Abstract
Objective
This study evaluated the cytotoxicity and genotoxicity of fixed orthodontic treatment with three different light-cured orthodontic bonding composites by analyzing micronucleus (MN) formation in the buccal mucosa during a 6-month period.
Methods
Thirty healthy volunteers were selected from consecutive patients referred for orthodontic treatment. Equilibrium 2 brackets and molar tubes (Dentaurum) were bonded with three different light-cured orthodontic bonding composites-Transbond XT (3M Unitek), Kurasper F (Kuraray Europe), or GrenGloo (Ormco Corporation)- to all teeth in both arches. Exfoliated buccal epithelial cells were scraped from the middle part of the inner cheeks with sterile cement spatulas before treatment and at 1, 3, and 6 months after treatment. MNs and nuclear alterations, such as karyorrhexis (KR), karyolysis (KL), and binucleated cells (BNs), were scored under a light microscope. Repeated measure ANOVA was used to calculate statistical differences in degenerative nuclear abnormalities.
Results
MN rates did not significantly differ among different time points within the same cell type (p > 0.05). In contrast, the number of BNs in buccal epithelial cells significantly increased in all composite groups (p < 0.01, Transbond XT; p < 0.001, Kurasper F and GrenGloo). KL frequency significantly increased between the beginning and end of the study in the Kurasfer F (0.80 ± 0.79 to 1.90 ± 1.10; p < 0.05) and GrenGloo (1.30 ± 1.06 to 2.40 ± 1.08; p < 0.05) groups.
Since the introduction of the acid-etch bonding technique several decades ago, orthodontists have successfully and reliably employed fixed appliances in clinical practice. Orthodontic light-cured adhesive materials have had additional advantages over previously used adhesives, including simplicity of use and increased time for bracket application.1 Despite great advancements in the development of photoactivated resin composites, their biocompatibility remains a concern for orthodontists, since the bonding agents are generally left in close contact with oral tissue over long periods of time.2
Bisphenol A diglycidyl dimethacrylate (bis-GMA) and triethylene glycol dimethacrylate (TEGDMA) are two primary monomers in the resin matrices of orthodontic bonding systems. The matrix sometimes exists as a combination of multiple different monomeric units, such as bis-GMA and urethane dimethacrylate (UDMA). Several co-monomers (e.g., ethylene glycol dimethacrylate [EGDMA], diethylene glycol dimethacrylate [DEGDMA], TEGDMA) and various additives such as photoinitiators (e.g., camphorquinone), co-initiators (e.g., 4-N,N-Dimethyl amino benzoic acid ethylester [DMABEE], 2-(diethylamino)ethyl methacrylate [DEAEMA]), inhibitors (e.g., butylated hydroxytoluene [BHT]), ultraviolet absorbers, photostabilizers, and pigments are selectively included.3
These compounds may induce allergic reactions in some treated individuals.4 Additionally, several studies have reported symptoms that could be attributable to the mutagenic/genotoxic5 and cytotoxic effects of the materials.6,7 Genotoxicity is the capacity of an agent to exert deleterious effects on a cell's genetic material, thereby affecting its genetic integrity. This agent may be a chemical compound or certain types of radiation. Cytotoxicity is the capacity of an agent to be toxic to living cells. Examples of cytotoxic agents include chemical compounds, immune cells, and certain types of venom. To evaluate the genotoxic and cytotoxic effects of orthodontic bonding composites, a variety of different test techniques have been used, such as the MTT assay,8 protooncogene expression analysis,2 the Comet assay,9 and the micronucleus test (MNT).10,11
The MNT has been used to evaluate the genotoxicity of dental adhesives.12 Micronucleus (MN) deposits contain abnormal genetic material that were lost from the genome during mitosis as a consequence of toxic exposure of cells to radiation or chemical agents. Estimation of the MN formation induced in cells by mutagenic factors has been widely used for assessment of cytogenetic alteration.13,14 This method has been applied to blood lymphocytes,14 gingival cells,2,5 and exfoliated epithelium cells.10 In addition to MN formation, measurement of other degenerative nuclear alterations-such as karyorrhexis (KR), karyolysis (KL), and binucleated cells (BNs)- has also been recommended for evaluating cytotoxicity effects.15
Fixed orthodontic treatment includes orthodontic composites as well as metallic components, such as bands, brackets, and wires. As a result, metallic ions and monomers are inevitably released from the orthodontic appliances into the oral environment during treatment.11 This possibility is of great clinical concern, especially for patients who are genetically predisposed to certain clinical conditions.
There is a lack of comprehensive data in the literature regarding the toxicity of light-cured orthodontic bonding composites.1 Moreover, only a few studies have examined genetic and cytological effects over time after light-induced polymerization and the potential of composites to interact with oral tissue.16 Therefore, the purpose of this study was to evaluate the frequency of MN formation as a measure of genotoxicity in the buccal mucosa during fixed orthodontic treatment with three different light-cured orthodontic composites, which were commonly used in orthodontic practice, during a 6-month period. Additionally, nuclear alterations in the form of BNs, KL, and KR were also assessed to determine the cytotoxic effects of these composite samples.
The study protocol conforms to the guidelines of the Helsinki Declaration on human experimentation. The study design was accepted by the ethics committee of Inonu University (no: 2011/219). Written informed consent was obtained from all participants.
The study group consisted of 30 healthy volunteers (12 boys and 18 girls) with a mean age of 14.08 ± 1.79 years (range: 11.17-17.67 years) (Table 1). All volunteers were selected from consecutive patients referred for orthodontic treatment to the Department of Orthodontics, Inonu University (Malatya, Turkey), were interviewed, and underwent an oral examination. Patients were included in the study if they had good oral hygiene, no tooth decay, and no fillings. Patients with more than four lost teeth, repeated aphthous stomatitis, and skin reactions were excluded. No subject had a history of alcoholism and smoking. Additionally, no subject was using any oral antiseptic solutions at the time of the study.
The selected subjects were randomly segregated with the help of a randomizer software (Research Randomizer, ver. 4.0 [computer software]; Urbaniak GC and Plous S, available from http://www.randomizer.org/) into three study groups (Transbond XT, 3M Unitek Ortho Prod, Monroe, LA, USA; Kurasper F, Kuraray Europe GmbH, Frankfurt, Germany; and GrenGloo, Ormco Corporation, Orange, CA, USA) (Table 2), each consisting of 10 subjects. Orthodontic records, such as lateral and frontal cephalometric X-rays and panoramic dental radiographs, were collected for all subjects. However, orthodontic treatment was only initiated at 3 months after X-ray exposure to eliminate the effects of radiation.
Buccal cell-exfoliated epithelium samples were collected at four separate time points from each patient. The first samples were collected at the beginning of the study (T1), regarded as the baseline control (Figure 1). For standardization, Equilibrium 2 brackets and direct molar tubes (Dentaurum, Ispringen, Germany) were bonded with three different orthodontic light-cured composites to all teeth in both arches (according to the manufacturer's instructions), including second molars if they had erupted. The bonding composites used in this study are shown in Table 2. In each group, 0.012-inch, 0.014-inch, and 0.016-inch Rematitan ideal, round, nickel titanium arch wires (Dentaurum) were applied with 0.010-inch Remanium preformed stainless steel ligature wires (Dentaurum) at 2-month intervals. One and three months after appliance placement, the same investigator (OHK) collected the second and third samples (T2 and T3, respectively). The final records (T4) were obtained after the sixth month of bracket placement.
All patients were instructed to rinse their mouths twice with tap water before the investigator collected exfoliated epithelial cells from the buccal mucosa. All samples were scraped from the middle part of the inner cheeks with sterile cement spatulas by the same investigator (OHK). The samples obtained were smeared onto clean microscope glass slides. Three to five slides from each sample were prepared, air-dried, and immediately fixed in a methanol:glacial acetic acid (3:1) mixture. Next, samples were stained with acridine orange (Sigma Chemical Co., St. Louis, MO, USA) dissolved in bi-distilled water (0.01%) for 10 minutes.
MNs and nuclear anomalies were analyzed in terms of the frequency of occurrence. A single investigator (SY) performed blinded analysis and scored a total of 2,000 cells from each set of stained slides under a light microscope without special filters for acridine orange (Olympus BX50; Olympus Co. Ltd., Tokyo, Japan). Verification of MNs and nuclear alterations was primarily conducted using the criteria of Tolbert et al.15 and applied as reported by Öztürk et al.11 In order to score MNs and nuclear anomalies, cells were analyzed for each sample at ×200 magnification, and photographs were taken at ×1,000 magnification using an Olympus BH2 oil immersion lens (Olympus Co. Ltd.) oil immersion lens.
Mean, standard deviation, minimum and maximum values of degenerative nuclear abnormalities, as appropriate, were calculated as the descriptive measurements of this study. Repeated measure ANOVA (RMANOVA)tests were used to calculate statistical differences in degenerative nuclear abnormalities (MN, BN, KL, and KR) during the different periods within these groups. Comparisons between all pairs of groups were tested with Bonferroni multiple comparison tests. The level of significance was set at p < 0.05. The data were computationally tested using SPSS for Windows, version 14.0 (SPSS Inc., Chicago, IL, USA).
Table 3 shows the comparative frequency of MNs, BNs, KL, and KR at different time points within the groups. When comparing the MN rates among different time points within the same cell type using RMANOVA analysis, no statistically significant differences were observed (p > 0.05; Figure 2).
The number of BNs in buccal epithelial cells significantly increased in all composite groups (p < 0.01 for Transbond XT; p < 0.001 for Kurasper F and GrenGloo; Figure 3A), as determined by RMANOVA analysis. Statistically significant increases were observed in all groups between the T1 and T4 time points (3.40 ± 1.27 to 5.40 ± 1.17 for Transbond XT; 3.00 ± 1.05 to 5.00 ± 0.82 for Kurasper F; 3.20 ± 1.69 to 5.30 ± 1.34 for GrenGloo; p < 0.01) and between the T2 and T4 time points (3.80 ± 1.03 to 5.40 ± 1.17 for Transbond XT, p < 0.01; 2.80 ± 1.03 to 5.00 ± 0.82 for Kurasper F, p < 0.001; 2.90 ± 0.74 to 5.30 ± 1.34 for GrenGloo, p < 0.001).
Furthermore, the frequency of KL significantly increased between T1 and T4 in the Kurasfer F group (0.80 ± 0.79 to 1.90 ± 1.10; p < 0.05) and GrenGloo group (1.30 ± 1.06 to 2.40 ± 1.08; p < 0.05; Figure 3B). Additionally, the frequency of KL significantly increased in the GreenGloo group between T2 and T4 (1.20 ± 0.92 to 2.40 ± 1.08; p < 0.01) and between T3 and T4 (1.40 ± 1.08 to 2.40 ± 1.08; p < 0.05). The frequency of KL did not differ in the Transbond XT group between the T1 and T4 time points.
The frequency of KR in buccal epithelial cells showed no significant differences between different time points within the groups (p > 0.05; Figure 3C), as determined by RMANOVA analysis.
Brackets, bands, and arch wires used as appliances in fixed orthodontic treatments are fabricated from alloys, including nickel, cobalt, and chromium. In addition to these metallic ions, a variety of monomers released from bonding composites have deleterious effects on adjacent oral tissue, such as allergic skin reactions. The mucosa of the mouth or pharynx may absorb residual monomers or they may be ingested into the digestive system via saliva.17,18,19 Therefore, in the present study, we aimed to evaluate the long-term effects of metallic ions and residual monomers reported to have harmful, perhaps synergistic, effects on buccal mucosa cells.10,20 Under most clinical conditions, it cannot be determined whether the effects are due to orthodontic composites or metallic components.
The extraction assay is one of the most frequently used methods in the study of orthodontic-bonding composites for investigating the mechanism underlying intraoral cytotoxicity.8 The MNT is a validated alternative way of sensitive detection for chromosomal aberrations.21 Some genotoxic chemicals, for example, tobacco products, pesticides, and alcohol, have been examined in exfoliated buccal mucosa cells by using estimates of MN formation.22
However, analysis of chromosomal abnormalities or signs of genotoxicity in mammalian cells is labor-intensive, time-consuming, and requires an experienced operator.12 Although cell culture tests are simple, accurate, reliable, and rapid, they detect the agent's effect only on isolated cells derived from animal or human tissue and grown in culture plates for in vitro testing.8 Previous studies have used animal models to perform biocompatibility testing of orthodontic adhesives.17,23
Various types of dental materials have been reported to possess genotoxic and cytotoxic capabilities, including alterations in chromosomal integrity, cell-cycle progression, DNA replication, and repair in normal cultured human lymphocytes, although researchers have acknowledged that their results could not be directly applied to real-world clinical settings.24 Therefore, we evaluated the genotoxic and cytotoxic effects of orthodontic treatment with various orthodontic composites on human oral mucosa cells in such a setting.
Evaluation of the increase in number of MNs within the adhesive groups by the MNT showed that the fixed orthodontic treatment had no genotoxic effects. For experimental standardization among all the adhesive groups examined, the same brand of metallic products, including brackets, tubes, arch wires, and ligature wires, were applied in the fixed orthodontic treatments during the time period evaluated. Since Öztürk et al.11 had demonstrated that band cementation had moderate genotoxic and cytotoxic effects, we applied bonded tubes to the first and second molars.
While orthodontic fixed attachments bonded with different types of adhesives showed no genotoxic effects during the 6-month periods, cytotoxic effects manifesting in terms of elevated BN and KL levels were observed. This finding may be attributed to the effect of adhesive contents and the properties of metallic appliances, either individually or in combination. The primary components of Transbond XT and Kurasper F are bis-GMA and TEGDMA. GrenGloo consists of uncured methacrylate ester monomers. Bis-GMA, TEGDMA, UDMA, and 2-hydroxyethyl methacrylate (HEMA) have been tested as resin components in the literature. Kleinsasser et al.9 reported that TEGDMA, UDMA, and HEMA induced significant increases in the rates of DNA migration in the Comet assay, a possible sign of genotoxic effects in human salivary glands and lymphocytes. Schweikl et al.22 reported that HEMA, GMA, and TEGDMA significantly elevated the number of MNs, whereas bis-GMA and UDMA only slightly elevated the number of MNs. Researchers have directly applied different types of monomers in mammalian cells in vitro. In contrast, in our study, these monomers were applied in vivo in composite form with different additives, such as photoinitiators, ultraviolet absorbers, and photostabilizers. This composite form could be a factor in causing reduction in the release of monomers.
Differences in BN growth between the time points T4 and T1 and between T4 and T2 were statistically significant in all the adhesive groups examined. Similarly, a statistically significant increase in the frequency of KL was observed, except in the Transbond XT group in the T1-T4 time period. Additionally, differences in the frequency of KL between the time points T4 and T2 and between T3 and T4 were only significant in the GreenGloo group. We speculate that GreenGloo may have a slightly greater cytotoxic effect than the other adhesives because of the presence of uncured methacrylate ester monomers. Unreacted methacrylate monomers, such as HEMA, UDMA, and Bis-GMA, are dissolved in the lipid bilayers of cell membranes. Since these hydrophobic monomers are often associated with HEMA, which increases the hydrophilic properties of the compound, these monomers can diffuse easily in the cell. Under existing circumstances, the hydrophobic monomers can attach and cause damage to the cells.23 The next step in the cellular process may be MN formation as a consequence of DNA fragmentation.25
A combination of the aforementioned monomers is typically used in the preparation of orthodontic adhesives. The materials tested in this study include largely similar resin matrices. However, Transbond XT also contains bis-EMA, which in monomeric form, has been reported to show a cytotoxic effect analogous to that of TEGDMA.26 Malkoc et al.8 examined five different adhesives and concluded that light-cured orthodontic-bonding adhesives showed significantly higher biocompatibility than other dental adhesives.6,7 A mixture of orthodontic adhesive monomers and a high degree of curing may have led to these results.
Corrosion-induced release of cobalt and nickel ions from metallic brackets and arch wires has been reported to cause DNA fragmentation in the buccal mucosa.20 Acid production from microorganisms, plaque, hard calcified deposits, and certain physicochemical events, such as varying chloride combinations and elevated levels of oxygen in saliva, are extremely corrosive to orthodontic appliances in the oral cavity.27 Several studies18,19 that metallic ions were released during the first 4-5 months of orthodontic treatments and subsequently moved into systemic transportation. However, the concentration of ions present in saliva or blood samples was significantly below their corresponding average dietary consumption and did not approach toxic levels.18,19 Therefore, we investigated the cytotoxic effects of fixed orthodontic treatment during a 6-month period, an adequate time interval for obtaining reliable results.
Baraba et al.28 reported that in vitro alterations induced by dental materials on human leukocytes are reversible in the long term. Similarly, it was shown that fixed orthodontic treatment in a minimum 1-year follow-up period did not cause apparent mutagenic or cytotoxic effects in oral mucosa cells.13 In our study, we observed cytotoxic changes during the 6-month period and observed no genotoxic effects based on MN formation, perhaps because the epithelial cells of the oral mucosa have the capacity of rapid turnover and regeneration (in general, in every 7 to 14 days). Therefore, the deleterious effects of monomers may not remain at the end of the study.
There has been some controversy about the effects of tobacco smoke and alcohol consumption on MN formation. For instance, the vast majority of participants consumed alcohol and tobacco in a study, which hindered researchers in elucidating the effects of individual variables.29 In another study, no genotoxic results associated with alcohol consumption were detected.30 In contrast, Schweikl and colleagues22 detected a synergistic effect between alcohol and nicotine. For this reason, we preferred to select nonsmoking and nondrinking individuals for this study.
To accurately assess the results, we selected patients who had no fillings or caries. In addition, none used oral antiseptic solutions; Erdemir et al.31 reported the presence of possible cytotoxic effects of the three commercial mouth rinses on gingival epithelial cells. Since viruses and variations in the immune system were potentially associated with an increased frequency of chromosome aberrations,32 subjects with repeated aphtous stomatitis or skin reactions were also excluded from the study. Furthermore, age-matched participants were recruited in order to control for age-related development of the MN.32
We evaluated the potential effect of radiation exposure on the buccal mucosa. Several previous studies have shown that radiation stimulated genotoxic effects on oral mucosa cells.33 Other studies reported that although radiographic exposure did not cause MN formation in target buccal epithelium cells, it did elevate cytotoxicity after 10 days of exposure.34 Moreover, Moore et al.35 proved that the rate of MN frequency increased shortly after radiotherapy, subsequently returning to baseline after a 3-month period. Therefore, in the present study, collection of epithelium cell samples was initiated after approximately 90 days after X-ray exposure in order to minimize the impact of any confounding variables.
Research on genotoxicity and cytotoxicity in patients undergoing orthodontic therapy can provide invaluable information concerning the carcinogenicity of orthodontic adhesives.
Figures and Tables
Figure 3
Cytotoxicity evaluation in this study. A, Binucleated cell; B, karyolysis and cell death; C, binucleated cell and karyorrhexis stained with acridine orange (×1,000).
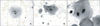
ACKNOWLEDGEMENTS
We thank Dr. Harika G. G. Bag, Assistant Professor, Department of Biostatistics, Inonu University, for her skillful assistance with the statistical analysis.
References
1. Malkoç S, Uysal T, Uşümez S, Işman E, Baysal A. In-vitro assessment of temperature rise in the pulp during orthodontic bonding. Am J Orthod Dentofacial Orthop. 2010; 137:379–383.


2. Huang FM, Chou MY, Chang YC. Dentin bonding agents induce c-fos and c-jun protooncogenes expression in human gingival fibroblasts. Biomaterials. 2003; 24:157–163.


3. Spahl W, Budzikiewicz H, Geurtsen W. Determination of leachable components from four commercial dental composites by gas and liquid chromatography/mass spectrometry. J Dent. 1998; 26:137–145.


4. Söhoel H, Gjerdet NR, Hensten-Pettersen A, Ruyter IE. Allergenic potential of two orthodontic bonding materials. Scand J Dent Res. 1994; 102:126–129.


5. Tadin A, Galic N, Mladinic M, Marovic D, Kovacic I, Zeljezic D. Genotoxicity in gingival cells of patients undergoing tooth restoration with two different dental composite materials. Clin Oral Investig. 2014; 18:87–96.


6. Koulaouzidou EA, Helvatjoglu-Antoniades M, Palaghias G, Karanika-Kouma A, Antoniades D. Cytotoxicity of dental adhesives in vitro. Eur J Dent. 2009; 3:3–9.


7. Ulker HE, Sengun A. Cytotoxicity evaluation of self adhesive composite resin cements by dentin barrier test on 3D pulp cells. Eur J Dent. 2009; 3:120–126.
8. Malkoc S, Corekci B, Ulker HE, Yalçin M, Sengün A. Cytotoxic effects of orthodontic composites. Angle Orthod. 2010; 80:571–576.


9. Kleinsasser NH, Schmid K, Sassen AW, Harréus UA, Staudenmaier R, Folwaczny M, et al. Cytotoxic and genotoxic effects of resin monomers in human salivary gland tissue and lymphocytes as assessed by the single cell microgel electrophoresis (Comet) assay. Biomaterials. 2006; 27:1762–1770.


10. Schweikl H, Schmalz G, Weinmann W. The induction of gene mutations and micronuclei by oxiranes and siloranes in mammalian cells in vitro. J Dent Res. 2004; 83:17–21.


11. Öztürk F, Yüksel Ş, Toy E, Kurtoğlu EL, Küçük EB. Genotoxic effects of banding procedure with different orthodontic cements on human oral mucosa cells. Turk J Med Sci. 2012; 42:Suppl 1. 1157–1165.
12. Angelieri F, Carlin V, Martins RA, Ribeiro DA. Biomonitoring of mutagenicity and cytotoxicity in patients undergoing fixed orthodontic therapy. Am J Orthod Dentofacial Orthop. 2011; 139:4 Suppl. e399–e404.


13. İz SG, Gürhan SİD, Şen BH, Endoğan T, HasircI N. Comparison of in vitro cytotoxicity and genotoxicity of MMA-based polymeric materials and various metallic materials. Turk J Med Sci. 2010; 40:905–916.
14. Faccioni F, Franceschetti P, Cerpelloni M, Fracasso ME. In vivo study on metal release from fixed orthodontic appliances and DNA damage in oral mucosa cells. Am J Orthod Dentofacial Orthop. 2003; 124:687–693. discussion 693-4.


15. Tolbert PE, Shy CM, Allen JW. Micronuclei and other nuclear anomalies in buccal smears: methods development. Mutat Res. 1992; 271:69–77.


16. Gioka C, Bourauel C, Hiskia A, Kletsas D, Eliades T, Eliades G. Light-cured or chemically cured orthodontic adhesive resins? A selection based on the degree of cure, monomer leaching, and cytotoxicity. Am J Orthod Dentofacial Orthop. 2005; 127:413–419. quiz 516.


17. Reichl FX, Durner J, Hickel R, Kunzelmann KH, Jewett A, Wang MY, et al. Distribution and excretion of TEGDMA in guinea pigs and mice. J Dent Res. 2001; 80:1412–1415.


18. Ağaoğlu G, Arun T, Izgi B, Yarat A. Nickel and chromium levels in the saliva and serum of patients with fixed orthodontic appliances. Angle Orthod. 2001; 71:375–379.
19. Kocadereli L, Ataç PA, Kale PS, Ozer D. Salivary nickel and chromium in patients with fixed orthodontic appliances. Angle Orthod. 2000; 70:431–434.
20. von der Hude W, Kalweit S, Engelhardt G, McKiernan S, Kasper P, Slacik-Erben R, et al. In vitro micronucleus assay with Chinese hamster V79 cells - results of a collaborative study with in situ exposure to 26 chemical substances. Mutat Res. 2000; 468:137–163.


21. Stich HF, Rosin MP. Quantitating the synergistic effect of smoking and alcohol consumption with the micronucleus test on human buccal mucosa cells. Int J Cancer. 1983; 31:305–308.


22. Schweikl H, Schmalz G, Spruss T. The induction of micronuclei in vitro by unpolymerized resin monomers. J Dent Res. 2001; 80:1615–1620.


23. Santos RL, Pithon MM, Fernandes AB, Cabral MG, Ruellas AC. Biocompatibility of orthodontic adhesives in rat subcutaneous tissue. J Appl Oral Sci. 2010; 18:503–508.


24. Bakopoulou A, Mourelatos D, Tsiftsoglou AS, Giassin NP, Mioglou E, Garefis P. Genotoxic and cytotoxic effects of different types of dental cement on normal cultured human lymphocytes. Mutat Res. 2009; 672:103–112.


25. Arossi GA, Lehmann M, Dihl RR, Reguly ML, de Andrade HH. Induced DNA damage by dental resin monomers in somatic cells. Basic Clin Pharmacol Toxicol. 2010; 106:124–129.


26. Geurtsen W, Lehmann F, Spahl W, Leyhausen G. Cytotoxicity of 35 dental resin composite monomers/additives in permanent 3T3 and three human primary fibroblast cultures. J Biomed Mater Res. 1998; 41:474–480.


27. de Soet JJ, Gruythuysen RJ, Bosch JA, van Amerongen WE. The effect of 6-monthly application of 40% chlorhexidine varnish on the microflora and dental caries incidence in a population of children in Surinam. Caries Res. 2002; 36:449–455.


28. Baraba A, Zelježić D, Kopjar N, Mladinić M, Anić I, Miletić I. Evaluation of cytotoxic and genotoxic effects of two resin-based root-canal sealers and their components on human leucocytes in vitro. Int Endod J. 2011; 44:652–661.


29. Ghose UR, Parida BB. Cytological study of exfoliated buccal mucosa cells of tribes in Orissa State (India) with high risk for oral cancer. Indian J Cancer. 1995; 32:95–99.
30. Burgaz S, Işcan A, Büyükbingöl ZK, Bozkurt A, Karakaya AE. Evaluation of micronuclei in exfoliated urothelial cells and urinary thioether excretion of smokers. Mutat Res. 1995; 335:163–169.


31. Erdemir EO, Sengün A, Ulker M. Cytotoxicity of mouthrinses on epithelial cells by micronucleus test. Eur J Dent. 2007; 1:80–85.


32. Xu GL, Bestor TH, Bourc'his D, Hsieh CL, Tommerup N, Bugge M, et al. Chromosome instability and immunodeficiency syndrome caused by mutations in a DNA methyltransferase gene. Nature. 1999; 402:187–191.


33. Cerqueira EM, Meireles JR, Lopes MA, Junqueira VC, Gomes-Filho IS, Trindade S, et al. Genotoxic effects of X-rays on keratinized mucosa cells during panoramic dental radiography. Dentomaxillofac Radiol. 2008; 37:398–403.

