Abstract
Objective
This study aimed to compare the frictional force (FR) in self-ligating brackets among different bracket-archwire angles, bracket materials, and archwire types.
Methods
Passive and active metal self-ligating brackets and active ceramic self-ligating brackets were included as experimental groups, while conventional twin metal brackets served as a control group. All brackets were maxillary premolar brackets with 0.022 inch [in] slots and a -7° torque. The orthodontic wires used included 0.018 round and 0.019 × 0.025 in rectangular stainless steel wires. The FR was measured at 0°, 5°, and 10° angulations as the wire was drawn through the bracket slots after attaching brackets from each group to the universal testing machine. Static and kinetic FRs were also measured.
Results
The passive self-ligating brackets generated a lower FR than all the other brackets. Static and kinetic FRs generally increased with an increase in the bracket-archwire angulation, and the rectangular wire caused significantly higher static and kinetic FRs than the round wire (p < 0.001). The metal passive self-ligating brackets exhibited the lowest static FR at the 0° angulation and a lower increase in static and kinetic FRs with an increase in bracket-archwire angulation than the other brackets, while the conventional twin brackets showed a greater increase than all three experimental brackets.
Tooth movement during orthodontic treatment is accomplished by the delivery of orthodontic forces through brackets. A frictional force (FR), defined as the resistant force against movement when two contacting objects are moving, is generated between the brackets and archwires during this process. FR can be divided into static and kinetic FRs. The former is the minimum amount of force required for the initial movement of a static object, while the latter is the force required to maintain the movement of two objects at a certain velocity.
Stoner1 reported that FRs generated during tooth movement considerably decrease orthodontic forces. Therefore, an orthodontic force that dissipates as FR should be considered during clinical treatment.2 Although high FRs can offset the force delivered to the teeth during orthodontic treatment and cause anchor loss and debonding of brackets, appropriate control of FRs can improve the treatment effects and decrease the treatment duration. The material constituting the brackets and archwires, surface conditions of arch wires, size of bracket slots, cross-section and torque of archwires, ligation method, interbracket distance, and salivary and intraoral conditions are factors that can affect FR.3,4,5,6,7 According to previous studies on the effects of brackets and archwires on FR, teeth move through repeated movements that cause them to tilt and become upright.8 Peterson et al.9 adjusted the angulation between the bracket and archwire to 0°-10° to evaluate FRs.
Meanwhile, Thorstenson and Kusy10 and Kusy and Whitley11 studied the critical slope angles at which FRs tended to increase. After examining various combinations of archwires and brackets, they found the critical slope angle to be approximately 3.7°, and FRs increased sharply at angles larger than 3.7°. Furthermore, the increase in FRs with an increase in the critical slope angle is affected by the physical properties of the materials of the appliances.11 The type of orthodontic wire can reportedly affect the increase in FR as well as the rate of increase, with nitinol and beta-titanium arch wires showing a higher FR than stainless steel (SS) archwires. Furthermore, there are reports that FRs increase with an increase in the thickness of orthodontic wires and are higher with rectangular wires than with round wires.7 Cacciafesta et al.12 reported that metal brackets exhibited a lower FR than ceramic or resin brackets after examining differences in FRs according to bracket materials. Ceramic brackets exhibit higher FRs than metal brackets, which have softer surfaces that are easily polished with archwire binding. FRs also highly depend on ligation method, among other factors. Generally, archwires are inserted into brackets and held with elastic rings or ligature wires, but self-ligating brackets have special hinge caps, eliminating the need for ligature wires. Self-ligating brackets reportedly facilitate effective tooth movement while shortening the total treatment duration because of decreased FRs.13 A decrease in FRs as a result of different ligation methods can decrease the treatment duration, thus providing an advantage during the treatment of orthodontic extraction cases. Furthermore, the surfaces of self-ligating brackets are generally smooth; therefore, they provide more comfort and allow better oral hygiene control to the patient.14 Previous studies on the FRs of metal self-ligating brackets have reported varying results, possibly because of different experimental conditions and limitations in mechanical reproduction.15 However, there are few comparative studies including ceramic self-ligating brackets. Therefore, in this study, the authors evaluated and compared FRs among different bracket-archwire angles and different bracket materials.
Four types of brackets were used in this experiment. The experimental groups included passive metal self-ligating brackets (Damon3 MX; Ormco, Orange, CA, USA), active metal self-ligating brackets (Quick; Forestadent, Pforzheim, Germany), and active ceramic self-ligating brackets (Clippy-C; Tomy, Tokyo, Japan). In the control group, conventional twin metal brackets (Micro-arch; Tomy) were used. All brackets were maxillary premolar brackets with 0.022 inch (in) slots and a -7° torque.
In order to measure the FR, 0.018 round and 0.019 × 0.025 rectangular SS archwires were used. Ten identical brackets from each group were used for measurements, and a total of 240 brackets were used according to the combinations of archwires and angles.
Brackets from each group were attached to 10 customized steel blocks (10 × 10 × 20 mm). Metal primer (Reliance Orthodontic Products, Itasca, IL, USA) was applied after microetching, and 1 min later, Transbond XT primer® (3M Unitek, Monrovia, CA, USA) was applied and light cured for 30 s. Vertical and horizontal lines crossing through the center of the blocks were drawn for use as guides during bracket positioning. The steel blocks with the attached brackets were inserted into larger rectangular blocks and fixed in place with screws such that angles of 0°, 5°, and 10° were created (Figure 1). The two blocks were positioned on the movable fixation cast of the universal testing machine (Instron 3366; Instron Corp., Norwood, MA, USA). One end of the archwire was fixed on a 150-g pendulum with a screw, while the other end was inserted into the tension load cell. Measurements were then obtained as the wire was drawn through the brackets. The conventional metal brackets were ligated with O-rings (Ormco, elastic rubber ligation material). FRs were measured at 0°, 5°, and 10° angles. Efforts were taken to prevent the generation of any extra torque, and the archwires and steel blocks with the attached brackets were replaced in each experiment. The experiments were conducted by the same individual. Measurements were obtained using a load cell of 500 N to draw 10 mm of wire at a crosshead speed of 10 mm/min using the universal testing machine. The maximum point on the weight-displacement curve that resulted from the measurement of the maximum FR was noted as the static FR. The kinetic FR was calculated as the mean value of 10 measurements obtained at 1-s intervals. Decay of the elastic ligatures was minimized. For each measurement, archwires and brackets were replaced with new ones to rule out possible wear effects or errors from repeated use. To assess the shape of the brackets, scanning electron microscopy (SEM, Hitachi-800; Hitachi, Tokyo, Japan) was used for comparison. Each bracket was measured in the 20 kV/s electron mode.
The SAS program (SAS Inc., Cary, NC, USA) was used for all statistical analyses. Standard deviations were calculated using the mean values for static and kinetic FRs measured in each group, and 3-way analysis of variance (ANOVA) was used to analyze the interaction effects between type of archwires, type of brackets, and bracket-archwire angles. For multiple comparisons, the Student-Newman-Keuls method was used, with a significance level of 0.05.
Tables 1 and 2 present the static and kinetic FRs for the four types of brackets according to bracket type, bracket-archwire angulation, and wire type. The results of three-factor ANOVA indicates that these factors had significant effects on both static and kinetic FRs. The interaction effect between bracket type and bracket-archwire angulation, between bracket type and wire type, and between bracket-archwire angulation and wire type were statistically significantly for both static and kinetic FRs (p < 0.001). The three-way interaction among bracket type, wire type, and bracket-archwire angulation was also statistically significant (p < 0.001).
Static FRs were generally increased with an increase in bracket-archwire angulation. The static FR was significantly higher with the rectangular wire than with the round wire (p < 0.001). The Damon3 MX brackets exhibited the lowest static FR at the 0° bracket-archwire angulation and a lower increase in static FR with an increase in bracket-archwire angulation than the other brackets.
Kinetic FRs also increased with an increase in bracket-archwire angulation and were higher with the rectangular wire than with the round wire (p < 0.001). Again, the Damon3 MX brackets exhibited the lowest kinetic FR, while the Micro-arch brackets exhibited the highest kinetic FR at the 0° bracket-archwire angulation. The increase in kinetic FR with an increase in the bracket-archwire angulation was higher with the Micro-arch bracket than with the experimental brackets.
Comparative observations of the shape and surface properties of the brackets were made using SEM (Figure 2). The overall shape of each bracket was observed under a 20× magnification, while the part of the slot where the archwire was bound was observed under a 200× magnification. The slot of the bracket was further magnified to 2,000×. The Damon3 MX brackets showed a soft area at the point of binding. The Quick brackets also revealed a relatively soft surface, but its active clip appeared somewhat rougher than that of the passive brackets. The Clippy-C brackets, while having well-polished surfaces and softer rounded angles in the slot, showed more porous surfaces than the other self-ligating brackets under the 2,000× magnification. The slot surfaces of the conventional metal brackets appeared somewhat rougher than those of the metal self-ligating brackets.
Forces from orthodontic wires are delivered to the teeth through bracket slots. This process often results in FRs between the orthodontic wires and bracket slots. It is important to obtain optimal forces during tooth movement, and an understanding of how FRs work in brackets is required. Kusy and Whitley11 reported that the loss of orthodontic force through FR generation ranges from 12% to 60% and that anchorage loss can result in orthodontic extraction cases. For satisfactory tooth movement, it is important to understand the magnitude of FRs developing between brackets and orthodontic wires. According to Cacciafesta et al.16, variables that can affect the FR include orthodontic wires, brackets, ligation method, and orthodontic appliances, among others. Orthodontic wires vary in size, shape, and material, but overall, SS wires cause the least FR. Thicker wires and rectangular wires tend to generate higher FRs.7 In this experiment, only SS wires were used, and similar to the findings of Garner et al.17, FRs were higher with rectangular wire than with round wire in all experimental groups. The rate of increase in FRs with an increase in the bracket-archwire angle was higher with the rectangular wire than with the round wire. With regard to FR according to bracket type, the passive metal self-ligating brackets exhibited lower FRs than the active ceramic self-ligating brackets. With rectangular wires, the active ceramic self-ligating brackets exhibited a slightly lower FR than the active metal self-ligating brackets. Kusy and Whitley11 compared the FRs of various types of metal self-ligating brackets and conventional metal brackets by altering the angle between the brackets and orthodontic wires, and they reported that the passive self-ligating brackets exhibited a low FR and that the active self-ligating brackets showed varying degrees of FR. Ceramic brackets exhibit a higher FR than metal brackets because orthodontic wires bind more easily with ceramic brackets, which have rough surfaces as opposed to metal brackets, which have relatively polished and softer surfaces. Although ceramic brackets are esthetically pleasing, higher FRs inside the bracket slots than those in metal brackets are considered a disadvantage.18 The reason why the ceramic self-ligating brackets used in this study may have shown lower FRs than the metal brackets could be polishing of the slot.
With regard to FRs according to the bracket-archwire angle, the rate of increase in FR was greater when the angle increased from 5° to 10° than when the angle increased from 0° to 5°. Furthermore, the rate of increase was greater with rectangular wires than with round wires. Pizzoni et al.15 reported that self-ligating brackets had low sliding forces at 0° and 3°, which increased proportionally with an increase in the angle to 6°, 9°, and 12°. This could be because the sliding movement of teeth can be disturbed by vertical forces produced at both ends of the bracket slot as the bracket-archwire angulation increases and causes binding. Frank and Nicolai19 reported that the effects of vertical forces decreased while the effects of archwire stiffness increased with an increase in the bracket-archwire angulation. With regard to the effects of the ligation method, the passive self-ligating brackets exhibited significantly lower FRs at all angles, while the conventional metal brackets that used elastic O-rings exhibited the highest FR. With regard to active self-ligating brackets, the FR was similar in the ceramic and metal brackets when a round archwire was used, while it was lower in the ceramic brackets when a rectangular archwire was used. In general, according to reports by previous researchers, self-ligating brackets are reportedly more effective in decreasing the FR than conventional brackets that use elastic or metal ligatures.20,21
Thorstenson and Kusy22 reported that passive self-ligating brackets exhibited a lower FR during sliding because the critical contact angle was large. The FR of self-ligating brackets in all groups increased more gradually than that of the conventional brackets as the bracket-archwire angle increased. The reason for the slightly higher FR in ceramic self-ligating brackets is that at the initial angle, ceramic causes more binding with orthodontic wires in the slot than metal.
Damon3 MX brackets are passive and showed low FRs at all bracket-archwire angles, with a gradual rate of increase in FR. Overall, under high magnification of an electron microscope, the corners of the bracket slots showed smooth surfaces (Figure 2A-2C). The Quick bracket, an active self-ligating bracket, showed well-polished surfaces overall (Figure 2D-2F). The ceramic self-ligating brackets also showed well-polished corners of the slot under high magnification as well as partially exposed crystalline particles and soft surfaces due to silica coating (Figure 2G-2I). The conventional metal brackets exhibited a higher overall FR; furthermore, under SEM, their slots had rougher surfaces than those of self-ligating brackets. These rougher surfaces may be correlated with the higher FRs exhibited by the conventional metal brackets (Figure 2J-2L). With an increase in the bracket-archwire angle, the ceramic self-ligating brackets exhibited lower FRs than the active metal self-ligating brackets. The ceramic self-ligating bracket clip is advantageous during sliding because it is more flexible than the active metal self-ligating bracket clip and because the contact angle is smaller because of the larger width of the ceramic bracket.
The width of the bracket also affects the contact angle formed between the bracket and orthodontic wire. The limit value where the passive configuration of the bracket and archwire becomes the active configuration is termed the critical contact angle. With an increase in bracket width, the critical contact angle decreases. Moreover, the width of the bracket can affect the moment that is delivered to the tooth; therefore, as the width of the bracket increases, a lower force is required to produce a moment and the contact angle decreases in space closure.
In this experiment, the four bracket types showed different widths. The Clippy-C brackets exhibited the largest width of approximately 0.132 in, while the Quick, Micro-arch, and Damon3 MX brackets exhibited widths of approximately 0.10-0.11 in. The Damon3 MX brackets exhibited the least width and a low FR in terms of critical contact angle. From this perspective, the Clippy-C brackets should exhibit a high FR, but it showed an FR similar to that exhibited by the Quick brackets from 5° to 10° bracket-archwire angulations. Considering that both brackets were active self-ligation systems, surface treatment and a round design in the slot angle area of the Clippy-C brackets also helped in decreasing the FR. Therefore, bracket design can also affect the FR.
The development of various types of self-ligating brackets is in progress to improve their physical and esthetic properties. Further studies on the esthetic advantages of and decreased FRs in self-ligating brackets during long-term orthodontic treatment are required. Furthermore, experiments using various angles and various types of orthodontic wires using artificial saliva are considered necessary.
In this study, compared to the active metal and ceramic self-ligating brackets, the passive metal self-ligating brackets showed the lowest static and kinetic FRs. However, the active ceramic self-ligating brackets showed a lower FR than the active metal self-ligating and conventional metal brackets. The findings of SEM analysis in our study suggest that self-ligating brackets can generate varying FRs in vitro according to the wire size, surface characteristics, and bracket-archwire angulation.
Figures and Tables
Figure 2
Comparison of scanning electron microscopy images of brackets. A-C, Damon3 MX brackets (20×, 200×, and 2,000× magnification, respectively). D-F, Quick brackets (20×, 200×, and 2,000× magnification, respectively). G-I, Clippy-C brackets (20×, 200×, and 2,000× magnification, respectively). J-L, Micro-arch brackets (20×, 200×, and 2,000× magnification, respectively).
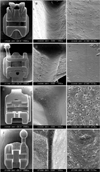
Table 1
Static frictional force values according to bracket type, bracket-archwire angle, and orthodontic wire type (unit: g)
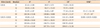
Notes
References
2. Kwak C, Kim JB, Son WS. A study on the changes of frictional force between bracket and orthodontic wire with time in artificial saliva. Korean J Orthod. 1993; 23:283–294.
3. Hwang HS, Park YC. An experimental study on frictional forces of various orthodontic wires under artificial saliva. Korean J Orthod. 1989; 19:245–256.
4. Ko JS, Yang KH. Frictional forces between orthodontic wire and bracket under artificial saliva. Korean J Orthod. 1988; 18:55–64.
5. Lee JH, Lee KS. An experimental study of dynamic frictional resistance between orthodontic bracket and arch wire. Korean J Orthod. 2001; 31:467–477.
6. Min JM, Suhr CH. A comparative study of frictional resistances between orthodontic brackets and arch wire during sliding movement of teeth. Korean J Orthod. 1988; 18:155–163.
7. Sung HM, Park YC. Comparison of the frictional resistance between orthodontic bracket & archwire. Korean J Orthod. 1991; 21:543–559.
8. Proffit WR. Contemporary orthodontics. St. Louis, USA: the C.V. Mosby Co.;1986. p. 263.
9. Peterson L, Spencer R, Andreasen G. A comparison of friction resistance for Nitinol and stainless steel wire in edgewise brackets. Quintessence Int Dent Dig. 1982; 13:563–571.
10. Thorstenson GA, Kusy RP. Comparison of resistance to sliding between different self-ligating brackets with second-order angulation in the dry and saliva states. Am J Orthod Dentofacial Orthop. 2002; 121:472–482.


11. Kusy RP, Whitley JQ. Influence of archwire and bracket dimensions on sliding mechanics: derivations and determinations of the critical contact angles for binding. Eur J Orthod. 1999; 21:199–208.


12. Cacciafesta V, Sfondrini MF, Scribante A, Klersy C, Auricchio F. Evaluation of friction of conventional and metal-insert ceramic brackets in various bracket-archwire combinations. Am J Orthod Dentofacial Orthop. 2003; 124:403–409.


13. Maijer R, Smith DC. Time savings with self-ligating brackets. J Clin Orthod. 1990; 24:29–31.
14. Kim H, Kim KY, Kang YG, Kim SH, Kook YA. Clinical considerations with self-ligating brackets. Korean J Orthod. 2006; 36:474–482.
15. Pizzoni L, Ravnholt G, Melsen B. Frictional forces related to self-ligating brackets. Eur J Orthod. 1998; 20:283–291.


16. Cacciafesta V, Sfondrini MF, Ricciardi A, Scribante A, Klersy C, Auricchio F. Evaluation of friction of stainless steel and esthetic self-ligating brackets in various bracket-archwire combinations. Am J Orthod Dentofacial Orthop. 2003; 124:395–402.


17. Garner LD, Allai WW, Moore BK. A comparison of frictional forces during simulated canine retraction of a continuous edgewise arch wire. Am J Orthod Dentofacial Orthop. 1986; 90:199–203.


18. Pratten DH, Popli K, Germane N, Gunsolley JC. Frictional resistance of ceramic and stainless steel orthodontic brackets. Am J Orthod Dentofacial Orthop. 1990; 98:398–403.


19. Frank CA, Nikolai RJ. A comparative study of frictional resistances between orthodontic bracket and arch wire. Am J Orthod. 1980; 78:593–609.


20. Shin HJ, Kwon OW, Kim KH. The effect of ligation method on the frictional force between orthodontic bracket and archwire. Korean J Orthod. 1998; 28:813–823.
21. Tecco S, Festa F, Caputi S, Traini T, Di Iorio D, D'Attilio M. Friction of conventional and self-ligating brackets using a 10 bracket model. Angle Orthod. 2005; 75:1041–1045.