Abstract
Objective
The purpose of this study was to examine the effect of commercially available fluoride-containing oral rinses on the corrosion behavior of titanium alloys, which are the main components of orthodontic miniscrews.
Methods
Four commercially available oral rinses (solution A, pH 4.46/260 ppm fluoride; solution B, pH 4.41/178 ppm fluoride; solution C, pH 6.30/117 ppm fluoride; and solution D, pH 4.17/3.92 ppm fluoride) were tested on titanium alloy (Ti-6Al-4V) circular plates, and saline was used as the control. The open-circuit potential and potentiodynamic polarization of these materials were measured. Thereafter, all samples were evaluated under a field-emission scanning electron microscope.
Results
Among the tested oral rinses, except solution D, the more the fluoride content was, the greater was the corrosion potential downtrend; the corrosion resistance of the titanium alloy sample was also lowered significantly (p < 0.05). Field-emission scanning electron microscopic analysis of the surface morphology of the titanium alloy samples revealed that all samples had some defects, crevices, or pitting after exposure to the oral rinses than before treatment. In particular, the samples in solution A showed the most changes.
Titanium and its alloys are considered good metallic implant materials because of their high corrosion resistance, excellent biocompatibility with oral tissues, and mechanical strength.1 Titanium implants are widely used for skeletal anchorage in orthodontic treatment because of their many advantages such as flexibility in site placement, low cost, simple implant procedure, and less patient trauma.2
Numerous studies have assessed the risk factors associated with miniscrew failure. One complication is miniscrew fracture during placement or removal, which sometimes makes it difficult to remove the fragment from inside the bone.3 Another complication is periscrew inflammation, which can damage the bone surrounding the neck of the miniscrew.345
Fluoride-containing adhesives, pastes, and oral rinses are commonly used to prevent dental caries and tooth demineralization in orthodontic treatment.67 Clinical evidence indicates that treatment using fluoridecontaining materials is beneficial for managing and preventing white spot lesions during fixed orthodontic treatment.8 However, many reports have suggested that fluoride causes corrosion of titanium implants, and this can lead to implant fracture due to stress corrosion.9101112 A solution containing more than 30 ppm hydrofluoric acid can cause corrosion by destroying the oxidation film of titanium.131415 Therefore, more focus should be placed on dissolved metal ions such as aluminum and vanadium ions that may cause cytotoxic effects, adverse tissue reactions, and stimulate carcinogenic reactions.16171819202122232425 The corrosion of titanium also has a potential effect on general health. Nevertheless, information related to the effects of these oral rinses on the corrosion behavior of titanium alloy miniscrews is lacking. Therefore, a well-controlled study on this issue is necessary.
The purpose of this study was to examine the effects of commercially available fluoride-containing oral rinses on the corrosion behavior of titanium alloys, which are the main components of orthodontic miniscrews. The null hypothesis of this study was that there would be no significant difference in the corrosion behavior of titanium alloys in oral rinses containing different levels of fluoride.
Twenty-five commercial titanium alloy (Ti-6Al-4V) circular plates (TC4; Sheng Xinyuan Titanium Industry Co., Ltd., Baoji, China) were examined under each set of test conditions in this study (n = 5 plates for each condition). The alloy specimens (diameter, 15 mm; thickness, 2 mm) were used for electrochemical corrosion tests and determination of surface characteristics. They were polished with #400-, #600-, #800-, #1,200-, and #2,000-grit silicon carbide sandpaper and mirrorpolished using a 1-micron diamond grinding paste. They were then ultrasonically cleaned for 10 minutes in alcohol and 10 minutes in distilled water. Electrochemical tests were performed using a VersaSTAT3 potentiostat (Model 300; AMETEK, Inc., Berwyn, PA, USA), which was coupled with the VersaStudio 2.43.3 software (AMETEK, Inc.) used for electrochemical control and data analyses.
The four commercially available oral rinses (pH, 4.17–6.30; fluoride concentration, 3.92–260 ppm; volume, 400 mL) that were used as the test solutions and saline, which was used as the control solution, are presented in Table 1. All products were subjected to pH measurement and a fluoride ion concentration test. The temperature of the test solutions was maintained at 37℃ ± 0.1℃ during the electrochemical analysis of the titanium alloys.
The counter electrode was graphite and the reference electrode was a potassium chloride (KCl)-saturated silver/silver chloride (Ag/AgCl) electrode. The potential of the reference electrode was denoted by E (Ag/AgCl) = E (saturated hydrogen electrode) + 197 mV. The test samples were connected as the working electrode and immersed for 2 hours in the test solution. Based on the findings of a previous study,26 the 2 hours of immersion used in this study corresponds approximately to the use of an oral rinse for 6 months (i.e., 40 seconds daily), which is similar to the normal orthodontic treatment time with a fixed miniscrew appliance. The exposure area of the working electrode was 0.95 mm2.
After the open-circuit potential (OCP) was monitored for 2 hours, a potentiodynamic polarization measurement was conducted. Because of the low OCP value measured on titanium in the test solutions, the potentiodynamic polarization was initiated at −1.5 V and continued until 0.5 V, at a scan rate of 10 mV/s.27 The potentiodynamic polarization was subsequently subjected to the Tafel tests arranged from an Eocp (OCP/natural electrode potential) of −250 mV to +250 mV to identify the corrosion current density (Icorr), the corrosion potential at Icorr (Ecorr), and the corrosion rate at Icorr (Rcorr).14
The morphology of the titanium alloy surfaces before (i.e., control) and after the electrochemical measurements were examined under a field-emission scanning electron microscope (FE-SEM) (JSM-7001F; JEOL, Ltd., Tokyo, Japan).
After conducting the Shapiro–Wilk normality tests, one-way analysis of variance with the post-hoc Tukey test was used to compare the corrosion resistance of the titanium alloy samples immersed in the fluoride-containing solutions to that of the control alloy samples immersed in saline. All data analyses were conducted using IBM SPSS Statistics ver. 23.0 (IBM Corp., Armonk, NY, USA) at a significance level of 0.05.
The changes in the OCP versus time in the four oral rinses containing different amounts of fluoride ions and in saline are shown in Figure 1. Under the open-air condition, the OCP values decreased more significantly over time in solutions A and B than in the other solutions. The OCP values on the curve of solution A decreased more rapidly than did those on the curve of solution B. The curves of solutions C and D revealed stable OCP values (to approximately 0. 2 V) in 2 hours. The OCP value of saline increased quickly during the first few minutes, and later gradually increased to nearly 0.2 V in the remaining time.
The potentiodynamic polarization curves recorded for the titanium alloy samples in saline and the four oral rinses containing different fluoride concentrations and different pH values are shown in Figure 2. The graph was drawn with the minimum values in each group.
A marked difference was observed in the corrosion potential between the five solutions. The corrosion potential of the solutions in sequence from high to low was as follows: solution C, saline, solution B, solution D, and solution A.
When a stable passive current plateau occurred, the values of current density in solutions A and B were higher than those of solutions C and D. The value of solution A was higher than that of solution B. In addition, the values of current density in solution C, solution D, and saline were similar.
Five samples in each solution were tested. The means and standard deviations of Icorr, Ecorr, and Rcorr are shown in Table 2. Statistically significant differences were observed between the five solutions in Icorr, Ecorr, and Rcorr (p < 0.05).
Statistically significant differences were also observed in the Icorr between solutions A and B, solutions A and C, and solution A and saline (p < 0.05). No statistically significant difference was observed in the Ecorr between solutions A and D and between solution B and saline. Moreover, statistically significant differences were observed in the Rcorr between solutions A and B, solutions A and C, and solution A and saline (p < 0.05).
The surface morphology of the titanium samples assessed using FE-SEM after the electrochemical corrosion tests (i.e., OCP and potentiodynamic polarization) are shown in Figure 3. Sample A primarily had scratches and defects originating from the grinding and polishing operation performed during the pretest. No clear signs of any general or localized corrosion were observed on its surface. However, the samples in the other four oral rinses and in saline had localized corrosion. The sample in solution A had many more defects, crevice corrosion, and pitting than did the other samples. The sample in solution B also had some defects and pitting, but not as much as in solution A. The samples in solutions C and D and in saline had lesser pitting than did the samples in solutions A and B.
To replicate clinical conditions, the present study used some commercially available oral rinses as the experimental solutions and saline as the control solution. A previous study28 showed that pure titanium and titanium alloys may easily corrode in an acidic environment, even at low fluoride concentrations.
According to the findings of the present study, as shown in Figure 1, the OCP values of the titanium alloy decreased in solutions A and B, and especially so in solution A. A previous study29 showed that a decrease in the OCP of a metallic material in a certain environment revealed an increase in its chemical reactivity, and a subsequent predisposition to corrosion. Thus, we can infer that the chemical reactivity of the titanium alloy in solutions A and B increased with a probable change in the properties of the titanium oxide surface film. The alloy samples in solutions C and D revealed a similar and relatively stable chemical reactivity at a noble potential. The corrosion potential gradually increased over time in saline, suggesting that the alloy samples maintained good corrosion resistance.
As shown by the anodic polarization curve, when a stable passive current plateau occurred, the current density values in solutions A, B, and C decreased in sequence with increasing fluoride concentration (Figure 2). The greater the current density, the greater the dissolution of metallic ions. This could indicate that the corrosion resistance of the titanium alloy decreases as the fluoride concentration increases.
The Ecorr values of the potentiodynamic polarization test revealed statistically significant differences between each solution pair, except between solutions A and D and between solution B and saline. In solutions A, B, and C, the mean Ecorr values decreased in sequence with increasing fluoride concentration (Table 2). This result indicated a reduction in the corrosion resistance of the titanium alloy as well as in the stability of its passive film when the fluoride concentration increased.
The mean Rcorr value also increased with increasing fluoride concentration, except in solution D. This finding suggested that the corrosion rates of the titanium alloy in solutions A, B, and C increased with increasing fluoride concentration.
The mean Ecorr was higher in solution C than in saline, even though the mean Icorr and mean Rcorr were much lower in saline than in solution C. An interpretation of this finding is that the actual corrosion rate was higher in solution C than in saline.
The pH values of solutions A and B were similar at approximately 4.4 (Table 1). The comparison of solutions A and B showed that the corrosion resistance of the titanium alloy decreased with increasing fluoride concentration. Previous studies152730 have shown that the decrease in the corrosion resistance of titanium or titanium alloys was more significant in a solution containing a greater concentration of fluoride at the same pH, and that a solution containing a higher concentration of fluoride could dissolve more metal ions.
As for solution D (which has no fluoride, according to the manufacturer), there may be several reasons why it reduced the corrosion resistance of the titanium alloy. There may have been a little fluoride in solution D, even though it was not listed by the manufacturer. The pH value in solution D was the lowest among the four oral rinses. Moreover, although no statistically significant difference was observed between solutions A and D, a comparison of the mean Ecorr showed that the sample in solution D had a higher value than did the sample in solution A. A previous study28 showed that a solution with a lower pH value induced a lower corrosion resistance in the titanium alloy at the same fluoride concentration. Solution D had a lower pH value than did solution A; despite this, the corrosion resistance of the alloy sample was higher in solution D than in solution A, which contained more fluoride. This result may be related to the concentration of fluoride in the oral rinses. Previous studies2731 have also demonstrated that a solution with a low pH value and a lower fluoride concentration could have induced a better corrosion resistance in the titanium alloy than did a solution with a higher fluoride concentration and a higher pH value. The fluoride content itself may be crucial for corrosion. Another possibility is that some other components in the rinses could have reduced the corrosion resistance.
According to the surface morphology of titanium samples observed under FE-SEM after electrochemical corrosion tests (Figure 3), the samples in solution A showed many more defects, crevice corrosion, and pitting corrosion than did the other samples. The greater the extent of pitting corrosion and crevice corrosion present on the surface of the samples, the more the corrosion. The surface of the alloy in saline also seemingly had some slight crevice corrosion and pitting corrosion, but this may have been caused by the autocatalytic reaction of chloride ions. When a metal surface has a pit or crevice, the quantity of metal cations increases inside the defect than in the other parts of the metal because of the lack of oxygen. To maintain a charge balance, more chloride ions move to the pit or crevice and cause an autocatalytic reaction. This process can cause metal corrosion. The results in Figure 3 could consequently indicate that the greater concentration of fluoride caused greater corrosion in the samples.
The results of the study partially rejected the null hypothesis. Among the tested oral rinses, except solution D, the more the fluoride content was, the lower was the corrosion resistance of the titanium alloy sample. Therefore, the long-term use of oral rinses with a high fluoride concentration is likely to influence the properties of titanium orthodontic appliances such as titanium-molybdenum alloy wires, miniscrews, and brackets.
Previous studies2731 have shown that a solution with a low pH value and no fluoride could induce a better corrosion resistance in the titanium alloy than does a solution that has a low fluoride concentration and a high pH value. If there had been another control group with a fluoride-free acidic solution in this study, it may have clarified the crucial impact of fluoride content on corrosion resistance. In addition, the evaluation method and small sample size may have limited the applicability of the results of this study. Future studies should aim to have larger sample sizes and use more clinically relevant methods to verify the current findings.
Fluoride has been used for preventing caries during orthodontic treatment. However, commercially available oral rinses that have a high concentration of fluoride and a low pH value may reduce the corrosion resistance of titanium alloys used in dental appliances such as orthodontic titanium miniscrews and brackets.
References
1. Choi SH, Jeong WS, Cha JY, Lee JH, Yu HS, Choi EH, et al. Time-dependent effects of ultraviolet and nonthermal atmospheric pressure plasma on the biological activity of titanium. Sci Rep. 2016; 6:33421. PMID: 27627871.


2. Choi SH, Kim SJ, Lee KJ, Sung SJ, Chun YS, Hwang CJ. Stress distributions in peri-miniscrew areas from cylindrical and tapered miniscrews inserted at different angles. Korean J Orthod. 2016; 46:189–198. PMID: 27478796.


3. Park HS, Jeong SH, Kwon OW. Factors affecting the clinical success of screw implants used as orthodontic anchorage. Am J Orthod Dentofacial Orthop. 2006; 130:18–25. PMID: 16849067.


4. Cheng SJ, Tseng IY, Lee JJ, Kok SH. A prospective study of the risk factors associated with failure of mini-implants used for orthodontic anchorage. Int J Oral Maxillofac Implants. 2004; 19:100–106. PMID: 14982362.
5. Venugopal A, Muthuchamy N, Tejani H, Gopalan AI, Lee KP, Lee HJ, et al. Incorporation of silver nanoparticles on the surface of orthodontic microimplants to achieve antimicrobial properties. Korean J Orthod. 2017; 47:3–10. PMID: 28127534.


6. Sudjalim TR, Woods MG, Manton DJ, Reynolds EC. Prevention of demineralization around orthodontic brackets in vitro. Am J Orthod Dentofacial Orthop. 2007; 131:705.e1–705.e9.


7. Mellberg JR, Ripa LW. Fluoride in preventive dentistry: theory and clinical applications. Chicago: Quintessence Pub Co;1983.
8. Lopatiene K, Borisovaite M, Lapenaite E. Prevention and treatment of white spot lesions during and after treatment with fixed orthodontic appliances: a systematic literature review. J Oral Maxillofac Res. 2016; 7:e1.


9. Toumelin-Chemla F, Rouelle F, Burdairon G. Corrosive properties of fluoride-containing odontologic gels against titanium. J Dent. 1996; 24:109–115. PMID: 8636481.


10. Lausmaa J, Kasemo B, Hansson S. Accelerated oxide growth on titanium implants during autoclaving caused by fluorine contamination. Biomaterials. 1985; 6:23–27. PMID: 3971014.


11. Könönen MH, Lavonius ET, Kivilahti JK. SEM observations on stress corrosion cracking of commercially pure titanium in a topical fluoride solution. Dent Mater. 1995; 11:269–272. PMID: 8621050.


12. Boere G. Influence of fluoride on titanium in an acidic environment measured by polarization resistance technique. J Appl Biomater. 1995; 6:283–288. PMID: 8589513.


13. Souza JCM, Barbosa SL, Ariza E, Celis JP, Rocha LA. Simultaneous degradation by corrosion and wear of titanium in artificial saliva containing fluorides. Wear. 2012; 292-293:82–88.


14. Oshida Y, Sellers CB, Mirza K, Farzin-Nia F. Corrosion of dental metallic materials by dental treatment agents. Mater Sci Eng C. 2005; 25:343–348.


15. Nakagawa M, Matsuya S, Shiraishi T, Ohta M. Effect of fluoride concentration and pH on corrosion behavior of titanium for dental use. J Dent Res. 1999; 78:1568–1572. PMID: 10512392.


16. Wang JJ, Sanderson BJ, Wang H. Cyto- and genotoxicity of ultrafine TiO2 particles in cultured human lymphoblastoid cells. Mutat Res. 2007; 628:99–106. PMID: 17223607.


17. Urban RM, Jacobs JJ, Tomlinson MJ, Gavrilovic J, Black J, Peoc'h M. Dissemination of wear particles to the liver, spleen, and abdominal lymph nodes of patients with hip or knee replacement. J Bone Joint Surg Am. 2000; 82:457–476. PMID: 10761937.


18. Manda MG, Psyllaki PP, Tsipas DN, Koidis PT. Observations on an in-vivo failure of a titanium dental implant/abutment screw system: a case report. J Biomed Mater Res B Appl Biomater. 2009; 89:264–273. PMID: 18837452.
19. Manaranche C, Hornberger H. A proposal for the classification of dental alloys according to their resistance to corrosion. Dent Mater. 2007; 23:1428–1437. PMID: 17466365.


20. Goodman SB. Wear particles, periprosthetic osteolysis and the immune system. Biomaterials. 2007; 28:5044–5048. PMID: 17645943.


21. Case CP, Langkamer VG, James C, Palmer MR, Kemp AJ, Heap PF, et al. Widespread dissemination of metal debris from implants. J Bone Joint Surg Br. 1994; 76:701–712. PMID: 8083255.


22. Broggini N, McManus LM, Hermann JS, Medina RU, Oates TW, Schenk RK, et al. Persistent acute inflammation at the implant-abutment interface. J Dent Res. 2003; 82:232–237. PMID: 12598555.


23. Guindy JS, Schiel H, Schmidli F, Wirz J. Corrosion at the marginal gap of implant-supported suprastructures and implant failure. Int J Oral Maxillofac Implants. 2004; 19:826–831. PMID: 15623057.
24. Oh EJ, Nguyen TD, Lee SY, Jeon YM, Bae TS, Kim JG. Enhanced compatibility and initial stability of Ti6Al4V alloy orthodontic miniscrews subjected to anodization, cyclic precalcification, and heat treatment. Korean J Orthod. 2014; 44:246–253. PMID: 25309864.


25. Golvano I, Garcia I, Conde A, Tato W, Aginagalde A. Influence of fluoride content and pH on corrosion and tribocorrosion behaviour of Ti13Nb13Zr alloy in oral environment. J Mech Behav Biomed Mater. 2015; 49:186–196. PMID: 26042765.


26. Muguruma T, Iijima M, Brantley WA, Yuasa T, Kyung HM, Mizoguchi I. Effects of sodium fluoride mouth rinses on the torsional properties of miniscrew implants. Am J Orthod Dentofacial Orthop. 2011; 139:588–593. PMID: 21536200.


27. Souza JC, Barbosa SL, Ariza EA, Henriques M, Teughels W, Ponthiaux P, et al. How do titanium and Ti6Al4V corrode in fluoridated medium as found in the oral cavity? An in vitro study. Mater Sci Eng C Mater Biol Appl. 2015; 47:384–393. PMID: 25492211.


28. Nakagawa M, Matsuya S, Udoh K. Corrosion behavior of pure titanium and titanium alloys in fluoride-containing solutions. Dent Mater J. 2001; 20:305–314. PMID: 11915624.


29. Blackwood DJ, Peter LM, Williams DE. Stability and open circuit breakdown of the passive oxide film on titanium. Electroch Acta. 1988; 33:1143–1149.


30. Robin A, Meirelis JP. Influence of fluoride concentration and pH on corrosion behavior of titanium in artificial saliva. J Appl Electroch. 2007; 37:511–517.


31. Nakagawa M, Matsuya S, Udoh K. Effects of fluoride and dissolved oxygen concentrations on the corrosion behavior of pure titanium and titanium alloys. Dent Mater J. 2002; 21:83–92. PMID: 12238790.


Figure 1
Representative open-circuit potential plots. Variations in the titanium alloy open-circuit potentials of four oral rinses containing (A) 260 ppm fluoride, (B) 178 ppm fluoride, (C) 117 ppm fluoride, (D) 3.92 ppm fluoride, and (S) saline.
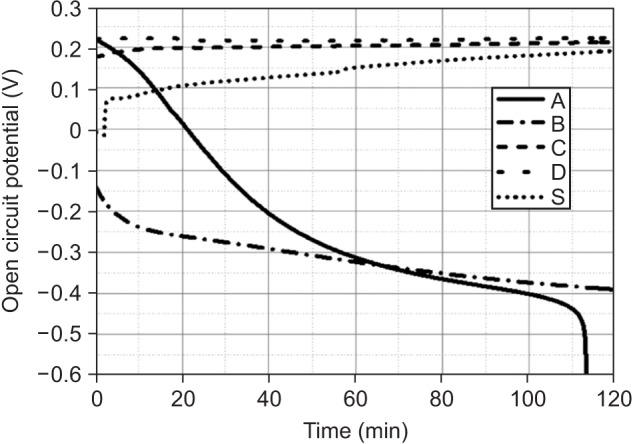
Figure 2
Representative potentiodynamic polarization plots. Titanium alloy samples in four oral rinses containing (A) 260 ppm fluoride, (B) 178 ppm fluoride, (C) 117 ppm fluoride, (D) 3.92 ppm fluoride, and (S) saline. The potential scan rate is 10 mV/s.
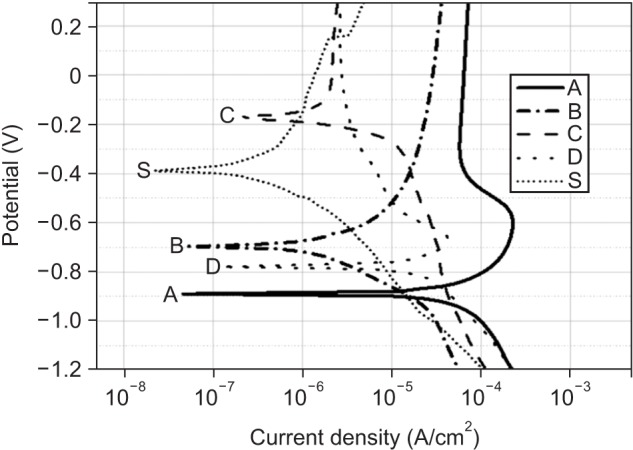
Figure 3
Surface morphology of the titanium samples under a field-emission scanning electron microscope after the electrochemical corrosion tests (magnification, 5,000×). (A) Micrograph of a sample before the electrochemical tests. Micrographs of samples after treatment with (B) solution A (260 ppm fluoride), (C) solution B (178 ppm fluoride), (D) solution C (117 ppm fluoride), (E) solution D (3.92 ppm fluoride), and (F) the saline solution. Scale bars = 5 µm.
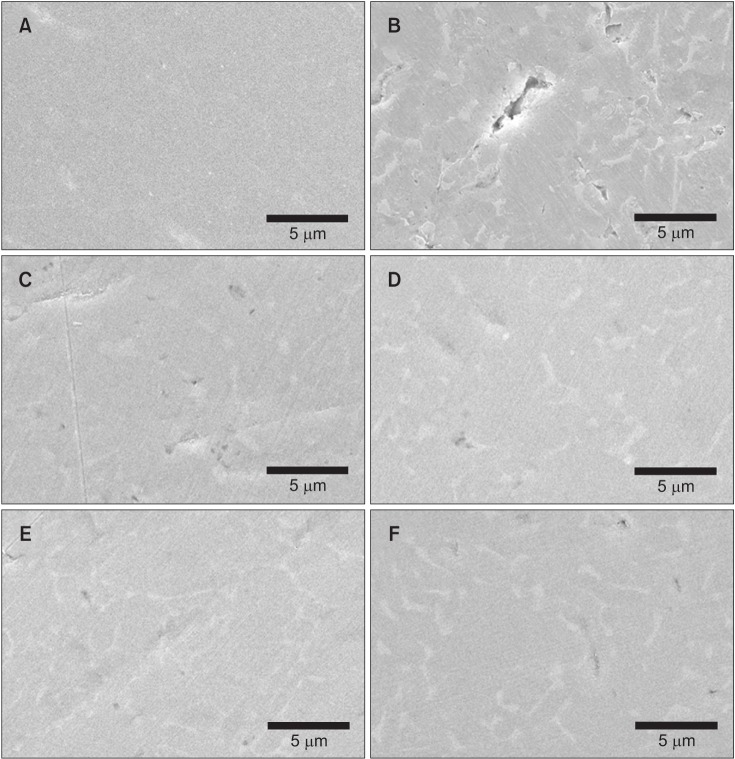
Table 2
Means and standard deviations (SD) of Icorr, Ecorr, and Rcorr in four oral rinses and saline
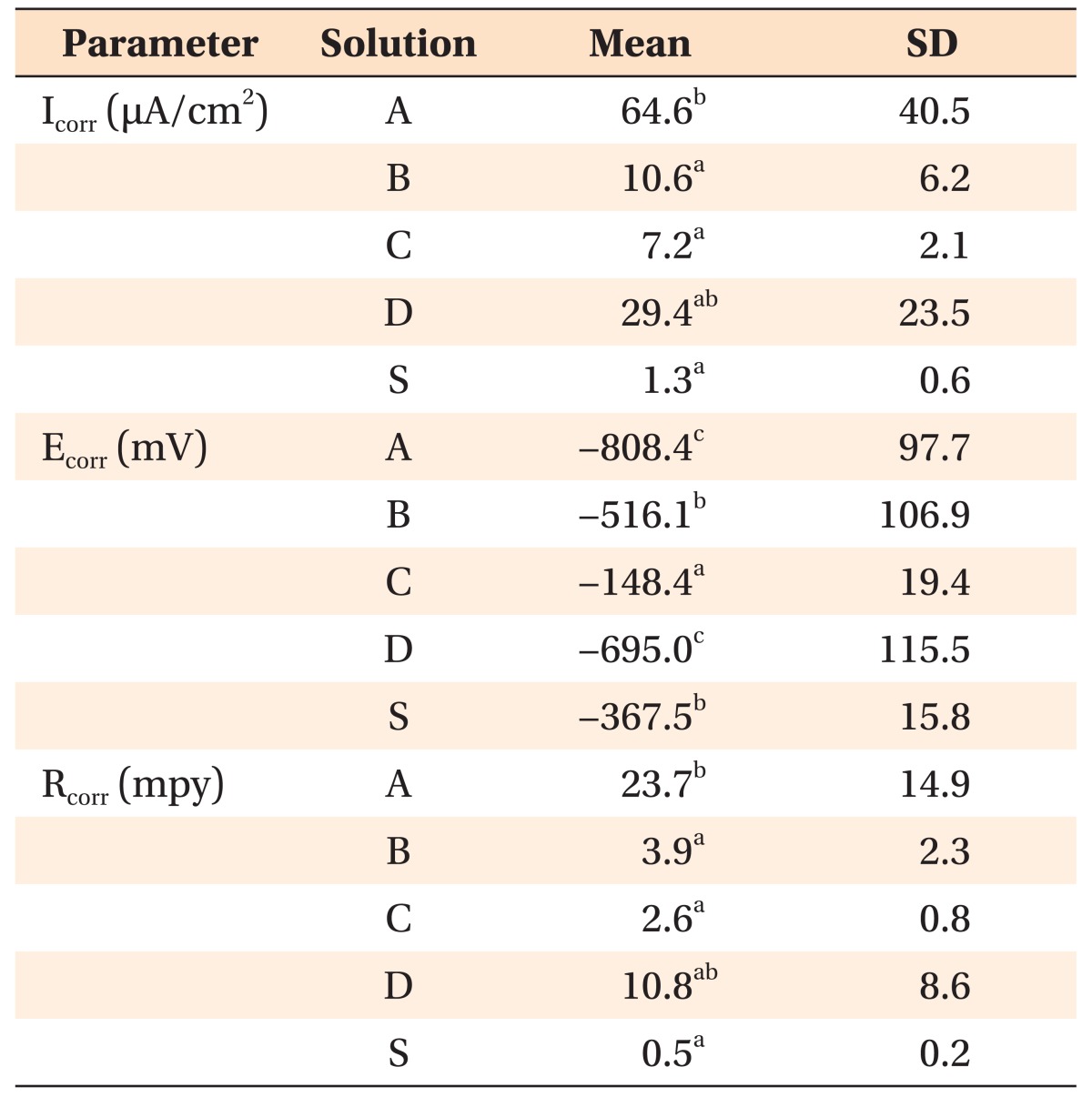
Icorr, corrosion current density; Ecorr, corrosion potential at Icorr; Rcorr, corrosion rate at Icorr.
The same superscript letters indicate no statistically significant difference between the groups (p > 0.05). Increasing group mean values are expressed in ascending alphabetical order.
Four oral rinses containing (A) 260 ppm fluoride, (B) 178 ppm fluoride, (C) 117 ppm fluoride, (D) 3.92 ppm fluoride, and (S) saline.