Abstract
Objective
To investigate the involvement of ephrinB2 in periodontal tissue remodeling in compression areas during orthodontic tooth movement and the effects of compressive force on EphB4 and ephrinB2 expression in osteoblasts and osteoclasts.
Methods
A rat model of experimental tooth movement was established to examine the histological changes and the localization of ephrinB2 in compressed periodontal tissues during experimental tooth movement. RAW264.7 cells and ST2 cells, used as precursor cells of osteoclasts and osteoblasts, respectively, were subjected to compressive force in vitro. The gene expression of EphB4 and ephrinB2, as well as bone-associated factors including Runx2, Sp7, NFATc1, and calcitonin receptor, were examined by quantitative real-time polymerase chain reaction (PCR).
Results
Histological examination of the compression areas of alveolar bone from experimental rats showed that osteoclastogenic activities were promoted while osteogenic activities were inhibited. Immunohistochemistry revealed that ephrinB2 was strongly expressed in osteoclasts in these areas. Quantitative real-time PCR showed that mRNA levels of NFATc1, calcitonin receptor, and ephrinB2 were increased significantly in compressed RAW264.7 cells, and the expression of ephrinB2, EphB4, Sp7, and Runx2 was decreased significantly in compressed ST2 cells.
Orthodontic tooth movement is coupled with the remodeling of alveolar bone, which occurs due to mechanical forces such as compression and tension.1,2 Compressive force causes bone resorption on the compressed side of an orthodontically moving tooth, resulting in tooth movement. Two major cell types that are actively involved in the remodeling of alveolar bone are osteoblasts and osteoclasts. They are sensitive communicators between microenvironment and genome, capable of restoring system homeostasis disturbed by orthodontic mechanics.3 Under the load of orthodontic forces, many molecular reactions occur in and around these cells. However, the mechanism of these reactions has not been fully elucidated. A more comprehensive understanding of the underlying mechanism will provide valuable information for the development of agents regulating the activities of osteoblasts and osteoclasts to better control the bone resorption in compression areas, which is the rate-limiting step in orthodontic tooth movement.4
Some effectors of bone remodeling have been reported, and one of the most well-studied molecular networks in this process is the receptor activator of nuclear factor kappa B (RANK)/RANK ligand (RANKL)/osteoprotegerin (OPG) pathway.5,6,7 Osteoblasts can regulate osteoclast differentiation via this pathway. OPG is one of the rate-limiting agents for osteoclast differentiation and function,8 since it can block the membrane docking of RANK and RANKL. Recently, another family of molecules known as ephrin/Eph has been demonstrated to modulate the differentiation of osteoblasts and osteoclasts.9,10,11 Similar to RANK and RANKL, ephrins and Ephs have a ligand-receptor relationship-ephrins are transmembrane ligands, and Ephs are tyrosine kinase receptors. Interaction between ephrinB- and EphB-expressing cells results in bidirectional signal transduction. The activation of EphB receptors by ephrinB ligands is referred to as "forward signaling", whereas the activation of ephrinB ligands by EphB receptors is designated "reverse signaling". Ephrin and Eph were characterized initially through their roles in embryogenesis,12 as ephrin-Eph signaling is critically involved in neural development, cell morphogenesis, tissue patterning, and angiogenesis.13
Within the ephrin/Eph family, EphB4 and its preferred ligand, ephrinB2, have been studied intensively in osteoblasts and osteoclasts. EphrinB2 expression is induced during osteoclast differentiation, and then reverse signaling through this ligand suppresses osteoclast differentiation, forming a negative feedback loop.9 Moreover, forward signaling through the EphB4 receptor on osteoblasts enhances their differentiation. The bidirectional activation of the ephrinB2-EphB4 signaling pathway in osteoclasts and osteoblasts leads to the suppression of osteoclast differentiation with concurrent stimulation of osteoblast differentiation and, consequently, bone formation. The communication between ephrinB2 and EphB4 has recently been shown to be involved in the stimulation of osteoblast differentiation within the osteoblast lineage.14,15 In addition, ephrinB2 and EphB4 have been demonstrated to be regulated by mechanical forces in endothelial progenitor cells and periodontal ligament fibroblasts.16,17,18 It was shown that mechanical strain induced ephrinB2 upregulation in periodontal ligament fibroblasts, contributing to osteogenesis at tension sites of orthodontic tooth movement.17 However, the effects of compressive force on these two molecules in osteoblasts and osteoclasts have not been clearly elucidated.
Our objective was to investigate the involvement of ephrinB2 in periodontal tissue remodeling in compression areas during orthodontic tooth movement and the effect of compressive force on ephrinB2 and EphB4 expression in osteoblasts and osteoclasts. We hypothesized that compressive force would affect alveolar bone remodeling through the regulation of ephrinB2 and EphB4 expression during orthodontic tooth movement.
The murine monocyte/macrophage cell line RAW264.7 and stromal cell line ST2 were obtained from the Chinese Academy of Sciences Cell Bank (Shanghai, China). A four-point bending system was obtained from Sichuan University (Chengdu, China). The force-loading plates were made from the bottom part of the 75-cm2 cell canted-neck culture flasks (Falcon #353135; Corning Life Sciences, Tewksbury, MA, USA), each 3 × 8 cm2 in area and 1.15 mm thick. Rabbit anti-human ephrinB2 polyclonal antibodies were purchased from Santa Cruz Biotechnology (Santa Cruz, CA, USA).
All procedures involving animals were approved by the Animal Care and Use Committee of Jilin University. The animals used were 8-week-old male Wistar rats, with an average weight of 200 g. The rats were anesthetized by intramuscular injection with 0.2 mL·kg-1 Sumianxin (Academy of Military Medical Sciences, Changchun, China). A nickel-titanium closed-coil spring exerting an orthodontic force of 50 cN was ligated unilaterally to the maxillary first molar and incisor (Figure 1). The maxillary first molar on the opposite side was not moved and was used as the control group. We examined a total of 10 teeth in five rats. Experimental tooth movement was conducted for 3 days (n = 5).
Rats were sacrificed by intracardial perfusion with 4% paraformaldehyde in 0.1 M of phosphate buffer (pH 7.4) under anesthesia. The maxillae were dissected and molar-bearing segments of alveolar bone were cut from each side and further fixed overnight in 4% paraformaldehyde buffer. The specimens were decalcified in 10% ethylenediamine tetraacetic acid solution (pH 7.4) for 8 weeks, and further dehydrated in ascending concentrations of ethanol and embedded in paraffin. Serial 5-µm-thick sections including the crestal areas mesial and distal to the maxillary molars were cut and mounted on L-poly-lysine-coated glass slides. Sections were stained with hematoxylin and eosin and immunohistochemistry was performed. For immunohistochemistry staining, endogenous peroxidase in the tissue section was blocked with 1% hydrogen peroxide for 10 minutes and then further blocked with 1% goat serum in phosphate-buffered saline for 30 minutes. The primary antibody used was polyclonal rabbit anti-human ephrinB2. An immunohistochemistry staining kit (Maixin Bio, Fuzhou, China) was used for further staining. Finally, sections were counterstained with hematoxylin and mounted. Negative controls were labeled using the same procedures, but omitting the incubation with primary antibodies. Staining density was analyzed using Image-Pro Plus (Media Cybernetics, Bethesda, MD, USA) and represented as the mean density.
RAW264.7 cells were maintained in low-glucose Dulbecco's modified Eagle medium (L-DMEM; Life Technologies, Gaithersburg, MD, USA) and ST2 cells in alpha-minimum essential medium containing 10% fetal bovine serum (Life Technologies), 100 U·mL-1 penicillin, and 100 mg·mL-1 streptomycin (Life Technologies) at 37℃ in a humidified atmosphere of 5% CO2. The medium was changed every 2-3 days. The cells were seeded onto the force-loading plate at a density of 1 × 105 cells·cm-2. RAW264.7 cells on the force-loading plates were cultured in L-DMEM containing 50 ng·mL-1 recombinant mouse RANKL (rmRANKL; R&D Systems, Minneapolis, MN, USA). RAW cells differentiated into tartrate resistant acid phosphatase-positive multinucleated osteoclastic cells in the presence of RANKL.19
The force-loading plates were subjected to cyclic uniaxial compressive strain by the four-point bending system20 at 0.5 Hz (Figure 2). Cells were loaded with compressive force at 2000 µε (µε is defined as deformation, with 1,000 µε corresponding to 0.1% deformation) for 1, 2, and 4 hours, respectively. Control cells were cultured on similar plates without any mechanical loading and kept in the same incubator.
Cells were harvested after 1, 2, and 4 hours of the application of compressive force. Total RNA was extracted using an EASYspin RNA extraction reagent kit (Biomed, Beijing, China) from cells cultured on the plates according to the manufacturer's instructions. RNA was reverse-transcribed using the PrimerScript® RT reagent kit (Takara, Dalian, China) with gDNA eraser to synthesize cDNA. qPCR (n = 3) was performed using SYBR Green Premix Ex Taq (Takara) and the MxPro Mx3005P real-time PCR detection system (Agilent Technologies, Santa Clara, CA, USA). Glyceraldehyde 3-phosphate dehydrogenase (GAPDH) was used as a normalization control. The primers used for qPCR were shown in Table 1. The cycling conditions were as follows: 95℃ for 30 seconds followed by 40 cycles of 95℃ for 5 seconds, 55℃ for 30 seconds, and 72℃ for 1 minute. The 2-ΔΔCt method was used for calculating the relative expression levels, and values were the average of triplicate measurements.
All experiments were performed on at least three individuals. Results are presented as the mean ± standard deviation (SD). The significance of the differences was determined using the two-tailed Student's t-test and one-way analysis of variance (ANOVA). The difference was considered significant if p < 0.05. The IBM SPSS ver. 19.0 for Windows (IBM Co., Armonk, NY, USA) was used for statistical analysis.
Histological examination showed a gap between the first and second molar indicating that the loaded maxillary first molar was moved mesially. In the compression area, the surface of alveolar bone was rough and there were resorption lacunae on the bone surface (Figure 3). Multinucleated osteoclasts were seen adjacent to the alveolar bone surface. No osteoblasts were observed in the compression area.
Since no osteoblasts were found in the compression area, only ephrinB2 was examined by immunohistochemistry. EphrinB2 immunoreactivity was observed in fibroblasts in periodontal tissues from both the control and experimental groups (Figure 4A and 4B). In periodontal tissues of the control group without tooth movement, ephrinB2 was weakly and uniformly expressed in periodontal fibroblasts (Figure 4A). In periodontal tissues of the experimental group, ephrinB2 was strongly expressed in osteoclasts adjacent to alveolar bone in the compression area (Figure 4B). Negative controls showed no immunolabeling (Figure 4C). As shown in Table 2, the difference in ephrinB2 expression levels in periodontal tissues between the experimental and control groups was statistically significant (p < 0.05).
In order to investigate whether compressive force could directly stimulate osteoclast differentiation, we applied cyclic uniaxial compressive force produced by a four-point bending system to RAW264.7 cells. Following this, gene expression was assessed for two well-known markers of differentiated osteoclasts, nuclear factor of activated T cells cytoplasmic 1 (NFATc1)21,22,23 and calcitonin receptor (CTR).24 As shown in Figure 5A and 5B, the levels of NFATc1 and CTR mRNA were significantly upregulated in RAW264.7 cells after 1 hour and 2 hours of the application of compressive force (p < 0.05). Our data indicate that cyclic compressive force can promote the differentiation of osteoclasts.
To understand the relationship between compressive force and ephrinB2 expression in osteoclasts, we evaluated the expression of ephrinB2 in RAW264.7 cells. As shown in Figure 5C, there was a significant increase in the expression of the ephrinB2 gene after 1 hour and 2 hours in compressed RAW264.7 cells (p < 0.05).
To investigate the response of osteoblasts to compressive force, we performed an in vitro experiment using ST2 cells. The expression of the transcription factors Runx2 and Sp7 was assessed in these cells after the application of compressive force. Runx2 plays a key role in mediating osteoblast maturation and is necessary for normal osteogenesis,25,26 while Sp7 is believed to be downstream of Runx2 and may promote osteoblast maturation.27,28 As shown in Figure 6A and 6B, the mRNA levels of Runx2 and Sp7 were significantly decreased in ST2 cells after the application of compressive force (p < 0.05). These findings suggest that compressive force can inhibit osteoblast differentiation.
It has been reported that both EphB4 and ephrinB2 are found in osteoblasts. To understand whether EphB4 and ephrinB2 expression in osteoblasts is affected by compressive force, we evaluated the expression of these molecules in ST2 cells. As shown in Figure 6C and 6D, there was a significant decrease in the expression of the EphB4 gene in ST2 cells after 1 hour and 2 hours of exposure to compressive force (p < 0.05). A significant decrease in the expression of ephrinB2 was also detected after 4 hours of compressive force (p < 0.05).
The findings of this study, which show the response of tissues in compression areas during orthodontic tooth movement, agree with many previous histological studies.29,30 We determined that multinucleated osteoclasts adhered to resorption lacunae along the alveolar bone surface, and no osteoblasts were observed. EphrinB2 was examined by immunohistochemistry; however, since no osteoblasts were found in the compression area, EphB4 expression was not assessed. We observed strong expression of ephrinB2 in osteoclasts, suggesting that this ligand may be involved in periodontal tissue remodeling in the compression area during orthodontic tooth movement. However, it is unknown whether the applied orthodontic force directly induced this cellular reaction. To clarify this point, an in vitro study was performed to investigate the effects of compressive force on ephrinB2 and EphB4 in cultured osteoblasts and osteoclasts.
The direct effect of mechanical force on osteoclast differentiation19 has previously been investigated in only a few studies. These yielded limited information, as many analyses were done using primary cultured bone cells, which were mixtures of osteoclasts, osteoblasts, and other undifferentiated cells. In the present study, by analyzing changes in the gene expression of NFATc1 and CTR in RAW 264.7 cells we provided direct evidence that compressive force can promote osteoclast differentiation.
It has been found that ephrinB2, but not EphB4, is present in multinucleated osteoclasts and differentiating mononuclear osteoclasts.9 As such, only ephrinB2 was examined in RAW264.7 cells in our study. Moreover, it has been reported that ephrinB2 expression is induced and upregulated during osteoclast differentiation. This molecule is markedly expressed at later stages of osteoclast differentiation, and its induction is dependent on NFATc1 and the proto-oncogene c-Fos. In our study, osteoclast differentiation was promoted by compressive force and accompanied by an increase in ephrinB2 expression. Our results therefore support previous findings.
Osteoblasts and osteoclasts are two major types of bone cells that are responsible for bone remodeling. These two types of cells can communicate with each other via ephrinB2-EphB4 bidirectional signal transduction. In addition, self-communication within osteoblasts is also possible because osteoblasts can express both ephrinB2 and EphB4. In our study, EphB4 and ephrinB2 expression in ST2 cells was downregulated by compressive force. This downregulation would attenuate ephrinB2-EphB4 signaling between osteoblasts and osteoclasts, as well as within the osteoblast lineage. Consequently, osteoblast differentiation and subsequent bone formation via the ephrinB2-EphB4 signaling pathway would be attenuated, which could facilitate bone resorption in compression areas of alveolar bone during orthodontic tooth movement.
EphrinB2 has been demonstrated to be induced by the c-Fos-NFATc1 transcriptional cascade in osteoclasts, and is expressed at a later stage of osteoclast differentiation.
Furthermore, EphB4-ephrinB2 reverse signaling through ephrinB2 suppresses c-Fos-NFATc1 transcription and osteoclast differentiation, forming a negative feedback loop.9 In our study, in vitro and in vivo ephrinB2 expression was induced by compressive force as well as osteoclast differentiation, which is consistent with previous studies.
The molecular genetic processes underlying orthodontic
tooth movement function as a feedback system of checks and balances.3 Activator molecules beget suppressor molecules and then return to their steady states, and examples of this system include tumor necrosis factor and its receptor, colony stimulating factor-1, OPG, and RANK and RANKL.4 EphrinB2 and EphB4 could be added to this list of regulatory molecules, as our findings suggest they regulate bone resorption during orthodontic tooth movement.
Figures and Tables
Figure 1
A rat model of orthodontic tooth movement can provide compressive force on alveolar bone. A nickel-titanium closed-coil spring exerting an orthodontic force was ligated unilaterally to the maxillary first molar and incisor. The maxillary first molar on the other side was without movement and was used as the control group. A, Lateral and occlusal view of the rat maxillae with appliance. B, The principle of orthodontic tooth movement: when the tooth was subjected to mechanical loading, some compression areas occurred on the alveolar bone surface around the tooth.
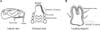
Figure 2
A four-point bending system can provide cyclic uniaxial compressive force on adherent cells in vitro. A, Actuator. B, Digital-control part. C, Culture dish with pressure head. D, Schematic representation of the apparatus used for application of mechanical force to cell cultures. The cell suspension was plated on a plastic plate, then, after 2 hours of cultivation, cells were subjected to cyclic compressive force in culture medium. E, The principle of the four-point bending system used for compressive force application (conceptual diagram).
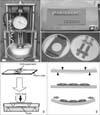
Figure 3
Histological examination of the compression areas of periodontal tissues from the experimental group. Multinucleated osteoclasts were seen adjacent to the alveolar bone surface, with no osteoblasts observed. Hematoxylin and eosin stain, 200× magnification.
AB, Alveolar bone; R, root of molar; OC, osteoclast.
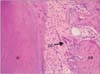
Figure 4
Immunohistochemical analysis of ephrinB2 expression in periodontal tissues. A, Photomicrograph showing weak anti-ephrinB2 antibody immunolabeling of fibroblasts in periodontal tissues from the control group (without tooth movement). B, Photomicrograph showing strong anti-ephrinB2 antibody immunolabeling of osteoclasts in the compression area of periodontal tissues from the experimental group. C, Negative controls show no immunolabeling of osteoclasts. All images are at 200× magnification.
AB, Alveolar bone; OC, osteoclast.
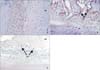
Figure 5
The effects of compressive force on osteoclastogenic gene and ephrinB2 expression in RAW264.7 cells. RAW264.7 cells stimulated with RANKL were exposed to compressive force or no force for 1, 2 and 4 hours. The mRNA expression of A, NFATc1, B, CTR, and C, ephrinB2 was then determined in control and compressed cells using quantitative real-time PCR. GAPDH was used as the internal reference gene. Data from one representative experiment of three are shown.
RANKL, Receptor activator of nuclear factor kappa B ligand; NFATc1, nuclear factor of activated T cells cytoplasmic 1; CTR, calcitonin receptor; PCR, polymerase chain reaction; GAPDH, glyceraldehyde 3-phosphate dehydrogenase.
*p < 0.05, **p < 0.01.
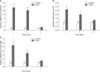
Figure 6
The effects of compressive force on the expression of osteogenic genes, ephrinB2, and EphB4 in ST2 cells. ST2 cells were exposed to compressive force or no force for 1, 2, and 4 hours. The mRNA expression of A, Runx2, B, Sp7, C, ephrinB2, and D, EphB4 was then determined in control and compressed cells. Glyceraldehyde 3-phosphate dehydrogenase (GAPDH) was used as the internal reference gene. Data from one representative experiment of three are shown.
*p < 0.05.
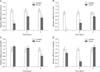
Notes
References
1. Krishnan V, Davidovitch Z. Cellular, molecular, and tissue-level reactions to orthodontic force. Am J Orthod Dentofacial Orthop. 2006; 129:469.e1–469.e32.


2. Wise GE, King GJ. Mechanisms of tooth eruption and orthodontic tooth movement. J Dent Res. 2008; 87:414–434.


3. Masella RS, Meister M. Current concepts in the biology of orthodontic tooth movement. Am J Orthod Dentofacial Orthop. 2006; 129:458–468.


4. Roberts WE, Huja S, Roberts JA. Bone modeling: biomechanics, molecular mechanisms, and clinical perspectives. Sem Orthod. 2004; 10:123–161.


5. Oshiro T, Shiotani A, Shibasaki Y, Sasaki T. Osteoclast induction in periodontal tissue during experimental movement of incisors in osteoprotegerin-deficient mice. Anat Rec. 2002; 266:218–225.


6. Kanzaki H, Chiba M, Shimizu Y, Mitani H. Periodontal ligament cells under mechanical stress induce osteoclastogenesis by receptor activator of nuclear factor kappaB ligand up-regulation via prostaglandin E2 synthesis. J Bone Miner Res. 2002; 17:210–220.


7. Kanzaki H, Chiba M, Takahashi I, Haruyama N, Nishimura M, Mitani H. Local OPG gene transfer to periodontal tissue inhibits orthodontic tooth movement. J Dent Res. 2004; 83:920–925.


8. Harada S, Rodan GA. Control of osteoblast function and regulation of bone mass. Nature. 2003; 423:349–355.


9. Zhao C, Irie N, Takada Y, Shimoda K, Miyamoto T, Nishiwaki T, et al. Bidirectional ephrinB2-EphB4 signaling controls bone homeostasis. Cell Metab. 2006; 4:111–121.


10. Edwards CM, Mundy GR. Eph receptors and ephrin signaling pathways: a role in bone homeostasis. Int J Med Sci. 2008; 5:263–272.


12. Holder N, Durbin L, Cooke J. Eph receptors and ephrins are key regulators of morphogenesis. Ernst Schering Res Found Workshop. 2000; (29):123–147.


13. Pasquale EB. Eph receptor signalling casts a wide net on cell behaviour. Nat Rev Mol Cell Biol. 2005; 6:462–475.


14. Allan EH, Häusler KD, Wei T, Gooi JH, Quinn JM, Crimeen-Irwin B, et al. EphrinB2 regulation by PTH and PTHrP revealed by molecular profiling in differentiating osteoblasts. J Bone Miner Res. 2008; 23:1170–1181.


15. Martin TJ, Allan EH, Ho PW, Gooi JH, Quinn JM, Gillespie MT, et al. Communication between ephrinB2 and EphB4 within the osteoblast lineage. Adv Exp Med Biol. 2010; 658:51–60.


16. Obi S, Yamamoto K, Shimizu N, Kumagaya S, Masumura T, Sokabe T, et al. Fluid shear stress induces arterial differentiation of endothelial progenitor cells. J Appl Physiol (1985). 2009; 106:203–211.


17. Diercke K, Kohl A, Lux CJ, Erber R. Strain-dependent up-regulation of ephrin-B2 protein in periodontal ligament fibroblasts contributes to osteogenesis during tooth movement. J Biol Chem. 2011; 286:37651–37664.


18. Diercke K, Sen S, Kohl A, Lux CJ, Erber R. Compression-dependent up-regulation of ephrin-A2 in PDL fibroblasts attenuates osteogenesis. J Dent Res. 2011; 90:1108–1115.


19. Suzuki N, Yoshimura Y, Deyama Y, Suzuki K, Kitagawa Y. Mechanical stress directly suppresses osteoclast differentiation in RAW264.7 cells. Int J Mol Med. 2008; 21:291–296.


20. Liu J, Liu T, Zheng Y, Zhao Z, Liu Y, Cheng H, et al. Early responses of osteoblast-like cells to different mechanical signals through various signaling pathways. Biochem Biophys Res Commun. 2006; 348:1167–1173.


21. Takayanagi H, Kim S, Koga T, Nishina H, Isshiki M, Yoshida H, et al. Induction and activation of the transcription factor NFATc1 (NFAT2) integrate RANKL signaling in terminal differentiation of osteoclasts. Dev Cell. 2002; 3:889–901.


22. Asagiri M, Sato K, Usami T, Ochi S, Nishina H, Yoshida H, et al. Autoamplification of NFATc1 expression determines its essential role in bone homeostasis. J Exp Med. 2005; 202:1261–1269.


23. Takayanagi H. Mechanistic insight into osteoclast differentiation in osteoimmunology. J Mol Med (Berl). 2005; 83:170–179.


24. Lee SK, Goldring SR, Lorenzo JA. Expression of the calcitonin receptor in bone marrow cell cultures and in bone: a specific marker of the differentiated osteoclast that is regulated by calcitonin. Endocrinology. 1995; 136:4572–4581.


25. Choi JY, Pratap J, Javed A, Zaidi SK, Xing L, Balint E, et al. Subnuclear targeting of Runx/Cbfa/AML factors is essential for tissue-specific differentiation during embryonic development. Proc Natl Acad Sci U S A. 2001; 98:8650–8655.


26. Pratap J, Galindo M, Zaidi SK, Vradii D, Bhat BM, Robinson JA, et al. Cell growth regulatory role of Runx2 during proliferative expansion of preosteoblasts. Cancer Res. 2003; 63:5357–5362.
27. Nakashima K, Zhou X, Kunkel G, Zhang Z, Deng JM, Behringer RR, et al. The novel zinc finger-containing transcription factor osterix is required for osteoblast differentiation and bone formation. Cell. 2002; 108:17–29.


28. Kaback LA, Soung do Y, Naik A, Smith N, Schwarz EM, O'Keefe RJ, et al. Osterix/Sp7 regulates mesenchymal stem cell mediated endochondral ossification. J Cell Physiol. 2008; 214:173–182.

