Abstract
Objective
To gain basic information regarding the biologic stability of plasma ion-implanted miniscrews and their potential clinical applications.
Methods
Sixteen plasma ion-implanted and 16 sandblasted and acid-etched (SLA) miniscrews were bilaterally inserted in the mandibles of 4 beagles (2 miniscrews of each type per quadrant). Then, 250 - 300 gm of force from Ni-Ti coil springs was applied for 2 different periods: 12 weeks on one side and 3 weeks contralaterally. Thereafter, the animals were sacrificed and mandibular specimens including the miniscrews were collected. The insertion torque and mobility were compared between the groups. The bone-implant contact and bone volume ratio were calculated within 800 µm of the miniscrews and compared between the loading periods. The number of osteoblasts was also quantified. The measurements were expressed as percentages and analyzed by independent t-tests (p < 0.05).
Orthodontic miniscrews can be inserted simply and produce tooth movement without the need for the patient's cooperation, which can increase the therapeutic effectiveness. Furthermore, numerous case reports and clinical studies have shown that movement beyond the limits of the conventional tooth movements can be achieved with these devices. Therefore, orthodontic miniscrews are widely used today.1,2
A problem associated with orthodontic miniscrews is loosening. Loosening occurs often during treatment, and the reported failure rate is 10 - 15%.3,4 This phenomenon is caused by complex internal and external factors that stem from the operator, patient, or miniscrew itself. With regard to patient and operator factors, Cheng et al.5 explained that loosening occurs because of the location of insertion and presence of keratinized tissue. Meanwhile, Büchter et al.6 and Joos et al.7 reported that the timing of force application and magnitude of force can affect the stability of miniscrews.
The initial stability of orthodontic miniscrews is an important factor that can affect the therapeutic success, help to prevent mobility within physiologic limits, and consequently, aid in new bone formation at the bone-miniscrew interface.8 Several studies are being performed to examine the effect of surface treatment on orthodontic materials, including TiN or ZrN coating by chemical vapor deposition (CVD), ion implantation, and physical vapor deposition (PVD). The temperature of the base metal is important for adequate hardness, sufficient tensile force of the coating layer, and excellent adhesive force between the base metal and the coating layer. High temperatures used in CVD can affect metal characteristics. Therefore, PVD and ion implantation are generally used; however, the latter method can achieve a higher adhesive force between the coating layer and the base material. Plasma ion implantation is used for corrosion and wear resistance because it can coat the material with a thin protective film. For example, Habig9 indicated that TiN coating of various dental metals by using this method improves wear and discoloration resistance as well as enhances mechanical characteristics. Further, Kim et al.10 reported that TiN-coated miniscrews have decreased corrosion current density and increased pitting resistance compared with noncoated miniscrews. These findings indicate that TiN coating by plasma ion implantation can improve the corrosion resistance and hardness of orthodontic miniscrews.
The aim of this study was to gain basic information regarding the biologic stability of plasma ion-implanted miniscrews and their potential clinical applications.
Miniscrews were placed in 4 beagle dogs. The experimental procedures were approved by the institutional review board of the animal experiment committee of Yonsei University Health Care System, Seoul, Korea (10-084).
Thirty-two self-drilling tapered miniscrews (1.45 mm in diameter and 6 mm in length; model 1O16106; Orlus Inc., Seoul, Korea) were used in this study; they are conventionally surface-treated by sandblasting and acid-etching (i.e., sandblasted and acid-etched [SLA] group). Sixteen of these miniscrews were further surface-treated by the plasma ion-implanting method (i.e., plasma ion-implanted group).
The animals were injected with 0.05 mg/kg of atropine subcutaneously; then, 2 mg/kg of xylazine and 10 mg/kg of ketamine were administered intravenously. General anesthesia was maintained with 2% enflurane. The insertion sites were infiltrated with lidocaine HCl (with 1:100,000 epinephrine) for local anesthesia and a 5 - 10 mm incision was made in the soft tissue. Then, the threads of the miniscrews were completely submerged in the alveolar bone under saline irrigation. Two plasma ion-implanted and 2 SLA miniscrews were bilaterally implanted in the mandible of every dog, between the roots of the second, third, and fourth premolars and the first molar. One of each type was placed adjacently per insertion area (Figure 1). The maximum insertion torque (Ncm) was measured by using a torque sensor (MGT50; Mark-10 Corporation, Copiague, NY, USA).
A Ni-Ti coil spring generating a force of 250 to 300 gm was connected to each miniscrew immediately after its insertion. The force was applied for 2 different periods according to the quadrant. On one side of the mandible, the force was continuously applied for 12 weeks after the miniscrew insertion; on the contralateral side, the miniscrews were inserted after 9 weeks from the beginning of the experiment and the force was applied for 3 weeks so that all the miniscrews could be removed simultaneously. Mobility was measured every 3 weeks by using the Periotest (Siemens AG, Munich, Germany). During the experimental period, a chlorhexidine rinse was used weekly to maintain oral hygiene.
The animals were sacrificed after 12 weeks of loading. The miniscrews were removed and specimens were collected for analysis. In brief, a low-speed diamond wheel was used to notch the head of the miniscrew before sectioning a block of mandibular bone including the miniscrew. This block was fixed in 10% formalin for 1 month and dehydrated in an ethanol series (70 - 100%) for 14 days. The samples were then embedded in polymethylmethacrylate by using a light curing and vacuuming machine. A diamond saw (Maruto, Tokyo, Japan) was used to bisect the blocks from the head to the apex by using the notch in the head of the miniscrew as a guide. A hard tissue-grinding system (Maruto) was used to create 100 - 110 µm sections, which were then stained with hematoxylin and eosin stain.
Histologic parameters were measured within 800 µm of the miniscrews by using a light microscope (×100 magnification). The bone-implant contact ratio was calculated by the sum of the regions where bone was in direct contact with the implant in proportion to the total length of the implant surface. To measure the bone volume ratio, a 10 × 10 grid was created from the implant-bone interface to 800 µm outward on the histologic sections. The numbers of squares filled and unfilled with bone were counted and their ratio was calculated. The number of osteoblasts was directedly counted in the same sections.
The data are presented as means and standard deviations. The Shapiro-Wilk and Kolmogorov-Smirnov tests were used for analyzing normality. Independent t-tests were used to compare the insertion torque and mobility between the groups and the bone-implant contact and bone volume ratio between the loading periods. p-values less than 0.05 were considered significant.
Of the 32 inserted miniscrews, the SLA miniscrews exhibited a 100% success rate. However, the plasma ion-implanted miniscrews had a success rate of 93.75% (one failure among 16 miniscrews).
The insertion torque depended upon the surface treatment. The SLA group showed an average insertion torque of 17.18 Ncm. Although the insertion torque in the plasma ion-implanted group was slightly higher, the difference was not significant (p = 0.61) (Figure 2, Table 1).
Miniscrew mobility was higher in the SLA group in the beginning. It demonstrated a continuous increase until week 12 and then decreased. In the plasma ion-implanted group, mobility increased up to week 6 and decreased in week 9, but increased again in week 12, showing no significant difference (p = 0.79) (Figure 3, Table 2).
Histologic analysis confirmed a bone-implant contact rate of 64.2% in the SLA group and 72.1% in the plasma ion-implanted group with 3 weeks of loading. Among the 12-week miniscrews, this rate was 63.4% in the plasma ion-implanted group and 66.2% in the SLA group. Although the bone-implant contact rates were slightly higher in the SLA group, no significant differences were noted (p = 0.11 in the 3-week group and p = 0.65 in the 12-week group) (Figure 4, Table 3).
The bone volume rate of the 3-week plasma ion-implanted miniscrews was 61.9%, which was slightly higher than that of the 3-week SLA miniscrews (52.9%). However, the 12-week SLA miniscrews showed a slightly higher bone volume rate (69.3%) than the 12-week plasma ion-implanted group (65.4%) (Table 3); these differences were not significant (p = 0.09 in the 3 week group and p = 0.32 in the 12-week group).
At 3 weeks of loading, the average number of osteoblasts in the SLA and plasma ion-implanted groups was 20.7 and 39.9, respectively. Among the 12-week miniscrews, they averaged 21.0 in the SLA group and 25.7 in the plasma ion-implanted group. There were similar numbers of osteoblasts in the 3 week SLA miniscrew group, 12 week Plasma miniscrew group, and 12 week SLA miniscrew group (Figure 5).
In 1981, Albrektsson et al.11 reported that many important factors influence the ideal fusion of an implant to the surrounding bone tissue: biocompatibility, design, surface quality, condition of the host tissue, surgical technique, and loading condition. Then, Han et al.12 reported that particle blasting can increase the implant surface area and eventually contribute to a wider bone contact area. These advantages, such as maximized bone contact area with surface-treated implants and maximized anchorage with coarse surfaces, can lead to higher success rates. Therefore, many implant manufacturers developed plasma-sprayed titanium. Several methods increase the implant contact surface with bone, but coarse surfaces produced by treatment with sulfuric or hydrochloric acid followed by coating with hydroxyapatite (HA) or other materials are seen frequently. Buser et al.13 reported that coarse surfaces, especially with HA coating, are good for bone deposition. However, the breakdown of coating materials and rough surfaces can become nesting areas for pathologic microorganisms. Despite these disadvantages, recent studies of the diverse biochemical bonding methods by using NaOH and heat treatment,14 anodic oxidation,15 and ion implantation16 have been conducted.
Ion implantation helps ions to permeate the thread surface without inducing changes in the design of the miniscrew. Beam-line ion implantation has been used by either rotating the implant or using several ion beam sources for uniform ion infiltration. Plasma ion implantation simplifies this complex process and allows a more uniform ion coating.16
Plasma ion implantation and PVD can allow surface coating at a comparatively low temperature, which prevents deformation of the base metal. However, PVD can cause coating fracture, delamination, and excessive wear because it coats materials that transform from soft to hard. In contrast, low-temperature ion implantation offers the advantage of a very thin nitrogen diffusion layer. In 1999, Wilson et al.17 reported that this treatment increases load support. Plasma ion implantation is favored among the diverse surface-treatment methods because of the assumption that it can achieve an increased bone fusion force with surface-treated dental implants.
In 2008, Kim et al.10 reported increased corrosion resistance and strength with less mechanical failures of miniscrews uniformly coated with ZrN and TiN compared with untreated miniscrews. An improvement in miniscrew strength can enhance the initial stability with less deformation of threads during insertion. Plasma ion implantation also offers the advantage of uniform coating of the miniscrew in a comparatively easy way. Furthermore, colors can be applied to distinguish clinical differences such as length and width or develop a miniscrew that matches the shade of the surrounding periodontal tissues.
During the current study, one plasma ion-implanted miniscrew failed, likely because of inflammation from poor plaque control in the implanted area. Further, the plasma ion-implanted miniscrews demonstrated a slightly higher insertion torque, without a significant difference; this result can be explained by the increased surface area due to the surface treatment.
Despite the fact that the histologic parameters showed no significant differences between the loading periods, the 3-week plasma ion-implanted miniscrews consistently had better results than the other groups, indicating that they are more favorable with regard to the initial stability. When examining the thread area near the apex before and after insertion by using an electron microscope in the 12-week groups, the plasma ion-implanted miniscrews exhibited less defective threads than the SLA miniscrews.
With further research by using more experimental animals, the size and length of the miniscrews can be varied to verify that plasma ion-implanted miniscrews provide superior initial stability. Additional research by using different types of surface treatments with different ions is required to determine whether other ion-treated surfaces demonstrate higher success rates. This information would be especially useful in the mandibular molar area, where the failure rate is higher, and provide valuable information for future orthodontic treatment. Furthermore, plasma ion-implanted miniscrews could be clinically relevant because colors could be added to differentiate screw length and diameter easily.
Figures and Tables
Figure 1
Images of a plasma ion-implanted miniscrew (A), an SLA miniscrew (B), and miniscrew insertion (C). After insertion, 250 - 300 gm of force was applied by using a Ni-Ti coil spring.
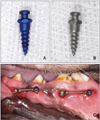
Figure 2
The insertion torque (Ncm) depending on the type of miniscrew. No significant difference was noted between the sandblasted and acid-etched (SLA) and the plasma ion-implanted miniscrews.
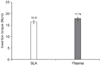
Figure 3
Change in mobility depending on the type of miniscrew. In the plasma ion-implanted group, the mobility increased up to week 6 and decreased in week 9, but increased again in week 12. In the sandblasted and acid-etched (SLA) group, the mobility increased continuously up to week 12 and then decreased.
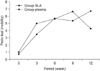
Figure 4
Histologic images (×100 magnification) of the bone-implant contact according to the loading period in each group. A, Plasma ion planted miniscrew (3 weeks). B, Plasma ion planted miniscrew (12 weeks). C, SLA miniscrew (3 weeks). D, SLA miniscrew (12 weeks).
SLA, Sandblasted and acid-etched.
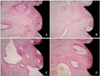
Figure 5
Quantification of osteoblasts depending on the type of miniscrew. The 3-week plasma ion-implanted miniscrews showed the highest number of osteoblasts.
SLA, Sandblasted and acid-etched.
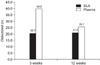
Notes
References
1. Wehrbein H, Merz BR, Diedrich P, Glatzmaier J. The use of palatal implants for orthodontic anchorage. Design and clinical application of the orthosystem. Clin Oral Implants Res. 1996. 7:410–416.


2. Park YC, Lee SY, Kim DH, Jee SH. Intrusion of posterior teeth using mini-screw implants. Am J Orthod Dentofacial Orthop. 2003. 123:690–694.


3. Miyawaki S, Koyama I, Inoue M, Mishima K, Sugahara T, Takano-Yamamoto T. Factors associated with the stability of titanium screws placed in the posterior region for orthodontic anchorage. Am J Orthod Dentofacial Orthop. 2003. 124:373–378.


4. Chen CH, Chang CS, Hsieh CH, Tseng YC, Shen YS, Huang IY, et al. The use of microimplants in orthodontic anchorage. J Oral Maxillofac Surg. 2006. 64:1209–1213.


5. Cheng SJ, Tseng IY, Lee JJ, Kok SH. A prospective study of the risk factors associated with failure of mini-implants used for orthodontic anchorage. Int J Oral Maxillofac Implants. 2004. 19:100–106.
6. Büchter A, Wiechmann D, Koerdt S, Wiesmann HP, Piffko J, Meyer U. Load-related implant reaction of mini-implants used for orthodontic anchorage. Clin Oral Implants Res. 2005. 16:473–479.


7. Joos U, Büchter A, Wiesmann HP, Meyer U. Strain driven fast osseointegration of implants. Head Face Med. 2005. 1:6.


8. Huja SS, Litsky AS, Beck FM, Johnson KA, Larsen PE. Pull-out strength of monocortical screws placed in the maxillae and mandibles of dogs. Am J Orthod Dentofacial Orthop. 2005. 127:307–313.


9. Habig KH. Friction and wear of sliding couples coated with TiC, TiN or TiB2. Surf Coat Technol. 1990. 42:133–147.


10. Kim SY, Moon YP, Park GH, Jo HH, Kim WG, Son MK, et al. Surface characteristics and electrochemical behaviors of TiN and ZrN coated orthodontic mini-screw. J Korean Ins Surf Eng. 2008. 41:232–239.


11. Albrektsson T, BråNemark PI, Hansson HA, Lindström J. Osseointegrated titanium implant. Requirements for ensuring a long-lasting, direct bone-to-implant anchorage in man. Acta Orthop Scand. 1981. 52:155–170.


12. Han CH, Johansson CB, Wennerberg A, Albrektsson T. Quantitative and qualitative investigations of surface enlarged titanium and titanium alloy implants. Clin Oral Implants Res. 1998. 9:1–10.


13. Buser D, Schenk RK, Steinemann S, Fiorellini JP, Fox CH, Stich H. Influence of surface characteristics on bone integration of titanium implants. A histomorphometric study in miniature pigs. J Biomed Mater Res. 1991. 25:889–902.


14. Kim HM, Miyaji F, Kokubo T, Nakamura T. Preparation of bioactive Ti and its alloys via simple chemical surface treatment. J Biomed Mater Res. 1996. 32:409–417.


15. Sul YT, Johansson CB, Jeong Y, Wennerberg A, Albrektsson T. Resonance frequency and removal torque analysis of implants with turned and anodized surface oxides. Clin Oral Implants Res. 2002. 13:252–259.

