was measured as described in the Materials and Methods. In the Opn-Luc graph, the black bar is for only pCDNA3.1 vector and the gray bar is for Runx2 + CBFbeta as expression cDNA.
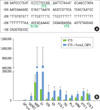
Figure 2
Synergistic effects of erythroblast transformation-specific (ETS) and Runx2 on the osteocalcin (Oc) promoter. (A) Sequence analysis of the Oc promoter showed potential ETS and Runx2 response elements. (B) Oc-luciferase (Oc-Luc) promoter construct (1,080 bp) was co-transfected with ETS transcription factors as designated with or without Runx2 + CBFbeta into COS7 cells. Twenty-four hours after transfection, the luciferase activity was measured. In Oc-Luc graph, the black bar is for pCDNA3.1 vector only and the grey bar is for Runx2 + CBFbeta as expression cDNA.
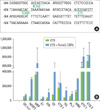
Figure 3
Synergistic effects of erythroblast transformation-specific (ETS) and Runx2 on the alkaline phosphatase (Alp) promoter. (A) Sequence analysis of the Alp promoter showed potential ETS and Runx2 binding elements. (B) Alp-luciferase (Alp-Luc) promoter construct (1.9 kb) was co-transfected with myeloid Elf-1-like factor (MEF) transcription factor with or without Runx2 + CBFbeta into COS7 cells. Twenty-four hours after transfection the luciferase activity was measured. In the Alp-Luc graph, the black bar is for pCDNA3.1 vector only and the grey bar is for Runx2 + CBFbeta as expression cDNA.
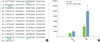
Figure 4
(A) Bone sialoprotein-luciferase (BSP-Luc) promoter construct was co-transfected with myeloid Elf-1-like factor (MEF) transcription factor with or without Runx2 + CBFbeta into COS7 cells. Twenty-four hours after transfection the luciferase activity was measured. In BSP-Luc graph, the black bar is for only pCDNA3.1 vector and grey bar is for Runx2 + CBFbeta as expression cDNAs. (B) ROS17/2.8 cells were transfected with Mock (pCDNA3.1), MEF, Runx2, or MEF together with Runx2. Runx2 was always introduced with CBFbeta cofactor on the mark of 'Runx2.' Twenty-four hours after transfection, the cells were harvested, RNA was collected and RT-PCR were performed with Opn primers as described in the Materials and Methods.
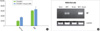
ACKNOWLEDGEMENTS
This work was supported by the Korea Research Foundation Grant funded by the Korean Government (MOEHRD) (KRF-2005-E00149) and by a grant from Korea Health 21 R&D Project (No. A090610).
References
1. Boyle WJ, Simonet WS, Lacey DL. Osteoclast differentiation and activation. Nature. 2003. 423:337–342.


2. Blair HC, Zaidi M, Schlesinger PH. Mechanisms balancing skeletal matrix synthesis and degradation. Biochem J. 2002. 364:329–341.


4. Karsenty G, Wagner EF. Reaching a genetic and molecular understanding of skeletal development. Dev Cell. 2002. 2:389–406.


5. Lemaire V, Tobin FL, Greller LD, Cho CR, Suva LJ. Modeling the interactions between osteoblast and osteoclast activities in bone remodeling. J Theor Biol. 2004. 229:293–309.


7. Ducy P, Schinke T, Karsenty G. The osteoblast: a sophisticated fibroblast under central surveillance. Science. 2000. 289:1501–1504.


8. Harada S, Rodan GA. Control of osteoblast function and regulation of bone mass. Nature. 2003. 423:349–355.


10. Katagiri T, Takahashi N. Regulatory mechanisms of osteoblast and osteoclast differentiation. Oral Dis. 2002. 8:147–159.


11. Wagner EF, Karsenty G. Genetic control of skeletal development. Curr Opin Genet Dev. 2001. 11:527–532.


12. Deng ZL, Sharff KA, Tang N, Song WX, Luo J, Luo X, et al. Regulation of osteogenic differentiation during skeletal development. Front Biosci. 2008. 13:2001–2021.


13. Raouf A, Seth A. Ets transcription factors and targets in osteogenesis. Oncogene. 2000. 19:6455–6463.


14. Miyazaki Y, Sun X, Uchida H, Zhang J, Nimer S. MEF, a novel transcription factor with an Elf-1 like DNA binding domain but distinct transcriptional activating properties. Oncogene. 1996. 13:1721–1729.
15. Suico MA, Koyanagi T, Ise S, Lu Z, Hisatsune A, Seki Y, et al. Functional dissection of the ETS transcription factor MEF. Biochim Biophys Acta. 2002. 1577:113–120.


16. Hedvat CV, Yao J, Sokolic RA, Nimer SD. Myeloid ELF-1 like factor is a potent activator of interleukin-8 expression in hematopoietic cells. J Biol Chem. 2004. 279:6395–6400.


17. Lu Z, Kim KA, Suico MA, Shuto T, Li JD, Kai H. MEF up-regulates human beta-defensin 2 expression in epithelial cells. FEBS Lett. 2004. 561:117–121.
18. Lee MH, Kim YJ, Kim HJ, Park HD, Kang AR, Kyung HM, et al. BMP-2-induced Runx2 expression is mediated by Dlx5, and TGF-beta 1 opposes the BMP-2-induced osteoblast differentiation by suppression of Dlx5 expression. J Biol Chem. 2003. 278:34387–34394.


19. Harada H, Tagashira S, Fujiwara M, Ogawa S, Katsumata T, Yamaguchi A, et al. Cbfa1 isoforms exert functional differences in osteoblast differentiation. J Biol Chem. 1999. 274:6972–6978.


20. Towler DA, Bennett CD, Rodan GA. Activity of the rat osteocalcin basal promoter in osteoblastic cells is dependent upon homeodomain and CP1 binding motifs. Mol Endocrinol. 1994. 8:614–624.


21. Towler DA, Rutledge SJ, Rodan GA. Msx-2/Hox 8.1: a transcriptional regulator of the rat osteocalcin promoter. Mol Endocrinol. 1994. 8:1484–1493.


22. Lacorazza HD, Nimer SD. The emerging role of the myeloid Elf-1 like transcription factor in hematopoiesis. Blood Cells Mol Dis. 2003. 31:342–350.


23. Komori T, Yagi H, Nomura S, Yamaguchi A, Sasaki K, Deguchi K, et al. Targeted disruption of Cbfa1 results in a complete lack of bone formation owing to maturational arrest of osteoblasts. Cell. 1997. 89:755–764.

