Abstract
Objective
In our previous study, glass-fiber-reinforced plastics (GFRPs) made from polycarbonate and glass fibers were prepared for esthetic orthodontic wires using pultrusion. These laboratory GFRP wires are more transparent than the commercially available nickel-titanium wire; however, an investigation of the color stability of GFRP during orthodontic treatment is needed. Accordingly, in the present study, the color stability of GFRP was assessed using colorimetry.
Methods
Preparation of GFRP esthetic round wires (diameter: 0.45 mm [0.018 inch]) using pultrusion was described previously. Here, to investigate how the diameter of fiber reinforcement affects color stability, GFRPs were prepared by incorporating either 13-µm (GFRP-13) or 7-µm glass (GFRP-7) fibers. The color changes of GFRPs after 24 h, and following 1, 2, and 4 weeks of coffee immersion at 37℃, were measured by colorimetry. We evaluated the color stability of GFRPs by two evaluating units: the color difference (ΔE*) and National Bureau of Standards (NBS).
Results
After immersion, both GFRPs showed almost no visible color change. According to the colorimetry measurements, the ΔE* values of GFRP-13 and GFRP-7 were 0.73-1.16, and 0.62-1.10, respectively. In accordance with NBS units, both GFRPs showed "slight" color changes. As a result, there were no significant differences in the ΔE* values or NBS units for GFRP-13 or GFRP-7. Moreover, for both GFRPs, no significant differences were observed in any of the immersion periods.
Orthodontic wires are widely used as part of orthodontic appliances throughout treatment. These wires are made from metal alloys such as stainless steel (SS), cobalt-chromium-nickel (Co-Cr-Ni), β-titanium (β-Ti), and nickel-titanium (Ni-Ti) alloys.1,2 The main advantage of using these metal alloys in orthodontic wires is their superior mechanical properties compared to other materials such as polymers and ceramics. However, one disadvantage of these metallic wires is the metal's opaque appearance, which deters many patients from orthodontic treatment.3 To address this issue, many types of esthetic brackets have been developed for orthodontic appliances.4,5 These brackets have been made from alumina, zirconia, polycarbonates, and their composites.6
Recently, polymer-coated alloy wires have been developed as esthetic orthodontic wires,3,7,8 whereby metal alloys were coated with polymers such as epoxy resin and polyethylene. However, there is the potential for exfoliation of the coated layer when using these materials.7,8 For example, da Silva et al.7 investigated the coating stability of four esthetic coated archwires. The authors reported that these coated wires had low esthetic value because their coating lacked durability after oral exposure. These studies highlight the need for non-metallic wires without a coated layer.
The transparent or translucent appearance of glass-fiber-reinforced plastic (GFRP) wires is considered attractive, and is more likely to be sustained during orthodontic treatment. GFRP has been used previously in the development of dental appliances such as crowns, bridges, frameworks for fixed partial dentures, and dental posts in both research and clinical experiments.9 In our previous study, GFRPs composed of a polycarbonate matrix with glass-fiber reinforcement were fabricated for esthetic orthodontic wires using the pultrusion method.10 The GFRP wires had similar flexural properties to those of commercially available Ni-Ti wires. Furthermore, these wires were more transparent than the available Ni-Ti wires (Figure 1); however, investigations regarding the color stability of these GFRP wires during orthodontic treatment is needed.
To date, numerous studies have focused on the color stability of esthetic orthodontic appliances such as brackets11,12,13 and ligatures.14,15 For example, Filho et al.12 evaluated the color stability of esthetic brackets made from ceramic and polymeric materials after staining them in two solutions containing coffee and black tea. Likewise, Martins da Silva et al.14 assessed the color stability of esthetic elastomeric ligatures after exposure to the oral environment. Despite the well-known color stability of orthodontic appliances such as brackets and ligatures, the color stability of such esthetic orthodontic wires is poorly understood. Hence, esthetic orthodontic wires are only commercially available in a limited number of materials.16 It is also difficult to measure the color stability of esthetic orthodontic wires because of their shape and dimensions.17
The purpose of this study was to prepare esthetic orthodontic wires from GFRP composed of glass fiber and a polycarbonate matrix using a pultrusion method. Furthermore, the color stability of these GFRP esthetic wires was assessed by a devised colorimetric measurement method as described later.
GFRP wires were fabricated using a pultrusion technique described in a previous study.10 A polycarbonate (H4000; Mitsubishi Engineering-Plastics Corp., Tokyo, Japan) was used as the thermoplastic matrix because of its low viscosity, which allowed us to fabricate GFRP wires with diameters that were as small as those in the currently available orthodontic wires. An E-glass fiber filament (Nittobo Co., Fukushima, Japan) was used as a unidirectional reinforcement for the polycarbonate matrix. To investigate how fiber diameter affects the properties of GFRP, we prepared GFRPs with two different E-glass fiber diameters: 13 µm (GFRP-13) and 7 µm (GFRP-7). The volume fraction of glass fiber in the GFRP was approximately 0.3. From the pultruded samples, we cut round GFRP wires, each with a diameter of 0.45 mm (0.018 inch) and a length of 36 cm.
The colorimetric measurement of the as-fabricated GFRP wires with such small diameters was impossible; samples with a total width of at least 3 mm are required so that the color can be properly measured by colorimetry, as described later. Accordingly, in the present study, a slight modification to the technique described by da Silva et al.16 was used to prepare a GFRP sample by tightly arranging seven wire segments (11 mm in length, 0.45 mm in diameter) by fixing both edges with commercially available flowable resin (Filtek™ Flow, 3M ESPE, Saint Paul, MN, USA), as shown in Figure 2A.
GFRP samples were immersed for 4 weeks in 20 mL of coffee (NESCAFE Excella®; Nestlé Japan Ltd., Hyogo, Japan), which was used as a staining solution, in a Teflon-sealed polystyrene bottle at 37℃, with the coffee solution refreshed weekly. After staining, the samples were washed with distilled water and dried with paper towels. Color changes after 24 h, and following 1, 2, and 4 weeks of immersion, were measured by a colorimeter (ShadeEye NCC; Shofu Inc., Kyoto, Japan) using a shade tab (A3, SOLARE; GC Corp., Tokyo, Japan), as shown in Figure 2B. This device contains a pulsed xenon lamp as an optical light source and a three-component silicon photocell as the optical sensor.18 The colorimetric measurements were performed by contacting the measurement tip of the optical sensor to the GFRP sample using the shade tabs as a reference. The measurement values were obtained as the average of 10 samples (n = 10) with each sample measured three times.
The color parameters were expressed using the Commission Internationale de l'Eclairage (CIE) L*a*b* color space system,1,19 relative to an illuminant standard, D65. In this three-dimensional color space, the three axes are L*, a*, and b*. The L* value is a measure of the lightness of an object and is quantified on a scale such that a perfect black has an L* value of 0 and a perfect reflecting diffuser has an L* value of 100. The a* value is a measure of the redness (+a*) or greenness (-a*) of an object, while the b* value is a measure of the yellowness (+b*) or blueness (-b*) of an object.
The color difference (ΔE*) before and after immersion was calculated according to the equation1:
Figure 3 shows photographs of the GFRP wires before and after 4 weeks of immersion in the coffee solution. After immersion, the GFRP wires showed almost no color change upon visual inspection. Table 2 shows the color differences reported using the ΔE* and NBS units for the GFRP wires after each immersion period. The ΔE* values at 24 h and 1, 2, and 4 weeks after immersion for GFRP-13 and GFRP-7 ranged from 0.73 to 1.16 and from 0.62 to 1.10, respectively. There were no significant differences in ΔE* among any of the measured values for either of the GFRP wires (p > 0.05). The NBS units at 24 h and 1, 2, and 4 weeks after immersion for GFRP-13 and GFRP-7 ranged from 0.67 to 1.06 and from 0.57 to 1.01, respectively; our results showed that all of the samples exhibited color changes according to the NBS values, which ranged from 0.57 to 1.06. As shown in Table 1, the color change values after immersion for 24 h and 1, 2, and 4 weeks were < 1.5 for both GFRPs, and only "slight" color changes were observed according to the NBS units. Furthermore, there were no significant differences in the color-change values for the GFRPs in any of the immersion periods (p > 0.05).
GFRP wires are much more esthetically pleasing than wires made from conventional orthodontic alloys because of their transparency. It is difficult for the naked human eye to discern the small-order color changes. Additionally, there are only a few experimental studies on the color stability of esthetic wires because of the small geometry (i.e., shape and dimension) of orthodontic wires and the currently available GFRP wires. Here, by applying the same methods as those used by other researchers,16 we devised a measurement method for determining the color stability of GFRP wires, i.e., colorimetric measurements were performed on GFRP samples prepared by arranging seven wire segments together (Figure 1A). Consequently, we were able to evaluate the color stability of GFRP wires according to two evaluation units (ΔE* and NBS).
First, color differences were assessed by determining the ΔE* values of the GFRPs after immersion in coffee using the CIE Lab system. The advantage of this system is that color differences can be expressed in units that may be related to visual perception. There are numerous studies on the threshold levels for the color differences of orthodontic appliances that are visually perceptible or clinically acceptable, and these threshold levels have been assessed using various standards.12,13,15,16,17,20 For example, Faltermeier et al.13 evaluated the color stability of four different polymer brackets after exposure to ultraviolet light or food dyes for 74 h. The authors applied a threshold level of 3.3 for ΔE*, which pertains to color differences that are visually perceptible and clinically unacceptable. They reported that newly developed plastic brackets consisting of polyoxymethylene may have clinically unacceptable color stability in the long term. According to other researchers, a color change of 3.7 as measured by ΔE* has been suggested as clinically unacceptable.12,17 Moreover, Douglas et al.21 reported that the predicted value at which 50% of dentists could perceive a color difference was 2.6 ΔE* units. In any case, all of the experimental values obtained for the GFRPs in the present study were lower than 1.5 ΔE* units, ranging from 0.62 to 1.16 (Table 2). Although evaluating the color changes of orthodontic appliances according to ΔE* units has not yet been clearly defined, the relatively low ΔE* values for the present GFRPs suggest that discoloration will not occur during orthodontic treatment.
In addition to using the ΔE* values, the degree of color differences in GFRPs were assessed in NBS units. According to the critical levels for color differences, both GFRPs underwent "slight" color changes after 4 weeks of immersion in the coffee solution, with NBS units ranging from 0.57 to 1.06 (Table 1). Results obtained in NBS and ΔE* units indicated that both of the GFRPs fabricated in our laboratory showed high color stability. Conversely, it has been reported that commercially available GFRPs exhibit "extremely marked" ("much") color changes, as measured in NBS units, after immersion in a coffee solution for 3 weeks at 37℃.16 The difference in color stability may be explained by differences in the matrix components of the GFRPs. In the present study, the GFRP orthodontic wires were fabricated using polycarbonate as the matrix and glass fiber as reinforcement via pultrusion. In contrast, commercially available GFRPs are typically composed of polymethylmethacrylate (PMMA) and glass fiber.22 Polycarbonates are generally recognized as low sorption materials23 and are widely used in orthodontic appliances such as esthetic brackets and clear retainers.6,24 In particular, Hamanaka et al.23 reported that polycarbonates have low sorption compared with PMMA, although this research was focused on denture base resins. Additionally, in some articles pertaining to the color stability of brackets, polycarbonate-based brackets showed lower color changes among esthetic plastic brackets.17 There is also a known correlation between the color stability and water sorption of a resin.25,26 Therefore, it is thought that the lower sorption and color changes exhibited by our laboratory-fabricated GFRP compared with commercially available GFRPs are owed to the polycarbonate matrix resin, which keeps the water sorption suitably low for use in orthodontic wire materials. Furthermore, it is worth noting that the flowable resins that we used to prepare the samples showed visible discoloration despite the GFRP wires showing no discoloration during the immersion period (Figure 3). Flowable resins are typically composed of dimethacrylates such as bisphenol-A-glycidyl methacrylate and triethylene glycol dimethacrylate (TEGDMA).27 It has been reported that materials containing TEGDMA show higher discoloration values because of its hydrophilic property.28 It is therefore speculated that using polycarbonate as the matrix of our fabricated GFRP is advantageous because of its higher color stability compared to both TEGDMA and PMMA. However, further experiments are needed to investigate the relationship between color stability and surface roughness in the oral environment because color changes of intra-orally exposed polymeric materials may be an effect of changes in surface roughness, which are caused by the mechanical effects of brushing and eating solid food.
In conclusion, the GFRPs fabricated in our study may be satisfactory for clinical duration of orthodontic treatment in terms of color stability and are thus expected to be attractive materials for esthetic orthodontic wires.
This study investigated the color stability of esthetic GFRP wires composed of polycarbonate and glass fibers prepared by pultrusion and the following conclusions were drawn.
First, following immersion, the color differences indicated by the ΔE* values of both GFRP-13 and GFRP-7 were lower than the ΔE* value of 1.5. In accordance with NBS units, both GFRPs showed "slight" color changes. This indicates that the color changes in the GFRPs were minimal during the 4 weeks of coffee immersion.
Second, there were no significant differences in the ΔE* values or NBS units for either GFRP-13 or GFRP-7. Moreover, there were no significant differences for either of the GFRPs for any of the immersion periods.
Within the limitations of this investigation, the results indicate that the esthetic properties of GFRPs may remain stable during orthodontic treatment.
Notes
References
1. O'Brien WJ. Orthodontic wire. In : O'Brien WJ, editor. Dental materials and their selection. Chicago: Quintessence Pub Co;2008.
2. Eliades T. Dental materials in orthodontics. In : Graber LW, Vanarsdall RL, Vig KWL, editors. Orthodontics: current principles and techniques. 5th ed. Philadelphia, PA: Elsevier/Mosby;2012. p. 1023–1037.
3. Elayyan F, Silikas N, Bearn D. Ex vivo surface and mechanical properties of coated orthodontic archwires. Eur J Orthod. 2008; 30:661–667. PMID: 19011166.


4. Yu B, Lee YK. Aesthetic colour performance of plastic and ceramic brackets-an in vitro study. J Orthod. 2011; 38:167–174. PMID: 21875990.
5. Lee YK. Colour and translucency of tooth-coloured orthodontic brackets. Eur J Orthod. 2008; 30:205–210. PMID: 18390838.


6. Eliades T, Eliades G, Brantly WA. Orthodontic brackets. In : Brantly WA, Eliades T, editors. Orthodontic materials. New York: Thieme;2001. p. 143–172.
7. da Silva DL, Mattos CT, Simão RA, de Oliveira Ruellas AC. Coating stability and surface characteristics of esthetic orthodontic coated archwires. Angle Orthod. 2013; 83:994–1001. PMID: 23650959.


8. Zegan G, Sodor A, Munteanu C. Surface characteristics of retrieved coated and nickel-titanium orthodontic archwires. Rom J Morphol Embryol. 2012; 53:935–939. PMID: 23303016.
9. Fujihara K, Teo K, Gopal R, Loh PL, Ganesh VK, Ramakrishna S, et al. Fibrous composite materials in dentistry and orthopaedics: Review and applications. Compos Sci Technol. 2004; 64:775–788.


10. Tanimoto Y, Inami T, Yamaguchi M, Nishiyama N, Kasai K. Preparation, mechanical, and in vitro properties of glass fiber-reinforced polycarbonate composites for orthodontic application. J Biomed Mater Res B Appl Biomater. 2014; doi:10.1002/jbm.b.33245 [Epub ahead of print].
11. de Mendonca MR, Fabre AF, Goiatto MC, Cuoghi OA, Verri ACG. Spectrophotometric evaluation of color changes of esthetic brackets stored in potentially staining solutions. RPG Rev pós-gra. 2011; 18:20–27.
12. Filho HL, Maia LH, Araújo MV, Eliast CN, Ruellas AC. Colour stability of aesthetic brackets: ceramic and plastic. Aust Orthod J. 2013; 29:13–20. PMID: 23785933.
13. Faltermeier A, Behr M, Müssig D. In vitro colour stability of aesthetic brackets. Eur J Orthod. 2007; 29:354–358. PMID: 17702794.


14. Martins da Silva AV, de Mattos GV, Kato CM, Normando D. In vivo color changes of esthetic orthodontic ligatures. Dental Press J Orthod. 2012; 17:76–80.
15. Kim SH, Lee YK. Measurement of discolouration of orthodontic elastomeric modules with a digital camera. Eur J Orthod. 2009; 31:556–562. PMID: 19474228.


16. da Silva DL, Mattos CT, de Araújo MV, de Oliveira Ruellas AC. Color stability and fluorescence of different orthodontic esthetic archwires. Angle Orthod. 2013; 83:127–132. PMID: 22591261.


17. Lee YK. Changes in the reflected and transmitted color of esthetic brackets after thermal cycling. Am J Orthod Dentofacial Orthop. 2008; 133:641.e1–641.e6. PMID: 18456136.


18. Yamanel K, Caglar A, Özcan M, Gulsah K, Bagis B. Assessment of color parameters of composite resin shade guides using digital imaging versus colorimeter. J Esthet Restor Dent. 2010; 22:379–388. PMID: 21126293.


19. Joiner A. Tooth colour: a review of the literature. J Dent. 2004; 32(Suppl 1):3–12. PMID: 14738829.


20. Faltermeier J, Simon P, Reicheneder C, Proff P, Faltermeier A. The influence of electron beam irradiation on colour stability and hardness of aesthetic brackets. Eur J Orthod. 2012; 34:427–431. PMID: 21502379.


21. Douglas RD, Steinhauer TJ, Wee AG. Intraoral determination of the tolerance of dentists for perceptibility and acceptability of shade mismatch. J Prosthet Dent. 2007; 97:200–208. PMID: 17499089.


22. Scabell AL, Elias CN, Fernandes DJ, Quintao CCA. Failure of fiber reinforced composite archwires. J Res Practice Dent. 2013; doi: 10.5171/2013.304484 [Epub ahead of print].


23. Hamanaka I, Iwamoto M, Lassila L, Vallittu P, Shimizu H, Takahashi Y. Influence of water sorption on mechanical properties of injection-molded thermoplastic denture base resins. Acta Odontol Scand. 2014; 72:859–865. PMID: 24850507.


24. Ardeshna AP. Clinical evaluation of fiber-reinforced-plastic bonded orthodontic retainers. Am J Orthod Dentofacial Orthop. 2011; 139:761–767. PMID: 21640882.


25. Shiozawa M, Takahashi H, Asakawa Y, Iwasaki N. Color stability of adhesive resin cements after immersion in coffee. Clin Oral Investig. 2014; doi: 10.1007/s00784-014-1272-8 [Epub ahead of print].


26. Dietschi D, Campanile G, Holz J, Meyer JM. Comparison of the color stability of ten new-generation composites: an in vitro study. Dent Mater. 1994; 10:353–362. PMID: 7498599.


27. Lee IB, Min SH, Kim SY, Ferracane J. Slumping tendency and rheological properties of flowable composites. Dent Mater. 2010; 26:443–448. PMID: 20170946.


28. Ertaş E, Güler AU, Yücel AC, Köprülü H, Güler E. Color stability of resin composites after immersion in different drinks. Dent Mater J. 2006; 25:371–376. PMID: 16916243.
Figure 1
Photograph of esthetic glass-fiber-reinforced plastic (GFRP) orthodontic archwires. Laboratory GFRP archwires and commercially available polycarbonate brackets fixed to the teeth in a dental model.
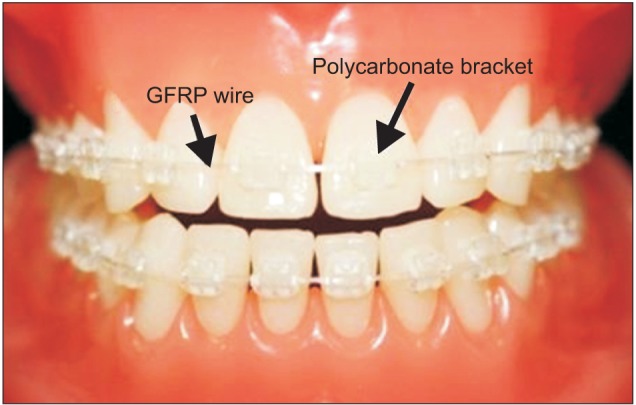
Figure 2
Experimental set-up for color-stability measurement. A, The glass-fiber-reinforced plastic (GFRP) sample devised for colorimetric measurement. This sample was prepared by arranging seven wire segments. Flowable resin was used to fix the wire segments. B, Photograph of the sample and device set-up during measurement. The elastic tip of the instrument was in contact with the middle of the sample.
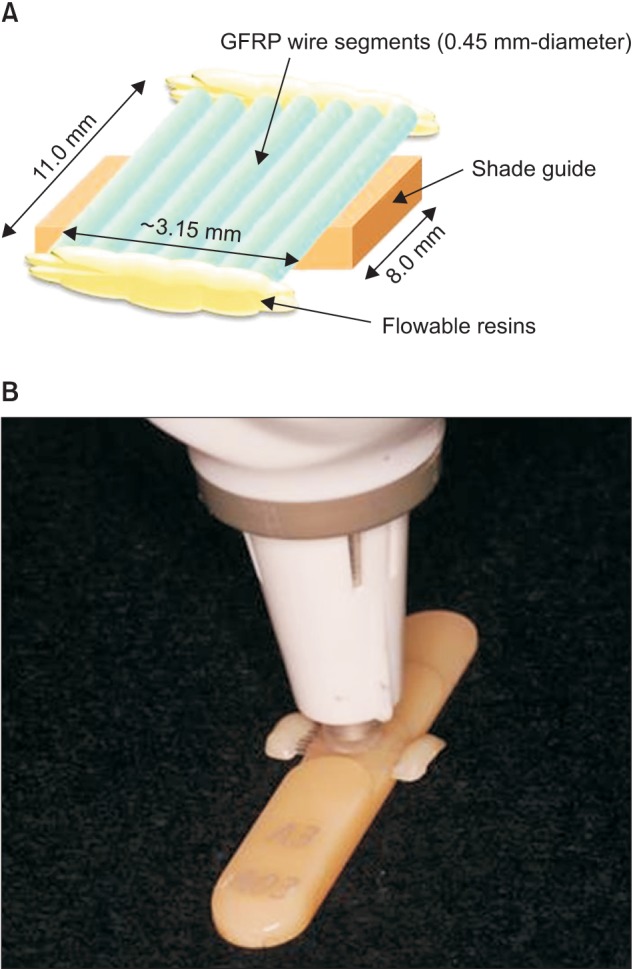
Figure 3
Photograph of the glass-fiber-reinforced plastic (GFRP) wires before and after immersion in the coffee solution for 4 weeks. A, GFRP-13 before immersion. B, GFRP-7 before immersion. C, GFRP-13 after immersion. D, GFRP-7 after immersion. As indicated by the white arrows, the flowable resin that was used to fix the GFRP wires showed discoloration after immersion, while the GFRP wires themselves showed no discoloration.
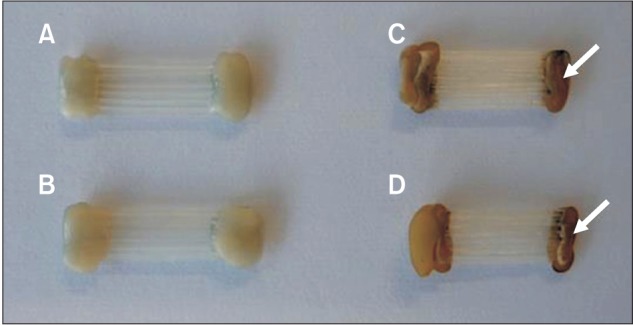