Abstract
Purpose
Connective tissue reattachment to periodontally damaged root surfaces is one of the most important goals of periodontal therapy. The aim of this study was to develop a root conditioning agent that can demineralize and detoxify the infected root surface.
Methods
Dentin slices obtained from human teeth were treated with a novel root planing agent for 2 minutes and then washed with phosphate-buffered saline. Smear layer removal and type I collagen exposure were observed by scanning electron microscopy (SEM) and type I collagen immunostaining, respectively. Cell attachment and lipopolysaccharides (LPS) removal demonstrated the efficiency of the root conditioning agent.
Results
SEM revealed that the smear layer was entirely removed and the dentinal tubules were opened by the experimental gel. Type I collagen was exposed on the surfaces of the dentin slices treated by the experimental gel, which were compared with dentin treated with other root planing agents. Dentin slices treated with the experimental gel showed the highest number of attached fibroblasts and flattened cell morphology. The agar diffusion assay demonstrated that the experimental gel also has effective antimicrobial activity. Escherichia coli LPS were effectively removed from well plates by the experimental gel.
Periodontitis is a chronic disease caused by periodontal pathogens and results in the destruction of periodontium [1]. To regenerate periodontal tissue, new connective tissue attachments to the root surfaces must first be achieved [2-5]. This process is controlled by fibroblast migration and attachment to root surface collagen fibrils. Root surfaces affected by periodontal diseases can undergo structural and chemical changes due to the effects of cytotoxic and other biologically active substances from periodontal pathogens [6-8]. This bacterial contamination prevents the reattachment of gingival and periodontal cells and cannot be reversed by the use of conventional scaling and root planing procedures alone, as they are not able to fully eliminate the infection due to the production of a compact smear layer [7,9-11]. Because the presence of a smear layer is unsuitable for reattachment of periodontal connective tissue, the purpose of surface demineralization is to recreate a biologically active substrate for periodontal cellular reattachment [3-5]. Therefore, chemical conditioning agents are often used to help remove root surface impurities including minerals and cytotoxic materials derived from bacterial products [12,13]. The demineralized root surface may also serve as a reservoir and retention site for biologically active extracellular matrix proteins and growth factors that could positively affect the wound healing environment [14,15]. In addition, exposed matrix proteins might initiate deposition of calcium and related new minerals, which is essential for cementum and bone formation [16]. Despite differences in clinical applications, ethylenediaminetetraacetic acid (EDTA), tetracycline, and citric acid have all been used as conditioning agents [5-7]. Ideally, the demineralization step should have a balanced aggressiveness to remove all instrumentation debris and to dissolve the smear layer and the mineral phase, properly exposing dentin matrix proteins but not altering the structural and biochemical properties of the exposed proteins [5]. In endodontology, EDTA is used to open calcified canals, to eliminate smear layer with possible infection [17] and to reduce possible microleakage [18]. EDTA is a unique molecule that has six potential binding sites for metal ions. EDTA forms stable complexes with Ca ions, demineralizes the root canal surface, and therefore, in some cases, simplifies root canal preparation. Smear layer removal is achieved with different preparations of EDTA such as liquid, paste [19], or gel application forms [20]. The aim of this study was to develop a root conditioning agent that can demineralize and detoxify root surfaces. The base component of the root conditioning agent is the adhesive polymer sodium carboxymethyl cellulose (CMC). The proper concentration of CMC gives the adhesive a gel-like quality, thus making it easy to apply to the root surface. EDTA and Triton X-100 were included in the experimental root conditioning gel. Scanning electron microscopy (SEM) observation was performed to verify the removal of the smear layer as well as followed cell attachment on the clear dentin slices after treatment with the experimental agent. Type I collagen immunostaining on dentin slices after treatment with the experimental agent was observed by confocal microscopy. The antimicrobial effect and remaining lipopolysaccharides (LPS) levels after treatment were demonstrated by agar diffusion and LPS detection assays.
Sodium CMC was purchased from Woonpoong Co. (Seoul, Korea). EDTA-2Na, Triton-X100, chlorhexidine digluconate, and citric acid were purchased from Sigma (St. Louis, MO, USA). Cell culture media were from Gibco (Invitrogen, Grand Island, NY, USA). Tryptic soy agar was purchased from Difco Laboratories (Detroit, MI, USA) containing 15.0 g pancreatic digest of casein, 5.0 g enzymatic digest of soybean meal, 5.0 g sodium chloride, and 15.0 g agar. Tryptic soy broth was also purchased from Difco Laboratories (Detroit, MI, USA) containing 17.0 g pancreatic digest of casein, 3.0 g enzymatic digest of soybean meal, 2.5 g dextrose, 5.0 g sodium chloride, and 2.5 g dipotassium phosphate. Bacterial strains used for the antimicrobial activity assays included Staphylococcus aureus (IFO 2340), Bacillus cereus (IFO 13597), Escherichia coli (IFO 3628), Prevotella intermedia (ATCC256211), Actinomyces israelii (ATCC12103), and Fusobacterium nucleatum (ATCC 25586) strains. The strains were stored at -80℃.
The experimental planing gel was prepared with EDTA and Triton X-100 provided by NIBEC (Seoul, Korea). To compare the demineralization effects and antimicrobial effects, 24% EDTA, 1% Triton X-100, 0.5% chlorhexidine, and 10% citric acid were combined in a 3% CMC solution, and the pH was then adjusted to 7.
Human teeth were extracted as a part of routine treatment at the Department of Periodontology, Seoul National University. Patient consent was obtained according to the guidelines of the School of Dentistry for the use of human samples in research. Teeth were immediately extracted, crowns were removed, and the roots were vertically cut and divided into three specimens (approximately 4×4×1 mm in size) using a low speed diamond saw under water irrigation. The dentin slices were planed and stored in phosphate-buffered saline (PBS), pH 7.4 at 4℃ until use. The dentin slices were immersed for 2 minutes in each root planing agent followed by at least three washes in distilled water. The controls were not treated with a root planing agent.
Immediately after rinsing, the tissue blocks were fixed in 2% glutaraldehyde in PBS for 15 minutes. After three 5-minute PBS washes, the blocks were subjected to further fixation with 1% OsO4 in 0.1 M PBS buffer for 30 minutes at room temperature and then rinsed in 0.1 M PBS buffer for 10 minutes and twice with distilled water for 10 minutes. Samples were then dehydrated in a sequence of 70%, 80%, and 90% ethanol solutions for 10 minutes, followed by washing with 95% and 100% ethanol for 10 minutes in a two times. The samples were then freeze-dried and mounted onto aluminum plates. The dried samples were coated with gold and observed by field emission scanning electron microscopy (FE-SEM, S-4700, JEOL, Tokyo, Japan) at a voltage of 15 kV.
NIH3T3 cells were plated in T75 flasks for continuous passaging in α-MEM medium supplemented with 10% fetal bovine serum (Gibco, Invitrogen, Grand Island, NY, USA) and 1% antibiotic-antimycotic solution (Gibco, Invitrogen, Grand Island, NY, USA). The medium was changed twice weekly, and cells were trypsinized and passaged into fresh culture flasks at a ratio of 1:4 upon reaching confluence. Cultures were incubated at 37℃ in a humidified incubator with 5% CO2.
Because the square dentin slices did not fit into the rounded culture wells, the appropriate number of cells per well was suspended in 50 µL of α-MEM medium, and the suspension was placed directly on the surface of the dentin block. After a 1-hour incubation period, 250 µL of culture medium was added to the suspension, and it was further incubated for 24 hours. The dentin slices were then fixed in 2% glutaraldehyde in PBS for 15 minutes following the method described in the scanning electron microscopy section. The dried samples were coated with gold and observed by FE-SEM at a voltage of 15 kV.
Immunolabeling of the root dentin specimens was performed using mouse monoclonal immunoglobulin G (IgG) anti-type I collagen (Sigma Chemical Co., St. Louis, MO, USA) to identify the presence and distribution patterns of collagen according to the method described by Breschi et al. [21] with some modifications. Briefly, specimens were rinsed in PBS at pH 7.4 and incubated in blocking buffer (1% bovine serum albumin [BSA] in PBS) for 1 hour at room temperature. Primary antibody incubations were performed overnight in 1% BSA/PBS (1:500) at 4℃, and the specimens were then washed three times for 10 minutes with PBS. Samples were incubated with fluorescein isothiocyanate (FITC) conjugated goat anti-mouse IgG (1:1,000 in 1% BSA/PBS) as a secondary antibody, for 1 hour at room temperature and then washed with PBS three times for 10 minutes. Observation of collagen structures was accomplished using an Olympus FV-300 laser scanning microscope operated with FluoView software (Olympus, Tokyo, Japan).
S. aureus, B. cereus, and E. coli cultures were placed in 5 mL of tryptic soy broth, which was then incubated with constant agitation at 37℃ for 24 hours. At this stage, the cultures of S. aureus, B. cereus, and E. coli each contained approximately 109 colony-forming units (CFU) per mL. Bacterial concentrations were determined spectrophotometrically (OD at 520 nm) by species-specific curves. All strains were diluted in PBS and plated in concentrations of 105 and 107 bacteria/mL on tryptic soy agar plates and were incubated at 37℃ for 24 hours. The number of bacterial cells in each culture was calculated by multiplying the number of colonies by the dilution factors. P. intermedia, A. israelii, and F. nucleatum were plated to concentrations of 105 and 107 bacteria/mL on Columbia agar plates containing Vitamin K (0.1%) and hemin (0.25%).
Twenty microliters of root planing agents and PBS were applied to the filter paper disks, which were allowed to dry at 37℃ and then placed on the bacteria-inoculated agar plates. After application of these substances, the plates were incubated under aerobic and strictly anaerobic conditions at 37℃. The inhibition diameters (ID) were measured after 5 days of incubation by two calibrated blinded examiners. The experiments were repeated at least four times.
Bacterial endotoxin concentration was detected using the HEK-Blue LPS detection kit (InvivoGen, San Diego, CA, USA). An LPS standard (E. coli K12 LPS) was coated on 24-well plates at a concentration of 100 ng/mL, allowed to incubate overnight at 4℃ and then washed three times with PBS. Root conditioning agents (200 µL) were applied to each well for 2 minutes and then washed three times with PBS. HEK-Blue cells were resuspended in detection media to a concentration of 4×104 cells/well and then seeded onto 24-well plates and incubated overnight at 37℃. The absorbance was measured at 620 nm using a microplate reader (BioTek, Winooski, VT, USA). The absorbance values of wells coated with endotoxin-free water and washed with PBS served as blanks. The relative absorbance ratio was determined by the following equation:
Relative absorbance ratio=absorbance of LPS coated and sample-treated well/absorbance of endotoxin-free water-coated well
Fig. 1 shows the surface of a dentin slice treated with of the each root conditioning agents. No specimens subjected to the surface cleaning treatment showed residual calculus or amorphous debris on the surface. These samples also revealed a thick, mineralized dentin smear layer. Nowhere in the specimens were the dentin surfaces or any patent dentinal tubules visible. Citric acid-, chlorhexidine-, and triton X-100-treated dentin slices were covered with homogenous smear layers and no observable open dentinal tubules. Remnants of debris were seen in most of the dentinal tubules. The surfaces of samples treated with 24% EDTA for 2 minutes showed removal of the smear layer. However, partially patent dentinal tubules were visible on the dentin surfaces. Approximately half of the tubules were plugged, but the total number of visible tubule openings was higher than in citric acid-, chlorhexidine-, or triton X-100-treated dentin slices. The dentin surface was readily visible in all root planed "diseased" areas as evidenced by lack of a smear layer and an abundance of patent dentinal tubules. The experimental gel-treated root surfaces showed complete smear layer removal and displayed widened dentinal tubules, which appeared as funnel shapes due to the removal of the peritubular dentin. The root canal walls were free of debris, and the dentinal tubule entrances were visible.
Fig. 2 shows exposed type I collagen on the surface of dentin slices treated with each root conditioning agent. Significant differences between the specimens were identified for both type I collagen fibrils. The experimental gel-treated specimens revealed the highest FITC labeling for type I collagen fibrils, confirming the exposure of the organic matrix. Twenty-four percent EDTA-conditioned samples revealed FITC labeling for collagen fibrils that appeared lower than the observed labeling of the experimental gel-treated samples due to the remaining smear layer on the dentin slices. Specimens that did not receive any treatment showed no apparent FITC labeling in dentin slices, thus confirming that collagen fibrils were not visible. In the cases the of citric acid-, chlorhexidine-, and Triton X-100-treated specimens, FITC labeling was almost undetectable, as collagen fibrils were still embedded in the mineralized components of the dentin slices.
Fig. 3 shows the morphology of attached cells on the surface of dentin slices treated with each of the root conditioning agents. The cells exhibited distinctly different morphologies depending upon the surface treatment agent. When cultured on experimental gel-treated dentin, NIH3T3 cells formed a monolayer. The cells had cellular extensions and seemed to be flat. Cells grown on 24% EDTA, citric acid, chlorhexidine, or Triton X-100-treated dentin slices were smaller and somewhat elongated, with a more cuboidal shape than cultures grown on experimental gel-treated surfaces. On surfaces that received no treatment, individual cells were small and round in appearance and no cellular extensions were observed.
Differing degrees of inhibition of bacteria growth were found with the experimental gel, 24% EDTA, chlorhexidine, and PBS (Fig. 4). The experimental gel-treated disks inhibited more than 23 mm of bacterial growth for six strains. The student's t-test analysis showed a statistically significant difference between the ID of the experimental gel and that of PBS. The ID measurements of 24% EDTA and chlorhexidine were lower than that of the experimental gel. However, the inhibition by Triton-X100 and citric acid failed to reach statistical significance relative to PBS. The pattern of anaerobic bacterial growth was similar to that of aerobic bacterial growth.
The normalized LPS level is shown in Fig. 5. LPS derived from E. coli was coated on 24-well plates overnight, root planing agents were added, and the wells were washed with PBS. An untreated well washed with PBS served as the control. The HEK-Blue cells were seeded and incubated overnight. According to the manufacturer, the HEK-Blue LPS Detection kit is based on the ability of proprietary HEK-Blue cells engineered to be extremely sensitive to LPS to recognize structurally different LPS from gram-negative bacteria and lipid A, their particular toxic moiety for TLR4 detection. The presence of very low concentrations of LPS, starting as low as 0.3 ng/mL, is detected by the HEK-Blue-4 cells, leading to the activation of NF-κB. By adding a specific detection medium, NF-κB activation can be quantified spectrophotometrically. The absorbance at 620 nm increased as the remaining LPS concentration increased. The experimental gel-treated wells showed the lowest LPS level when compared to wells treated with other root planing agents. Cells treated with Triton X-100-treated showed similar LPS level with experimental gel-treated wells. Twenty-four percent EDTA and chlorhexidine treated wells showed slight removal of the LPS compared with untreated wells. Chlorhexidine had no effect on the removal of LPS, even when used as an antimicrobial agent. In contrast, Triton X-100 had significant effects on the removal of LPS.
The smear layer has been described as a layer composed of very small particles of mineralized collagen matrix [22]. Studies have suggested that the presence of this smear layer interposed between the root surface and the adjacent connective tissue may serve as a physical barrier to the development of a connective tissue attachment to the root surface [5]. It has been shown that EDTA treatment of root dentin by burnishing action results in the removal of the smear layer [23]. In the current study, surfaces that received only root planing treatment presented an amorphous, irregular coating with little evidence of patent dentinal tubules. This characteristic appearance was probably due to the presence of a surface smear layer. Treatment with citric acid, chlorhexidine, or triton-X100 was not effective for the removal of the smear layer. In periodontal therapy, citric acid has been the most commonly used root planing agent [24], but a recent study has indicated that EDTA may be an alternative choice [23]. In vivo study by other group reported that conditioning with EDTA solution with neutral pH did not evoke tissue necrosis, however, conditioning with acidic citric acid induced necrosis of flap along with adjacent periodontium even in 20 seconds of exposure [23]. A recent in vitro study demonstrated that EDTA applied for 3 minutes and citric acid applied for 20 seconds by the rubbing technique could remove mineral build-up from the dentin surfaces, exposing a matrix structurally resembling collagen fibers of the unmineralized predentin [8]. Treatment of dentin specimens with 24% EDTA and the experimental gel resulted in partial and total disappearance of the amorphous surface, respectively. The application of the experimental gel was more effective than 24% EDTA in removing the smear layer.
The dentin organic matrix is characterized by a complex network of fibrillar and globular structures [21,25]. Type I collagen represents the main component of the dentin fibrillar network and is held together by non-collagenous proteins [21,26]. Standard cleaning procedures such as scaling and root planing resulted in a thick smear layer mainly determined by residual inorganic debris and microparticles of dentin/cement organic matrix [8,27]. Because this smear layer acts as a physical barrier to reattachment [28], it negatively affects cell growth and may enclose bacterial colonies and toxins [27]. Various chemical treatments have been suggested over the years to remove the smear layer and disinfect the remaining substrate [29]. It was demonstrated that the application of the experimental gel was effective for exposing collagen fibrils by removing smear layer from the surface of the dentin and thus improved cell attachment on the root surface. The demineralized dentin surfaces are more biocompatible with NIH3T3 cell attachment than untreated dentin surfaces. Demineralization of dentin surfaces might more effectively expose such collagen fibrils and allow them to undergo an enhanced periodontal regeneration process. The attachment rate of fibroblasts was significantly higher when compared to the control group, supporting the reported results that the experimental gel appeared to promote early cell and tissue colonization by providing a more biocompatible surface for cell and tissue attachment.
The antimicrobial effect of the experimental gel resulted from the chelation of cations from the outer membranes of bacteria [30]. In a clinical study, Yoshida et al. [31] examined the effectiveness of 15% EDTA solution used in combination with ultrasound treatment. After 1 week, no bacteria could be found in 93 root canals out of a total of 129 cases. EDTA proved to have a greater antimicrobial effect than saline. Chlorhexidine was the first antimicrobial agent shown to inhibit dental plaque formation and the development of chronic gingivitis [32]. The antibacterial mode of action is explained by the fact that the positively charged bis-biguanide molecule is rapidly attracted by the negatively charged bacterial tooth surfaces and oral mucosal cell surfaces due to substantial increase of antibacterial activity through controlled release of the agent [33]. It was demonstrated that the experimental gel exerted more antimicrobial activity than chlorhexidine, even if chlorhexidine was used as a common oral antimicrobial agent.
The 0.1-0.5% Triton X-100 has been used as a permeabilizing agent in eukaryotic cells and preparations with concentrations higher than 1%. Triton X-100 has the ability to permeabilize the bacterial cell wall. Schnaitman reported that the nonionic detergent Triton X-100 removed 50% of LPS from E. coli with no alteration in morphology of the cell wall [34]. Gram negative bacteria contain, in the outermost membranes of their cell walls, an LPS complex generally referred to as endotoxin that is capable of inducing many biological effects (e.g., complement activation, cytotoxicity, and bone resorption). A polysaccharide moiety is a potent antigen that can elicit antibody formation even in submicrogram concentrations [35]. Anaerobic Gram-negative bacteria have been frequently isolated from root canals of endodontically treated teeth; consequently, their endotoxins may affect the periapical tissues and exert a role in the pathogenesis of inflammatory lesions of pulpal origin [36]. The experimental gel used in this study was effective for antimicrobial activity and removal of LPS.
In conclusion, the experimental gel sufficiently demineralized, detoxified, and also exposed type I collagen on dentin slices. Antimicrobial activity of aerobic and anaerobic bacteria was clearly demonstrated by agar diffusion assay, and E. coli-derived LPS was removed after treatment with the experimental gel. Taken together, these results show that the experimental gel may be useful as a root conditioning agent for dentin surfaces and can also be used to detoxify infected implant surfaces.
Figures and Tables
Figure 1
Surface morphology of human dentin slices after treatment by tested root conditioning agents as analyzed by scanning electron microscopy. Each root planing agent was applied to dentin slices for 2 minutes and washed with phosphate-buffered saline. (A) Experimental gel, (B) ethylenediaminetetraacetic acid, (C) citric acid, (D) chlorhexidine, (E) Triton X-100, and (F) no treatment. Magnification ×500.
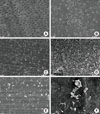
Figure 2
Histochemical study of the exposed type I collagen on human dentin slices by confocal microscope. Each root planing agent was applied to dentin slices for 2 minutes and then washed with phosphate-buffered saline. (A) Experimental gel, (B) ethylenediaminetetraacetic acid, (C) citric acid, (D) chlorhexidine, (E) Triton X-100, and (F) no treatment. Magnification ×100.
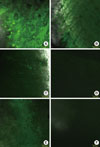
Figure 3
Histochemical study of the attached cell morphology of human dentin slices by scanning electron microscopy. Each root planing agent was applied to dentin slices for 2 minutes and washed with phosphate-buffered saline. (A) Experimental gel, (B) ethylenediaminetetraacetic acid, (C) citric acid, (D) chlorhexidine, (E) Triton X-100, and (F) no treatment. Magnification ×500.
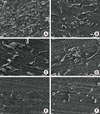
Figure 4
Comparative study of the antimicrobial activity of root planing agents. (A) Inhibition diameter of aerobic bacteria cultured agar plate. (B) Inhibition diameter of anaerobic bacteria cultured agar plate. 1: experimental gel, 2: ethylenediaminetetraacetic acid, 3: citric acid, 4: chlorhexidine, 5: Triton X-100, 6: phosphate-buffered saline (PBS). The data represent the mean±SD from triplicate experiments (N=4). *P<0.05, compared to PBS-treated filter paper diameter.
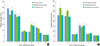
Figure 5
Normalized lipopolysaccharides (LPS) levels remaining on the Escherichia coli LPS coated wells after treatment with root planing agents. 1: experimental gel, 2: ethylenediaminetetraacetic acid, 3: citric acid, 4: chlorhexidine, 5: Triton X-100, 6: no treatment. The data represent the mean±SD from triplicate experiments (N=4). *P<0.05, compared to no treatment.
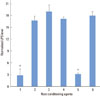
ACKNOWLEDGEMENTS
This study was supported by the Ministry of Health, Welfare and Family Affairs (#A085056), Republic of Korea.
References
1. Martorelli de Lima AF, Cury CC, Palioto DB, Duro AM, da Silva RC, Wolff LF. Therapy with adjunctive doxycycline local delivery in patients with type 1 diabetes mellitus and periodontitis. J Clin Periodontol. 2004. 31:648–653.


2. Nishimine D, O'Leary TJ. Hand instrumentation versus ultrasonics in the removal of endotoxins from root surfaces. J Periodontol. 1979. 50:345–349.


3. Baker PJ, Rotch HA, Trombelli L, Wikesjo UM. An in vitro screening model to evaluate root conditioning protocols for periodontal regenerative procedures. J Periodontol. 2000. 71:1139–1143.


4. Wikesjo UM, Selvig KA. Periodontal wound healing and regeneration. Periodontol 2000. 1999. 19:21–39.


5. Polson AM, Frederick GT, Ladenheim S, Hanes PJ. The production of a root surface smear layer by instrumentation and its removal by citric acid. J Periodontol. 1984. 55:443–446.


6. Isik G, Ince S, Saglam F, Onan U. Comparative SEM study on the effect of different demineralization methods with tetracycline HCl on healthy root surfaces. J Clin Periodontol. 1997. 24:589–594.


7. Babay N. The effect of EDTA on the attachment and growth of cultured human gingival fibroblasts in periodontitis-affected root surface. J Contemp Dent Pract. 2001. 2:13–23.
8. Blomlof J, Lindskog S. Root surface texture and early cell and tissue colonization after different etching modalities. Eur J Oral Sci. 1995. 103:17–24.


9. Babay N. Attachment of human gingival fibroblasts to periodontally involved root surface following scaling and/or etching procedures: a scanning electron microscopy study. Braz Dent J. 2001. 12:17–21.
10. Blomlof J, Lindskog S. Periodontal tissue-vitality after different etching modalities. J Clin Periodontol. 1995. 22:464–468.


11. Barkmeier WW, Jabro MH, Latta MA. Scanning electronic microscopic analysis of the local effects of a periodontal scaling gel on selected surfaces. J Clin Dent. 1992. 3:39–42.
12. Chandra RV, Jagetia GC, Bhat KM. The attachment of V79 and human periodontal ligament fibroblasts on periodontally involved root surfaces following treatment with EDTA, citric acid, or tetracycline HCL: an SEM in vitro study. J Contemp Dent Pract. 2006. 7:44–59.


13. Rompen EH, Goffinet GH, Nusgens B. Human periodontal ligament fibroblast behavior on chemically conditioned dentine: an in vitro study. J Periodontol. 1999. 70:1144–1152.


14. Karp W, Sodek J, Aubin JE, Melcher AH. A comparison of fibronectin and laminin binding to undemineralized and demineralized tooth root surfaces. J Periodontal Res. 1986. 21:30–38.


15. McAllister B, Narayanan AS, Miki Y, Page RC. Isolation of a fibroblast attachment protein from cementum. J Periodontal Res. 1990. 25:99–105.


16. Egelberg J. Regeneration and repair of periodontal tissues. J Periodontal Res. 1987. 22:233–242.


17. Aktener BO, Bilkay U. Smear layer removal with different concentrations of EDTA-ethylenediamine mixtures. J Endod. 1993. 19:228–231.


18. Czonstkowsky M, Wilson EG, Holstein FA. The smear layer in endodontics. Dent Clin North Am. 1990. 34:13–25.
19. Hulsmann M, Heckendorff M, Lennon A. Chelating agents in root canal treatment: mode of action and indications for their use. Int Endod J. 2003. 36:810–830.


20. Boveda C, Fajardo M, Millan B. Root canal treatment of an invaginated maxillary lateral incisor with a C-shaped canal. Quintessence Int. 1999. 30:707–711.
21. Breschi L, Gobbi P, Lopes M, Prati C, Falconi M, Teti G, et al. Immunocytochemical analysis of dentin: a double-labeling technique. J Biomed Mater Res A. 2003. 67:11–17.


22. Pashley DH. Smear layer: physiological considerations. Oper Dent Suppl. 1984. 3:13–29.
23. Blomlof J. Root cementum appearance in healthy monkeys and periodontitis-prone patients after different etching modalities. J Clin Periodontol. 1996. 23:12–18.


24. Larjava H, Salonen J, Hakkinen L, Narhi T. Effect of citric acid treatment on the migration of epithelium on root surfaces in vitro. J Periodontol. 1988. 59:95–99.


25. Perdigao J, Lambrechts P, Van Meerbeek B, Vanherle G, Lopes AL. Field emission SEM comparison of four postfixation drying techniques for human dentin. J Biomed Mater Res. 1995. 29:1111–1120.


26. Breschi L, Perdigao J, Gobbi P, Mazzotti G, Falconi M, Lopes M. Immunocytochemical identification of type I collagen in acid-etched dentin. J Biomed Mater Res A. 2003. 66:764–769.


27. Pitaru S, Aubin JE, Gray A, Metzger Z, Melcher AH. Cell migration attachment and orientation in vitro are enhanced by partial demineralization of dentine and cementum and inhibited by bacterial endotoxin. J Periodontal Res. 1984. 19:661–665.


28. Heritier M. Effects of phosphoric acid on root dentin surface. A scanning and transmission electron microscopic study. J Periodontal Res. 1984. 19:168–176.
29. Wikesjo UM, Nilveus RE, Selvig KA. Significance of early healing events on periodontal repair: a review. J Periodontol. 1992. 63:158–165.


30. DePamphilis ML. Dissociation and reassembly of Escherichia coli outer membrane and of lipopolysaccharide, and their reassembly onto flagellar basal bodies. J Bacteriol. 1971. 105:1184–1199.


31. Yoshida T, Shibata T, Shinohara T, Gomyo S, Sekine I. Clinical evaluation of the efficacy of EDTA solution as an endodontic irrigant. J Endod. 1995. 21:592–593.


32. Daneshmand N, Jorgensen MG, Nowzari H, Morrison JL, Slots J. Initial effect of controlled release chlorhexidine on subgingival microorganisms. J Periodontal Res. 2002. 37:375–379.

