Abstract
Objective
To determine the interleukin (IL)-6 levels in gingival crevicular fluid (GCF) of patients with severe root resorption after orthodontic treatment and investigate the effects of different static compressive forces (CFs) on IL-6 production by human periodontal ligament (hPDL) cells and the influence of IL-6 on osteoclastic activation from human osteoclastic precursor (hOCP) cells in vitro.
Methods
IL-6 levels in GCF samples collected from 20 patients (15 and 5 subjects without and with radiographic evidence of severe root resorption, respectively) who had undergone orthodontic treatment were measured by ELISA. The levels of IL-6 mRNA in hPDL cells and IL-6 protein in conditioned medium after the application of different uniform CFs (0, 1.0, 2.0, or 4.0 g/cm2 for up to 72 h) were measured by real-time PCR and ELISA, respectively. Finally, the influence of IL-6 on mature osteoclasts was investigated by using hOCP cells on dentin slices in a pit-formation assay.
Results
Clinically, the IL-6 levels were significantly higher in the resorption group than in the control group. In vitro, IL-6 mRNA expression significantly increased with increasing CF. IL-6 protein secretion also increased in a time- and magnitude-dependent manner. Resorbed areas on dentin slices were significantly greater in the recombinant human IL-6-treated group and group cultured in hPDL cell-conditioned medium with CF application (4.0 g/cm2) than in the group cultured in hPDL cell-conditioned medium without CF application.
Orthodontic tooth movement is achieved by repeated bone resorption on the pressure side and bone formation on the tension side. According to Krishnan and Davidovitch,1 the induction of tooth movement by orthodontic force application is characterized by remodeling changes in the dental and paradental tissues, including the dental pulp, periodontal ligament (PDL), alveolar bone, and gingiva. These tissues exhibit extensive macroscopic and microscopic changes upon exposure to mechanical force of varying magnitudes, frequencies, and durations.
Various cytokines, which are extracellular signaling proteins that act in an autocrine or paracrine fashion and at low concentrations on nearby target cells to provide cell-to-cell communications, affect bone metabolism and, thereby, orthodontic tooth movement. Interleukin (IL)-6 is a multifunctional cytokine that plays an important role in osteoclastic bone resorption.2 Kurihara et al.3 demonstrated that IL-6 can stimulate the formation of early osteoclastic precursor (OCP) cells. Further, Adebanjo et al.4 reported that IL-6 can activate mature osteoclasts. Moreover, IL-6 can stimulate osteoclastogenesis through stromal or osteoblastic cells.5 Alhashimi et al.6 reported the induction of IL-1β and IL-6, which peaked on day 3, on the pressure side after the application of force in vivo. Başaran et al.7 reported that the level of IL-6 in gingival crevicular fluid (GCF) increases during orthodontic tooth movement. These findings suggest that IL-6 may play important roles in bone resorption during orthodontic tooth movement.
Orthodontically induced inflammatory root resorption (OIIRR) is an unavoidable pathological consequence of orthodontic tooth movement. It can be defined as an iatrogenic disorder that unpredictably occurs after orthodontic treatment, wherein the resorbed apical root portion is replaced with normal bone. OIIRR is an extremely complex, sterile inflammatory process that involves various disparate components including mechanical force, teeth, bone, different types of cells, the surrounding matrix, and certain biological messengers.1,8 With regard to the relationship between OIIRR and IL-6, Hayashi et al.9 indicated that IL-6 is important for the induction and further processing of mechanically induced root resorption in rats. Yamaguchi et al.10 reported that stimulation with substance P increases the level of IL-6 in dental pulp fibroblasts obtained from patients with severe root resorption. Therefore, IL-6 may contribute to OIIRR. However, little is known about the relationship between OIIRR and IL-6 in humans.
The aims of this study were to determine the IL-6 levels in GCF of patients with severe root resorption after orthodontic treatment and investigate the effects of different static compressive forces (CFs) on IL-6 production by human PDL (hPDL) cells and the influence of IL-6 on osteoclastic activation from human OCP (hOCP) cells in vitro.
The subjects were 20 patients who had undergone treatment at the Department of Orthodontics, Nihon University School of Dentistry at Matsudo (Matsudo, Japan). The control group included 15 patients (2 men and 13 women; mean age, 28.0 ± 5.3 years; mean duration of treatment, 26.4 ± 3.1 months) without radiographic evidence of root resorption. The resorption group included 5 patients (5 women; mean age, 28.9 ± 6.1 years; mean duration of treatment, 27.8 ± 3.3 months) with radiographic signs of severe root resorption involving more than one-third of the original root length. The study was approved by the Ethics Committee of Nihon University School of Dentistry at Matsudo (EC 10-019). All the patients gave written informed consent for participation.
The inclusion criteria were as follows: Class I malocclusion with mild crowding (≤ 6 mm; mean arch length discrepancy, 5.4 ± 0.55 mm), extraction of all the four premolars, availability of excellent clinical records, and no history or evidence of tooth injury or wear. All the subjects were in good general health and had healthy periodontal tissues before the orthodontic treatment; the probing depths were less than or equal to 3 mm and periodontal bone loss was not evident radiographically. Patients were excluded if they had received antibiotic therapy during the orthodontic treatment or taken anti-inflammatory medication during the month preceding the start of the study.
The method used for GCF collection was as previously described by Yamaguchi et al.11 GCF was collected from the mesial and distal aspects of the maxillary central and lateral incisors of all the subjects, after debonding, by using filter paper strips (PerioPaper; Oraflow Inc., Smithtown, NY, USA) inserted 1 - 2 mm into the gingival sulcus for 60 s (Figure 1). After 1 min, the sampling was repeated. Care was taken to avoid mechanical injury to the soft tissue. The contents of the paper strips were eluted into 1× phosphate-buffered saline (PBS) containing a protease inhibitor (0.1 mM phenylmethylsulfonyl fluoride) and stored at -80℃ until analysis. The volume of GCF in each paper strip was measured with a Periotron 8000 meter (Oraflow Inc., Amityville, NY, USA).
For evaluating IL-6 expression, the paper strips were placed individually in 100 µL of PBS and vortexed thrice over 30 min. Each strip was then removed and the eluate was centrifuged for 5 min at 3,000 g. The supernatants were separated and frozen at -30℃ for later use. The protein concentration in the extract was estimated by using bovine serum albumin as a standard.
The Yamaguchi et al.12 method was used to prepare hPDL fibroblasts. In brief, hPDL tissues were obtained from the roots of premolars extracted from 6 healthy young patients (3 male and 3 female adolescents; 14 - 16 years of age) during the course of orthodontic treatment. The experimental protocol was approved by the Ethics Committee of Nihon University School of Dentistry at Matsudo (EC 10-019), and the parents or guardians of all the patients gave written informed consent for the use of the tissues.
The specimens were placed in 35-mm tissue culture dishes and covered with sterilized glass coverslips. The cells were maintained in minimum essential medium (α-MEM; Wako Pure Chemical Industries Ltd., Osaka, Japan) supplemented with 100 µg/mL of penicillin-G (Sigma-Aldrich Inc., St. Louis, MO, USA), 50 µg/mL of gentamicin sulfate (Sigma-Aldrich Inc.), 0.3 µg/mL of amphotericin B (Flow Laboratories Inc., McLean, VA, USA), and 10% of fetal calf serum (FCS; Cell Culture Laboratories, Arthens, OH, USA). The cultures were maintained at 37℃ in a humidified incubator (Forma CO2 incubator MIP-3326; Sanyo Electric Co./Medical Systems Division, Tokyo, Japan) in the presence of 95% air and 5% CO2. The medium was changed twice weekly. When the cells reached confluence, they were detached with 0.05% trypsin (Gibco; Life Technologies, Grand Island, NY, USA) in PBS for 10 min and subcultured in culture flasks. The cells attached to the bottom of the flasks were discarded to avoid contamination by epithelial cells.
Purchased hOCP cells (Lonza, Walkersville, MD, USA) were seeded onto 16-well Lab-Tek chamber slides (Nunc; Thermo Fisher Scientific Inc., Lake Barrington, IL, USA) at a density of 1 × 104 cells/100 µL and cultured in commercial medium (Lonza) for 7 days. After the osteoclasts had matured, the medium was replaced with commercial medium containing recombinant human IL-6 (rhIL-6; 25 ng/mL) or hPDL cell-conditioned medium without or with CF (4.0 g), and the cells were incubated for another 72 h.
This in vitro experiment was performed as described by Yamaguchi et al.13 In brief, hPDL cells were preincubated for 1 h in culture medium containing 1% FCS. Then, a cell disk (90 mm in diameter) was placed over nearly confluent cell layers and pressed for up to 72 h with a glass cylinder containing lead granules that controlled the magnitude of CF (1.0, 2.0, or 4.0 g/cm2) applied. Previous studies have shown that compressive mechanical stress can be applied by the system utilized in the present experiment.12 Control cells were covered with thin glass plates without lead weights, which produced a CF of 0.032 g/cm2. The possibility that removal of the glass plates might generate static electricity, which could affect the cells, was controlled by using glass plates in both the groups (Figure 2).
Total ribonucleic acid (RNA) was isolated from hPDL cells cultured with or without static CF application by using an RNeasy mini kit (Qiagen Co., Tokyo, Japan) according to the manufacturer's protocol. Aliquots containing equal amounts of messenger RNA (mRNA) were subjected to real-time PCR. The mRNA was converted to cDNA by using a PrimeScript RT reagent kit (TaKaRa Bio Inc., Shiga, Japan). Real-time PCR amplification was performed by using SYBR Premix Ex Taq (TaKaRa Bio Inc.) in a thermal cycler (TP-800 Thermal Cycler Dice; TaKaRa Bio Inc.) according to the manufacturer's protocol. The PCR conditions were 95℃ for 3 s and 60℃ for 30 s for 40 cycles after a hot start, and measurements were taken at the end of the annealing step at 60℃ during each cycle. The primers for IL-6 and glyceraldehyde 3-phosphate dehydrogenase (GAPDH) (TaKaRa Bio Inc.) were designed with reference to the respective cDNA sequences. The primer sequences were as follows: IL-6, 5'-AAGCCAGAGCTGTGCAGATGAGTA-3' and 5'-TGTCCTGCAGCCACTGGTTC-3'; GAPDH, 5'-GCACCGTCAAGGCTGAGAAC-3' and 5'-TGGTGAAGACGCCAGTGGA-3'.
The real-time PCR analysis was repeated after 3, 6, 9, 12, and 24 h of CF application. The data were analyzed by using SmartCycler software (Multiplate RQ version 1.00; TaKaRa Bio Inc.). The levels of the PCR products were confirmed to be the same in the experimental groups; therefore, these levels were assumed to reflect the levels of the respective mRNAs.
To examine the in vitro production of IL-6 protein in response to CF, cultured hPDL cells were seeded in 10-cm dishes in FCS-free α-MEM and static CF (1.0, 2.0, or 4.0 g/cm2) was applied for up to 72 h as already described. Thereafter, the culture medium was collected for ELISA. The protein concentration in the culture medium was determined from a standard curve of IL-6 generated by using a human IL-6 ELISA kit (R&D Systems Co.).
The resorptive activity of mature rat osteoclasts formed in vitro was evaluated by their ability to form resorption pits on dentin slices (6 mm in diameter, 0.15 mm in thickness). After incubation for 10 days, samples were washed thrice with PBS. The slices were placed in 1 M NH4OH for 30 min, cleaned by ultrasonication to remove adherent cells, and then washed and dried. Thereafter, they were mounted onto stubs for scanning electron microscopy and sputter-coated with platinum or mounted on glass slides for light microscopy. The entire surface of each slice was examined by using a scanning electron microscope (JSM-6340F; JEOL, Tokyo, Japan). The resorbed area in a single well was determined by an image processing system (WinROOF; Mitani Corp., Tokyo, Japan).
All the subjects had minimal plaque accumulation and excellent gingival health throughout the study. The probing depths remained less than 3 mm throughout the experimental period, and bleeding on probing was absent.
The mean volumes of GCF collected were not significantly different between the resorption and the control groups (0.41 ± 0.05 and 0.43 ± 0.05 µL, respectively). ELISA revealed that the IL-6 concentration in the resorption group was significantly higher than that in the control group (Figure 3).
Real-time PCR analysis revealed that the expression of IL-6 mRNA significantly increased with increasing static CF and peaked at 6 h after the application of the force (p < 0.01, Tukey's test; Figure 4). Further, ELISA showed that the release of IL-6 protein from loaded hPDL cells increased in a time- and magnitude-dependent manner (p < 0.05, Tukey's test; p < 0.01, one-way ANOVA; Figure 5). In the pit-formation assay, resorbed areas on dentin slices were significantly greater in the rhIL-6-treated group and the group cultured in hPDL cell-conditioned medium with CF application than in the group cultured in hPDL cell-conditioned medium without CF application (p < 0.01; Figure 6).
GCF was first utilized by periodontists attempting to develop a diagnostic test for periodontal diseases. This fluid is an osmotically mediated transudate. Its aqueous component is derived mainly from serum; the constituents are derived from serum, gingival tissues through which the fluid passes, and bacteria present in the tissues and gingival sulcus.14 GCF was chosen for the present study because of its accessibility and the minimal risk or harm to the patients during collection.
Orthodontic forces induce the movement of PDL fluids and any cellular and biochemical products produced before the mechanical perturbation. During orthodontic treatment, the forces exerted produce distortion of the PDL extracellular matrix, resulting in alterations in the cellular shape and cytoskeletal configuration. Such events lead to the synthesis and presence of extracellular matrix components, tissue-degrading enzymes, acids, and inflammatory mediators in the deeper periodontal tissues; these agents induce cellular proliferation and differentiation and promote wound healing and tissue remodeling.15 Dudic et al.16 reported that the GCF composition changes during orthodontic tooth movement. The levels of inflammatory cytokines such as IL-1β, IL-6, and receptor activator of nuclear factor kappa-B ligand (RANKL) are elevated in GCF during orthodontic tooth movement.17-19 George and Evans20 also reported high levels of RANKL in the GCF of patients with root resorption. The present study demonstrated a significant difference in IL-6 protein levels in the GCF of subjects with and without severe root resorption. IL-6 might therefore increase or facilitate root resorption during orthodontic treatment. However, further studies in a large number of subjects are required to elucidate the role of IL-6 in root resorption.
To determine the source of IL-6, hPDL and hOCP cells were stimulated with IL-6. Remarkable production of IL-6 was found in hPDL cells, but the increase was minimal in hOCP cells (data not shown). Therefore, hPDL cells were considered the predominant source of IL-6 in this study. IL-6 is reportedly produced by fibroblasts.21 Oshiro et al.22 suggested that osteoblast/stromal cells and PDL fibroblasts are involved in supporting osteoclastic differentiation during tooth movement. Odontoclasts are thought to be derived from mononuclear precursors of the monocyte/macrophage lineage and demonstrate characteristics similar to osteoclasts.23
To investigate the mechanism responsible for the changes in IL-6 production in OIIRR, the levels of IL-6 mRNA expression and IL-6 protein secretion in hPDL cells stimulated by static CF were measured. The results indicated a significant magnitude-dependent increase in IL-6 mRNA expression at 6 h after the CF application. The release of IL-6 protein also increased in a time- and magnitude-dependent manner. These findings are supported by previous studies that showed that mechanical stress stimulates IL-6 production in hPDL cells. Yamamoto et al.24 reported that hydrostatic pressure also induces IL-6 production from these cells. Moreover, Lee et al.25 reported that a continuous static CF (1.76 g/cm2) induces the expression of the IL-6 gene. Koyama et al.26 reported that the expression of IL-6 increases depending on the strength and duration of the CF on osteoblastic Saos-2 cells.
Trans-signaling between the soluble IL-6 receptor (sIL-6R) and IL-6 is considered important for the expansion of osteoclastic bone resorption.27 The sIL-6R reportedly also triggers osteoclast formation.28 Moreover, Dovio et al.29 demonstrated that the serum sIL-6R levels are correlated with bone resorption. Koyama et al.26 reported that the expression of the sIL-6R increases by the application of CF in Saos-2 cells. Further studies are necessary to determine whether CF stimulates the sIL-6R in hPDL cells.
In the pit-formation assay, the resorbed area was significantly increased by the treatment with rhIL-6 or hPDL cell-conditioned medium with CF application. Kotake et al.27 reported that IL-6 stimulates osteoclast formation. Moreover, Hashizume and Mihara30 reported that IL-6 causes bone resorption by inducing osteoclast formation via the induction of RANKL in synovial cells. Taken together, these findings and the present results suggest that the increased IL-6 secretion from hPDL cells induced by excessive orthodontic force may activate osteo/odontoclastogenesis.
Whether the high level of IL-6 in GCF is maintained even after debonding is questionable. Yamaguchi et al.13 reported that compressed PDL cells obtained from tissues with severe external apical root resorption produce a large amount of RANKL and upregulate osteoclastogenesis. These results suggest that OIIRR is significantly correlated with individual patient susceptibility. Therefore, the basal level of IL-6 in GCF of patients with severe root resorption may be high. In addition, a large amount of IL-6 produced by CF may stimulate osteo/odontoclastogenesis and aggravate root resorption. Further investigations should be performed to clarify the mechanisms underlying OIIRR.
In this study, a high level of IL-6 was detected in the GCF of patients with severe root resorption after orthodontic treatment, suggesting that IL-6 may be a biomarker for root resorption. Furthermore, heavy CF induced IL-6 production and stimulated osteo/odontoclastogenesis, indicating that IL-6 may induce or facilitate OIIRR.
Figures and Tables
Figure 1
Sampling of gingival crevicular fluid from the mesial and distal aspects of the maxillary central incisors.
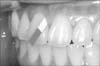
Figure 2
Illustration of the method used for uniform compressive force (CF) application in vitro. A confluent layer of cultured human periodontal ligament cells in one well of a six-well plate was compressed continuously by using a glass cylinder containing lead granules. The number of lead granules in the cylinder controlled the magnitude of CF applied.
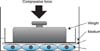
Figure 3
Concentrations of interleukin (IL)-6 protein detected by ELISA in gingival crevicular fluid samples from the control and resorption groups. The data represent means ± standard deviation. *p < 0.01 by Mann-Whitney U-test.
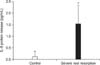
Figure 4
Relative levels of interleukin (IL)-6 mRNA expression determined by real-time PCR in human periodontal ligament cells subjected to different uniform compressive forces (0, 1.0, 2.0, or 4.0 g/cm2) for 24 h. The data represent means ± standard deviation of 4 independent experiments. *p < 0.01 by Mann-Whitney U-test and p < 0.01 by one-way ANOVA.
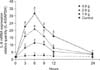
Figure 5
Levels of interleukin (IL)-6 protein release determined by ELISA using cultured human periodontal ligament cells subjected to different uniform compressive forces (0, 1.0, 2.0, or 4.0 g/cm2) for up to 72 h. The data represent means ± standard deviation of four independent experiments. *p < 0.01 by Mann-Whitney U-test and p < 0.01 by one-way ANOVA.
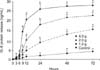
Figure 6
Percent resorbed areas on dentin slices determined by the pit-formation assay using human osteoclastic precursor cells cultured in commercial medium containing recombinant human interleukin (rhIL)-6 or human periodontal ligament (hPDL) cell-conditioned medium without or with uniform compressive force (CF) application (4.0 g). The data represent means ± standard deviation of four independent experiments. *p < 0.01 by Mann-Whitney U-test.
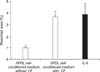
Notes
References
1. Krishnan V, Davidovitch Z. Cellular, molecular, and tissue-level reactions to orthodontic force. Am J Orthod Dentofacial Orthop. 2006; 129:469.e1–469.e32.


2. Ohsaki Y, Takahashi S, Scarcez T, Demulder A, Nishihara T, Williams R, et al. Evidence for an autocrine/paracrine role for interleukin-6 in bone resorption by giant cells from giant cell tumors of bone. Endocrinology. 1992; 131:2229–2234.


3. Kurihara N, Bertolini D, Suda T, Akiyama Y, Roodman GD. IL-6 stimulates osteoclast-like multinucleated cell formation in long term human marrow cultures by inducing IL-1 release. J Immunol. 1990; 144:4226–4230.
4. Adebanjo OA, Moonga BS, Yamate T, Sun L, Minkin C, Abe E, et al. Mode of action of interleukin-6 on mature osteoclasts. Novel interactions with extracellular Ca2+ sensing in the regulation of osteoclastic bone resorption. J Cell Biol. 1998; 142:1347–1356.


5. O'Brien CA, Gubrij I, Lin SC, Saylors RL, Manolagas SC. STAT3 activation in stromal/osteoblastic cells is required for induction of the receptor activator of NF-kappaB ligand and stimulation of osteoclastogenesis by gp130-utilizing cytokines or interleukin-1 but not 1,25-dihydroxyvitamin D3 or parathyroid hormone. J Biol Chem. 1999; 274:19301–19308.
6. Alhashimi N, Frithiof L, Brudvik P, Bakhiet M. Orthodontic tooth movement and de novo synthesis of proinflammatory cytokines. Am J Orthod Dentofacial Orthop. 2001; 119:307–312.


7. Başaran G, Ozer T, Kaya FA, Hamamci O. Interleukins 2, 6, and 8 levels in human gingival sulcus during orthodontic treatment. Am J Orthod Dentofacial Orthop. 2006; 130:7.e1–7.e6.


8. Meikle MC. The tissue, cellular, and molecular regulation of orthodontic tooth movement: 100 years after Carl Sandstedt. Eur J Orthod. 2006; 28:221–240.


9. Hayashi N, Yamaguchi M, Nakajima R, Utsunomiya T, Yamamoto H, Kasai K. T-helper 17 cells mediate the osteo/odontoclastogenesis induced by excessive orthodontic forces. Oral Dis. 2012; 18:375–388.


10. Yamaguchi M, Ozawa Y, Mishima H, Aihara N, Kojima T, Kasai K. Substance P increases production of proinflammatory cytokines and formation of osteoclasts in dental pulp fibroblasts in patients with severe orthodontic root resorption. Am J Orthod Dentofacial Orthop. 2008; 133:690–698.


11. Yamaguchi M, Yoshii M, Kasai K. Relationship between substance P and interleukin-1beta in gingival crevicular fluid during orthodontic tooth movement in adults. Eur J Orthod. 2006; 28:241–246.


12. Yamaguchi M, Ozawa Y, Nogimura A, Aihara N, Kojima T, Hirayama Y, et al. Cathepsins B and L increased during response of periodontal ligament cells to mechanical stress in vitro. Connect Tissue Res. 2004; 45:181–189.


13. Yamaguchi M, Aihara N, Kojima T, Kasai K. RANKL increase in compressed periodontal ligament cells from root resorption. J Dent Res. 2006; 85:751–756.


14. Lamster IB, Hartley LJ, Vogel RI. Development of a biochemical profile for gingival crevicular fluid. Methodological considerations and evaluation of collagen-degrading and ground substance-degrading enzyme activity during experimental gingivitis. J Periodontol. 1985; 56:11 Suppl. 13–21.


15. Kavadia-Tsatala S, Kaklamanos EG, Tsalikis L. Effects of orthodontic treatment on gingival crevicular fluid flow rate and composition: clinical implications and applications. Int J Adult Orthodon Orthognath Surg. 2002; 17:191–205.
16. Dudic A, Kiliaridis S, Mombelli A, Giannopoulou C. Composition changes in gingival crevicular fluid during orthodontic tooth movement: comparisons between tension and compression sides. Eur J Oral Sci. 2006; 114:416–422.


17. Uematsu S, Mogi M, Deguchi T. Interleukin (IL)-1 beta, IL-6, tumor necrosis factor-alpha, epidermal growth factor, and beta 2-microglobulin levels are elevated in gingival crevicular fluid during human orthodontic tooth movement. J Dent Res. 1996; 75:562–567.


18. Iwasaki LR, Haack JE, Nickel JC, Reinhardt RA, Petro TM. Human interleukin-1 beta and interleukin-1 receptor antagonist secretion and velocity of tooth movement. Arch Oral Biol. 2001; 46:185–189.


19. Nishijima Y, Yamaguchi M, Kojima T, Aihara N, Nakajima R, Kasai K. Levels of RANKL and OPG in gingival crevicular fluid during orthodontic tooth movement and effect of compression force on releases from periodontal ligament cells in vitro. Orthod Craniofac Res. 2006; 9:63–70.


20. George A, Evans CA. Detection of root resorption using dentin and bone markers. Orthod Craniofac Res. 2009; 12:229–235.


21. Chiu YC, Lin CY, Chen CP, Huang KC, Tong KM, Tzeng CY, et al. Peptidoglycan enhances IL-6 production in human synovial fibroblasts via TLR2 receptor, focal adhesion kinase, Akt, and AP-1-dependent pathway. J Immunol. 2009; 183:2785–2792.


22. Oshiro T, Shibasaki Y, Martin TJ, Sasaki T. Immunolocalization of vacuolar-type H+-ATPase, cathepsin K, matrix metalloproteinase-9, and receptor activator of NFkappaB ligand in odontoclasts during physiological root resorption of human deciduous teeth. Anat Rec. 2001; 264:305–311.


23. Sasaki T. Differentiation and functions of osteoclasts and odontoclasts in mineralized tissue resorption. Microsc Res Tech. 2003; 61:483–495.


24. Yamamoto T, Kita M, Kimura I, Oseko F, Terauchi R, Takahashi K, et al. Mechanical stress induces expression of cytokines in human periodontal ligament cells. Oral Dis. 2006; 12:171–175.


25. Lee YH, Nahm DS, Jung YK, Choi JY, Kim SG, Cho M, et al. Differential gene expression of periodontal ligament cells after loading of static compressive force. J Periodontol. 2007; 78:446–452.


26. Koyama Y, Mitsui N, Suzuki N, Yanagisawa M, Sanuki R, Isokawa K, et al. Effect of compressive force on the expression of inflammatory cytokines and their receptors in osteoblastic Saos-2 cells. Arch Oral Biol. 2008; 53:488–496.


27. Kotake S, Sato K, Kim KJ, Takahashi N, Udagawa N, Nakamura I, et al. Interleukin-6 and soluble interleukin-6 receptors in the synovial fluids from rheumatoid arthritis patients are responsible for osteoclast-like cell formation. J Bone Miner Res. 1996; 11:88–95.


28. Tamura T, Udagawa N, Takahashi N, Miyaura C, Tanaka S, Yamada Y, et al. Soluble interleukin-6 receptor triggers osteoclast formation by interleukin 6. Proc Natl Acad Sci U S A. 1993; 90:11924–11928.


29. Dovio A, Perazzolo L, Saba L, Termine A, Capobianco M, Bertolotto A, et al. High-dose glucocorticoids increase serum levels of soluble IL-6 receptor alpha and its ratio to soluble gp130: an additional mechanism for early increased bone resorption. Eur J Endocrinol. 2006; 154:745–751.


30. Hashizume M, Mihara M. The roles of interleukin-6 in the pathogenesis of rheumatoid arthritis. Arthritis. 2011; 2011:765624.