Abstract
Objective
Different methods have been utilized to prevent enamel demineralization and other complications during orthodontic treatment. However, none of these methods can offer long-lasting and effective prevention of orthodontic complications or interventions after complications occur. Considering the photocatalytic effect of TiO2 on organic compounds, we hoped to synthesize a novel bracket with a TiO2 thin film to develop a photocatalytic antimicrobial effect.
Methods
The sol-gel dip coating method was used to prepare TiO2 thin films on ceramic bracket surfaces. Twenty groups of samples were composed according to the experimental parameters. Crystalline structure and surface morphology were characterized by X-ray diffraction and scanning electron microscopy, respectively; film thickness was examined with a surface ellipsometer. The photocatalytic properties under ultraviolet (UV) light irradiation were analyzed by evaluating the degradation ratio of methylene blue (MB) at a certain time. Antibacterial activities of selected thin films were also tested against Lactobacillus acidophilus and Candida albicans.
Most orthodontic patients are adults, especially women. Because of their professions and other reasons (such as social interaction), these patients require less visible and more esthetic treatment. Because the color of ceramic brackets is close to that of natural teeth, they have become the first choice among these patients.12 Bracket irregularities can result in increased dental plaque formation, thus inducing enamel demineralization and other complications.34 Recent investigations determined that the incidence of white spot lesions was 73–95% in the orthodontic populations studied.5 Gorelick et al.6 showed that fixed orthodontic appliances induced a rapid increase in the volume of dental plaque. Furthermore, Chatterjee and Kleinberg7 showed that the plaque of orthodontic patients had a resting pH lower than that of non-orthodontic subjects. A rapid shift was reported in the bacterial flora of plaque after introducing orthodontic appliances.8 Scheie et al.9 observed significantly elevated levels of Streptococcus mutans in plaque and saliva after the insertion of orthodontic appliances. Both S. mutans and lactobacilli are often associated with caries development. Therefore, plaque control plays an important role in the prevention of demineralization.
Photocatalytic TiO2 has been widely applied in the pollution removal, environmental protection, and biomedical fields because of its great chemical stability,10 biocompatibility,111213 and self-cleaning ability14 since Fujishima and Honda15 reported the photo-induced and electrochemically assisted decomposition of water in 1972. The photo-killing of bacteria by exposure to photocatalytic TiO2 has also drawn much attention.161718 TiO2 becomes strongly oxidative when illuminated by ultraviolet (UV) light at wavelengths less than 385 nm, which generates holes and hydroxyl radicals in the valence band, and electrons and superoxide ions in the conduction band. By illuminating TiO2, photocatalysts can decompose organic compounds by participating in a series of oxidation reactions leading to the formation of carbon dioxide.19
A variety of techniques have been used for the preparation of TiO2 films including sputtering,20 chemical vapor deposition,21 physical vapor deposition,22 and electron beam evaporation.23 Among the many preparation techniques, the sol-gel method fulfills the requirements for nanostructured TiO2 and has numerous advantages, including purity, homogeneity, and stoichiometry control.242526 It is suitable for coating large and complex areas composed of many fine particles at low process costs, and offers numerous possibilities to vary film properties by changing the composition of the solution.27
We prepared a TiO2 thin film by using the radio frequency (RF) magnetron sputtering method in our previous study.28 However, the greatest drawback of this type of modified bracket is the change in color. After a series of experiments, we finally concluded that the color change of the thin film caused by the RF magnetron sputtering method could not be eliminated, despite changing the parameters and substrate. Our main consideration was to determine a method to modify a bracket without changing any of its original properties. Therefore, after studying the literature, we decided to prepare TiO2 thin films by using the solgel method. Thus, the issue of color change could be avoided to the greatest extent.
While ensuring the brackets' esthetic appearances would not be affected by the coatings, we aimed to (1) prepare TiO2 thin films on the surfaces of ceramic brackets using the sol-gel dip coating method; (2) characterize surface morphology by scanning electron microscopy, examine crystalline structure by X-ray diffractometer, and evaluate film thickness by surface ellipsometer; (3) test photocatalytic ability via methylene blue (MB) decomposition; and (4) evaluate antibacterial activity against a common oral microbes.
Ceramic brackets were provided by a manufacturer of orthodontic brackets (Standard MBT monocrystal ceramic brackets, BR212-33; Hangzhou Xingchen 3B Dental Instrument Material Co., Ltd., Zhejiang Province, China). The substrates were ultrasonically cleaned in 99.5% acetone, 99% ethanol, and deionized water for 30 minutes in each, and then dried in nitrogen.
TiO2 thin films were prepared using the sol-gel dip coating method. Tetrabutyl titanate was used as the precursor and absolute ethanol as the solvent. Acetylacetone (AcAc) was added as a chelating agent to decrease the reactivity and stabilize the sol. Acetic acid (Ac) was added to initiate hydrolysis by an esterification reaction with ethanol. Briefly, a TiO2 sol was prepared by dropping proportional amounts of tetrabutyl titanate and AcAc into ethanol. After stirring for 30 minutes, Ac was added to the solution. The solution was then stirred until a transparent yellow sol was obtained. The molar ratio of the TiO2 sol was 1:0.3:0.2:34 for Ti/AcAc/Ac/ethanol. The solution was continually stirred for 2.5 hours and subsequently aged for 24 hours at ambient temperature in room air. The thickness of the TiO2 films could be adjusted by repeating the dipping cycle. Just after coating, the precursor films were dried at 100℃ for 15 minutes. Finally, dried gel films were annealed at different temperatures for 2 hours (Table 1). The rate of heating was 5℃/minute. Samples were then stored in Petri dishes for further use.
The surface morphology was examined by scanning electron microscopy (SEM; Hitachi S-4800, Hitachi High-Technologies Corporation, Tokyo, Japan). The crystalline structure of the prepared thin films was identified by using a D/Max-2400 X-ray diffractometer (XRD; Rigaku, Tokyo, Japan) with Ni-filtered Cu Kα radiation. The thickness of the film was measured with a surface ellipsometer (NanoMap500LS; AEP Technology, Santa Clara, CA, USA).
The photocatalytic activities of the UV-illuminated brackets coated with TiO2 thin films were evaluated by measuring the degradation ratio of MB. The initial concentration of the MB solution was 5 mg/L. The thin film was immersed in the MB solution and the suspension was placed in the dark for 40 minutes before irradiation to ensure the establishment of an adsorption-desorption equilibrium. A 500-W high-pressure Hg lamp with the main wavelength at 365 nm was employed as the UV light irradiation source and positioned 20 cm away from the reactor to trigger a photocatalytic reaction. The degradation ratio was determined using a spectrophotometer (UNICO7200; Unico, Inc., Franksville, WI, USA) and the absorbance at 664 nm was recorded.
Candida albicans (ATCC 10231) and Lactobacillus acidophilus (ATCC 4356) were chosen as standard microbes and the colony counting method was used in our experiment. Separate suspensions of microbes were prepared, each at a concentration of 1.5 × 106 colony-forming units (CFU)/mL. Then, 100 µL of each suspension was added to 3 mL of liquid medium in tubes. The test substrates (coated with TiO2 thin films) and control substrates (undoped substrates) were placed into the tubes, which were incubated at 25℃ and 37℃ for 1 hour under UV-A light irradiation (TLD15W/08, F15T8/BLB; Philips Electronics, Blue Bell, PA, USA). C. albicans was cultured on Sabouraud medium (Guidechem, Shanghai, China); L. acidophilus was cultured on TPY (Tryptone Yeast) medium (Guidechem). This procedure was repeated 5 times. After incubation, 10 µL of each suspension was diluted in 10 mL of sterile water. Then, 10 µL of the dilution was added to cool (55℃) solid medium, which was slightly shaken and then poured into a Petri dish. C. albicans was cultured at 25℃, while L. acidophilus was cultured at 37℃ for 24 hours, after which the numbers of colonies on each plate were determined by a colony counter. This procedure was repeated 3 times.
The antimicrobial tests were repeated 3 times to obtain the most precise outcome. Data were analyzed using SPSS ver. 13.0 software (SPSS Inc., Chicago, IL, USA) and are expressed as the mean ± standard deviation. The antimicrobial activity rate was calculated using the following formula: antimicrobial activity rate (%) = (colony count of the control group - colony count of the test group)/colony count of the control group × 100. Statistically significant differences between groups were determined by a one-way analysis of variance (ANOVA), and the least significant difference (LSD) test was used for multiple comparisons. Values of p < 0.05 were considered statistically significant.
The crystalline microstructures of the TiO2 thin films were characterized using XRD. The results of the XRD analysis (Figures 1 and 2) show that TiO2 thin films annealed at 300℃ were in an amorphous phase, since there is no obvious peak at all. It can be clearly seen that thin films annealed at 500℃ show a weak anatase peak. However, those annealed at 700℃ were characterized by 2 major peaks centered at 2θ = 25.4° and 37.8°, corresponding to the (101) and (200) reflections of the anatase phase, with no sign of the rutile or brookite phase. The crystalline structure changed from the anatase to rutile phase after a 900℃ calcination. The diffraction peak increased slightly with an increase in the number of coatings.
Figures 3 and 4 show SEM images of TiO2 thin films with different numbers of coating layers after a 700℃ calcination. The film morphologies consisted of agglomerates of nano-sized grains or particulates distributed on the substrate surface. It can be clearly seen that the surfaces of all films are covered in fine grains. In addition, the coating layer was found to be continuous and uniformly distributed. The structure was also compact and homogeneous. Moreover, the number of coating layers did not affect the microstructures of thin films calcined at the same temperature. As the calcination temperature increased, the sizes of the nanoparticles increased.
The film thicknesses from different numbers of coatings were measured by surface ellipsometer (films annealed at 700℃ were selected). The results are shown in Table 2. As can be seen, the thickness of the film increased along with an increased number of coating layers. The increase in thickness of the TiO2 thin films showed a linear relationship with the number of coats.
Figure 5 shows the photocatalytic activity of TiO2 thin films annealed at different temperatures under UV light irradiation. From Figure 5A, we can see that the group of films annealed at 300℃ showed no photocatalytic activity. Figure 5B shows the degradation curves of the MB solution degraded by the TiO2 thin film after a 500℃ calcination. After a 180-minute photocatalytic reaction, degradation efficiency increased as the thickness of the film increased. TiO2 thin films with 5 coating layers showed the greatest photocatalytic activity, as 60% of the MB molecules were decomposed within 180 minutes. Figure 5C shows the MB solution degradation curves from a TiO2 thin film annealed at 700℃, and we can see that photocatalytic activity increased as the thickness increased. Films with 5 coating layers had the greatest degradation rate; nearly 90% of the MB solution was decomposed. Figure 5D demonstrates MB solution degradation curves from a TiO2 thin film annealed at 900℃. After a 180-minute photocatalytic reaction, degradation efficiency increased as the thickness increased. It is obvious that the TiO2 thin film with 5 layers exhibited the greatest photocatalytic activity among these test samples, as 20% of the MB molecules were decomposed within 180 minutes.
After comparing the degradation rates of MB solution by TiO2 thin films with 5 coating layers annealed at different temperatures, it was observed that thin films annealed at 700℃ showed the greatest photocatalytic activity, as 90% of the MB molecules were decomposed within 180 minutes. Compared with the others, thin films annealed at 300℃ had the least photocatalytic activity. The photocatalytic activity of the thin film annealed at 500℃ under UV light irradiation showed greater photocatalytic activity than films annealed at 300℃ and 900℃.
Photocatalytic activity is directly proportional to the antibacterial ability of a catalyst. Thus, films annealed at 700℃, which showed the greatest photocatalytic activity, were chosen for the photocatalytic antibacterial test under UV-A light irradiation, and the results are shown in Figure 6. The cell viability of a ceramic substrate on a bare Petri dish in the dark was set as 100%. It can be clearly seen that the thin film with 5 coating layers showed the lowest cell viability at 8% for L. acidophilus and 15% for C. albicans, which exhibited the best antimicrobial performance against both microbes compared with the rest of the samples.
Crystalline structure is a significant factor that affects the photocatalytic performance of TiO2.29 It has been demonstrated that the photocatalytic activity of the anatase structure is greater than that of the rutile structure. Moreover, annealing temperature has the greatest influence on the crystalline structure of TiO2.30 In our study, 4 annealing temperatures were tested to determine optimal calcination conditions. The results of the calcinations are mentioned above. According to the XRD patterns, the films annealed at 500℃ and 700℃ were in anatase form; the greatest photocatalytic activity was observed in thin films annealed at 700℃. Additionally, with an increase in annealing temperature, crystallinity improves. The SEM images also illustrate that with an increase in annealing temperature, the grain size increases.
The antibacterial activity of TiO2 is closely related to its photocatalytic activity. The results show that films annealed at 300℃ had no ability to decompose MB solution. This is mainly because the films were still amorphous in structure after the 300℃ calcination. It can be explained that the poor crystallinity of the films annealed at 300℃ resulted in their low photocatalytic activities. Therefore, the high photocatalytic activities of the 700℃ films can be ascribed to synergetic effects of good crystallization, appropriate phase composition, and a slower recombination rate of photo-generated charge carriers. Thin films annealed at 500℃ showed a certain amount of antibacterial activity. However, compared to thin films annealed at 700℃, the diffraction peak was weaker because of the low calcination temperature. After a 900℃ calcination, TiO2 thin films transformed from anatase to rutile phase, as described in the XRD analysis. However, according to reports,31 the rutile phase of TiO2 has little photocatalytic ability.
An investigation of the influence of different numbers of coating layers showed that prepared thin films with 5 coating layers demonstrated the greatest photocatalytic activity when calcination temperatures were equal. The thicker the film, the greater its photocatalytic activity. It is clear that film thickness influences photocatalytic activity. When the thickness of the film is low, light can pass through the film easily, and the efficiency of the light energy is lower; thus, the photocatalytic efficiency of the film can be enhanced with greater thicknesses.32
The main mechanism for the inactivation of microbes by TiO2 photocatalysis was a hydroxyl radical attack and lipid peroxidation reaction.33 Maness et al.34 proposed a detailed mechanism for the bactericidal effect of the TiO2 photocatalytic reaction. They pointed out that the initial oxidative damage takes place on the cell wall, where the TiO2 photocatalytic surface makes first contact with intact cells. However, cells with damaged cell walls are still viable. After eliminating the protection of the cell wall, oxidative damage occurs on the underlying cytoplasmic membrane. Photocatalytic action progressively increases cell permeability, and subsequently allows the free efflux of intracellular contents that eventually leads to cell death.353637
Özyıldısz38 reported the high antibacterial ability of TiO2-coated ceramic brackets against S. mutans and C. albicans under UV-A irradiation. Shah et al.39 reported that TiO2-modified orthodontic brackets showed a statistically significant decrease in the survival rate of lactobacilli under UV-A light irradiation. Our study showed similar results to the above reports.
Several studies report that transparent thin films can be achieved by using the sol-gel method.40414243 Moreover, the transmittance rate of the thin film could exceed 80%.44 Our main purpose was to prepare TiO2 thin films on ceramic brackets without affecting the esthetics of the brackets, and to improve antimicrobial activity. In our study, transparent thin films were also prepared by the sol-gel method and demonstrated similar results to previous studies.
1) TiO2 thin films were successfully prepared on the surfaces of ceramic bracket substrates using the solgel dip coating method. Films with 5 coating layers annealed at 500℃ and 700℃ exhibited anatase phase structures. An increase in temperature led to an increase in crystallinity.
2) TiO2 thin films annealed at 700℃ showed higher photocatalytic activity with increased thickness. TiO2 thin film brackets with 5 coating layers annealed at 700℃ showed the greatest photocatalytic activity in the decomposition of MB solution under UV light irradiation.
3) Additionally, the films with 5 coating layers and annealed at 700℃ exhibited the greatest antibacterial activity against L. acidophilus and C. albicans under UV-A light irradiation. Therefore, TiO2 thin films can be used for further study and continuing development, and we offer a theoretical basis for their practical use.
Figures and Tables
Figure 1
X-ray diffractometer patterns of TiO2 thin films with different numbers of coating layers and annealed at different temperatures. A, TiO2 thin films with 1-5 coating layers annealed at 300℃; B, TiO2 thin films with 1-5 coating layers annealed at 500℃; C, TiO2 thin films with 1-5 coating layers annealed at 700℃; D, TiO2 thin films with 1-5 coating layers annealed at 900℃.
a.u., Arbitrary unit.
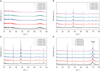
Figure 2
X-ray diffractometer patterns of TiO2 thin films with 5 coating layers annealed at 4 different temperatures.
a.u., Arbitrary unit.
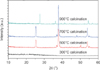
Figure 3
Scanning electron microscopy images of TiO2 thin films annealed at 700℃. A, TiO2 thin film with 1 coating layer; B, TiO2 thin film with 2 coating layers; C, TiO2 thin film with 3 coating layers; D, TiO2 thin film with 4 coating layers; E, TiO2 thin film with 5 coating layers.
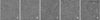
Figure 4
Scanning electron microscopy images of TiO2 thin films with 5 coating layers annealed at 4 different temperatures. A, Thin film annealed at 300℃; B, thin film annealed at 500℃; C, thin film annealed at 700℃; D, thin film annealed at 900℃.
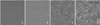
Figure 5
Photocatalytic degradation of methylene blue solution by TiO2 thin films under ultraviolet light irradiation. A, TiO2 thin films with 1-5 coating layers annealed at 300℃; B, TiO2 thin films with 1-5 coating layers annealed at 500℃; C, TiO2 thin films with 1-5 coating layers annealed at 700℃; D, TiO2 thin films with 1-5 coating layers annealed at 900℃.
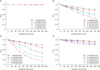
ACKNOWLEDGEMENTS
We sincerely thank Yuren Li from the School of Physical Science and Technology, Lanzhou University, Lanzhou City, P. R. China for supplying and providing assistance with devices used in this study. We appreciate the valuable comments from other members of our laboratories.
Notes
References
1. Bishara SE, Fehr DE. Ceramic brackets: something old, something new, a review. Semin Orthod. 1997; 3:178–188.


2. Eliades T, Lekka M, Eliades G, Brantley WA. Surface characterization of ceramic brackets: a multitechnique approach. Am J Orthod Dentofacial Orthop. 1994; 105:10–18.


3. Eissaa OE, El-Shourbagy EM, Ghobashy SA. In vivo effect of a fluoride releasing adhesive on inhibition of enamel demineralization around orthodontic brackets. Tanta Dent J. 2013; 10:86–96.


4. Tufekci E, Dixon JS, Gunsolley JC, Lindauer SJ. Prevalence of white spot lesions during orthodontic treatment with fixed appliances. Angle Orthod. 2011; 81:206–210.


5. Richter AE, Arruda AO, Peters MC, Sohn W. Incidence of caries lesions among patients treated with comprehensive orthodontics. Am J Orthod Dentofacial Orthop. 2011; 139:657–664.


6. Gorelick L, Geiger AM, Gwinnett AJ. Incidence of white spot formation after bonding and banding. Am J Orthod. 1982; 81:93–98.


7. Chatterjee R, Kleinberg I. Effect of orthodontic band placement on the chemical composition of human incisor tooth plaque. Arch Oral Biol. 1979; 24:97–100.


8. Øgaard B. White spot lesions during orthodontic treatment: mechanisms and fluoride preventive aspects. Semin Orthod. 2008; 14:183–193.


9. Scheie AA, Arneberg P, Krogstad O. Effect of orthodontic treatment on prevalence of Streptococcus mutans in plaque and saliva. Scand J Dent Res. 1984; 92:211–217.


10. Mattsson A, Lejon C, Bakardjieva S, Štengl V, Österlund L. Characterisation, phase stability and surface chemical properties of photocatalytic active Zr and Y co-doped anatase TiO2 nanoparticles. J Solid State Chem. 2013; 199:212–223.


11. Cui C, Liu H, Li Y, Sun J, Wang R, Liu S, et al. Fabrication and biocompatibility of nano-TiO2/titanium alloys biomaterials. Mater Lett. 2005; 59:3144–3148.


12. Madhan Kumar A, Rajendran N. Electrochemical aspects and in vitro biocompatibility of polypyrrole/TiO2 ceramic nanocomposite coatings on 316L SS for orthopedic implants. Ceram Int. 2013; 39:5639–5650.


13. Poma AM, Di Bucchianico S, Galano A, Santucci S. Biocompatibility evaluation of TiO2 nanoparticles and thin films by means of the murine macrophages RAW 264.7 cell line. J Biotechnol. 2010; 150:Suppl. 466.


14. Nejand BA, Sanjabi S, Ahmadi V. Sputter deposition of high transparent TiO2-xNx/TiO2/ZnO layers on glass for development of photocatalytic self-cleaning application. Appl Surf Sci. 2011; 257:10434–10442.


15. Fujishima A, Honda K. Electrochemical photolysis of water at a semiconductor electrode. Nature. 1972; 238:37–38.


16. Chun MJ, Shim E, Kho EH, Park KJ, Jung J, Kim JM, et al. Surface modification of orthodontic wires with photocatalytic titanium oxide for its antiadherent and antibacterial properties. Angle Orthod. 2007; 77:483–488.


17. Wang H, Tang B, Li X, Ma Y. Antibacterial properties and corrosion resistance of nitrogen-doped TiO2 coatings on stainless steel. J Mater Sci Technol. 2011; 27:309–316.


18. Choi JY, Kim KH, Choy KC, Oh KT, Kim KN. Photocatalytic antibacterial effect of TiO(2) film formed on Ti and TiAg exposed to Lactobacillus acidophilus. J Biomed Mater Res B Appl Biomater. 2007; 80:353–359.
19. Zhou L, Deng J, Zhao Y, Liu W, An L, Chen F. Preparation and characterization of N-I codoped nanocrystal anatase TiO2 with enhanced photocatalytic activity under visible-light irradiation. Mater Chem Phys. 2009; 117:522–527.


20. Barrocas B, Monteiro OC, Jorge MEM, Sério S. Photocatalytic activity and reusability study of nanocrystalline TiO2 films prepared by sputtering technique. Appl Surf Sci. 2013; 264:111–116.


21. Lee H, Park SH, Kim SJ, Kim BH, Yoon HS, Kim JS, et al. The effect of combined processes for advanced oxidation of organic dye using CVD TiO2 film photo-catalysts. Prog Org Coat. 2012; 74:758–763.


22. Tavares CJ, Marques SM, Rebouta L, Lanceros-Méndez S, Sencadas V, Costa CM, et al. PVD-Grown photocatalytic TiO2 thin films on PVDF substrates for sensors and actuators applications. Thin Solid Film. 2008; 517:1161–1166.


23. Balasubramanian K, Han XF, Guenther KH. Comparative study of titanium dioxide thin films produced by electron-beam evaporation and by reactive low-voltage ion plating. Appl Opt. 1993; 32:5594–5600.


24. Akpan UG, Hameed BH. The advancements in solgel method of doped-TiO2 photocatalysts. Appl Catal A Gen. 2010; 375:1–11.


25. Fu T, Wen CS, Lu J, Zhou YM, Ma SG, Dong BH, et al. Sol-gel derived TiO2 coating on plasma nitrided 316L stainless steel. Vacuum. 2012; 86:1402–1407.


26. Nakaruk A, Lin CY, Perera DS, Sorrell CC. Effect of annealing temperature on titania thin films prepared by spin coating. J Sol-Gel Sci Technol. 2010; 55:328–334.


27. Pomoni K, Vomvas A, Trapalis C. Electrical conductivity and photoconductivity studies of TiO2 sol-gel thin films and the effect of N-doping. J Non-Cryst Solid. 2008; 354:4448–4457.


28. Cao S, Liu B, Fan L, Yue Z, Liu B, Cao B. Highly antibacterial activity of N-doped TiO2 thin films coated on stainless steel brackets under visible light irradiation. Appl Surf Sci. 2014; 309:119–127.


29. Singh P, Kaur D. Room temperature growth of nanocrystalline anatase TiO2 thin films by dc magnetron sputtering. Phys B Condens Matter. 2010; 405:1258–1266.


30. Hou YQ, Zhuang DM, Zhang G, Zhao M, Wu MS. Influence of annealing temperature on the properties of titanium oxide thin film. Appl Surf Sci. 2003; 218:98–106.


31. Kaplan R, Erjavec B, Dražić G, Grdadolnik J, Pintar A. Simple synthesis of anatase/rutile/brookite TiO2 nanocomposite with superior mineralization potential for photocatalytic degradation of water pollutants. Appl Catal B Environ. 2016; 181:465–474.


32. Chen Y, Dionysiou DD. Correlation of structural properties and film thickness to photocatalytic activity of thick TiO2 films coated on stainless steel. Appl Catal B Environ. 2006; 69:24–33.


33. Wang X, Hou X, Luan W, Li D, Yao K. The antibacterial and hydrophilic properties of silver-doped TiO2 thin films using sol-gel method. Appl Surf Sci. 2012; 258:8241–8246.


34. Maness PC, Smolinski S, Blake DM, Huang Z, Wolfrum EJ, Jacoby WA. Bactericidal activity of photocatalytic TiO(2) reaction: toward an understanding of its killing mechanism. Appl Environ Microbiol. 1999; 65:4094–4098.


35. Chen Y, Wong RW, Seneviratne CJ, Hägg U, McGrath C, Samaranayake LP, et al. The antimicrobial efficacy of Fructus mume extract on orthodontic bracket: a monospecies-biofilm model study in vitro. Arch Oral Biol. 2011; 56:16–21.


36. Karunakaran C, Rajeswari V, Gomathisankar P. Enhanced photocatalytic and antibacterial activities of sol-gel synthesized ZnO and Ag-ZnO. Mater Sci Semicond Process. 2011; 14:133–138.


37. Sayilkan F, Asiltürk M, Kiraz N, Burunkaya E, Arpaç E, Sayilkan H. Photocatalytic antibacterial performance of Sn(4+)-doped TiO(2) thin films on glass substrate. J Hazard Mater. 2009; 162:1309–1316.


38. Özyıldız F, Güden M, Uzel A, Karaboz I, Akil O, Bulut H. Antimicrobial activity of TiO2-coated orthodontic ceramic brackets against Streptococcus mutans and Candida albicans. Biotechnol Bioprocess Eng. 2010; 15:680–685.


39. Shah AG, Shetty PC, Ramachandra CS, Bhat NS, Laxmikanth SM. In vitro assessment of photocatalytic titanium oxide surface modified stainless steel orthodontic brackets for antiadherent and antibacterial properties against Lactobacillus acidophilus. Angle Orthod. 2011; 81:1028–1035.


40. Calderon-Moreno JM, Preda S, Predoana L, Zaharescu M, Anastasescu M, Nicolescu M, et al. Effect of polyethylene glycol on porous transparent TiO2 films prepared by sol-gel method. Ceram Int. 2014; 40:2209–2220.


41. Malengreaux CM, Timmermans A, Pirard SL, Lambert SD, Pirard JP, Poelman D, et al. Optimized deposition of TiO2 thin films produced by a non-aqueous sol-gel method and quantification of their photocatalytic activity. Chem Eng J. 2012; 195-196:347–358.


42. Sharma SK, Vishwas M, Rao KN, Mohan S, Reddy DS, Gowda KVA. Structural and optical investigations of TiO2 films deposited on transparent substrates by sol-gel technique. J Alloy Compd. 2009; 471:244–247.

