Abstract
Objective
The aim of this study was to investigate and compare the in vivo effects of prostaglandin E2 (PGE2) administered by different methods on orthodontic tooth movement and bone metabolism macroscopically, histopatologically, and biochemically.
Methods
Forty-five young adult New Zealand rabbits were randomly divided into 3 experimental groups (n = 10/group), 1 positive control group (n = 10), and 1 negative control group (n = 5). The experimental rabbits were fitted with springs exerting 20-g reciprocal force on the maxillary incisors and PGE2 (10 µg/mL) was administered by the intravenous, submucosal, or intraligamentous route after appliance insertion and on days 1, 3, 7, and 14 thereafter. All rabbits were sacrificed on day 21 and their premaxillae were resected for histologic evaluation.
Remodeling of the surrounding alveolar bone and cellular changes in the periodontal ligament (PDL) underlie orthodontic tooth movement.1 Experimental studies on the histologic changes during tooth movement have been performed since Sanstedt's dog experiments in 1902.2 Simultaneous changes in both the chemical structure3 and the enzyme levels4 of periodontal tissues have also been reported, such as increased levels of prostaglandins (PGs),5,6 neuropeptides,7 cytokines and neurotransmitters,8 and leukotrienes.9 Increasing mechanical force to reduce the treatment time, a major problem in orthodontic practice, leads to several sequelae such as root resorption, PDL injury, and bone hyalinization. Although some authors have reported that orthodontic tooth movement is difficult to achieve by avoiding injury to the roots or alveolar bone,10 experimental studies have shown that rapid tooth movement is possible without such injury.6,7-9 These studies used PGs,6 cytokines,8 neuropeptides,7 leukotrienes,9 or nitrous oxide,11 which are considered physiologic mediators between the applied mechanical force and the cellular responses, to reduce tissue resistance during orthodontic tooth movement.
PGs are synthesized in almost all bodily tissues in response to physical, chemical, mechanical, immunologic, or neurohormonal stimuli. Two types of PGs that do not reach the systemic circulation but act only in the area of synthesis are PGEs and PGFs. PGE2 is the most active PG type in bone resorption.12 Several studies have shown that PGE2 has no systemic effects,5 and local application does not cause PDL injury.5,13,14 PGs, especially PGE2, are potent multifunctional regulators of bone metabolism.5 The anabolic activation of bone by PGE2 requires a mechanical stimulus. In vitro studies have demonstrated a stimulatory effect of PGE2 on bone resorption, and clinical investigation has shown focal concentrations of PGs in areas of bone resorption related to inflammation and other types of lesions.15 PGEs induce morphologic changes in osteoclasts and osteoblasts via increased intracellular levels of cAMP and calcium.16 Kanzaki et al.17 studied PGE2 synthesis in PDL cells undergoing mechanical stress and found a substantial increase in the control group. They also established that exogenous PGE2 increases the mRNA synthesis and protein secretion of the receptor activator of nuclear factor kappa-B ligand (RANKL). Therefore, these authors reported that the changes in PDL cells subjected to mechanical stress are PGE2 dependent.
The aim of the present study was to investigate and compare the in vivo effects of PGE2 administered by different routes on orthodontic tooth movement and bone metabolism macroscopically, histologically, and biochemically.
Forty-five adult male New Zealand rabbits (average body weight = 2.8 kg) were procured from the institutional Experimental Animal Research Center and randomly assigned to 3 experimental groups and 2 control groups (Table 1). No device or PGE2 was used in the negative control group (n = 5). In the positive control group (n =10), each animal was fitted with a spring exerting 20-g reciprocal force on the maxillary incisors, and PGE2 was not administered. The experimental animals (n = 10/group) were fitted with the same appliance and injected with 0.06-mL PGE2 (10 µg/mL) in the bilateral auricular veins (intravenous PGE2 group), submucosa immediately distal to the maxillary incisors (submucosal PGE2 group), or PDL surrounding the maxillary incisors (intraligamentous PGE2 group). PGE2 was administered after appliance insertion (day 0) and on days 1, 3, 7, and 14 thereafter (total dose = 1.2 µg) by using an insulin syringe or intraligamentous injector (Sopira Citoject; Heraeus Kulzer, Hanau, Germany).
The 0.06-mL injection volume was chosen because it corresponded to the minimal injection volume of the intraligamentous injector. PGE2 powder (p5640) was obtained from Sigma-Aldrich (St. Louis, MO, USA) and prepared as a 1 mg/mL stock solution by dissolving in ethanol. This solution was then diluted with a 2% lidocaine solution (2 mL lidocaine and 98 mL distilled water) containing 1:100,000 epinephrine to yield the 10 µg/mL PGE2 solution. This working solution was prepared immediately before each injection at the School of Pharmacy.
To apply the 20-g reciprocal force on the maxillary incisors, a round stainless steel wire (diameter = 0.2794 mm or 0.011 inches) was shaped into a spring with 13-mm-long arms extending bilaterally at 70° (Figure 1A). For appliance insertion, the experimental animals were anesthetized with 35 mg/kg ketamine (Ketalar®, Pfizer, New York, USA) and 2 mg/kg xylazine (Rompun®, Bayer, Leverkusen, Germany). The midpoint of the mesiodistal aspect of each incisor crown was marked, and a hole was bored into the crown in the vestibulopalatine direction at 1.5 - 2 mm from the palatine mucosa. To ensure correct alignment of the appliance without mucosal injury, each hole was bored parallel to the palatine mucosa. The free ends of the appliance were then bent distally and their tips were trimmed with cutting tongs (Figure 1B and 1C).
To evaluate the extent of tooth movement, the distance between the mesial surfaces of the maxillary incisors was measured with 0.01-mm precision electronic calipers on the day the rabbits were sacrificed.
Further, a 2-mL blood sample was drawn from the auricular vein of every animal immediately before sacrifice and centrifuged to collect serum for measuring the calcium and phosphorus levels.
The animals were sacrificed under anesthesia on day 21. Their premaxillae were removed and fixed in 10% formalin for 2 weeks; the appliances were maintained on the teeth during this procedure. After fixation, the tissues were decalcified in 5% nitric acid for 14 days. Then, 4-mm-thick slices were sectioned from each premaxilla, starting under the collum of the teeth, and embedded in paraffin. The paraffin blocks were stained with hematoxylin and eosin, and 40-µm sections were cut and examined by light microscopy.
The optical dissector method was used to determine the osteoblastic and osteoclastic populations in the sections. This method involves counting of units while advancing through virtual optical sections. Hundred 40-µm-thick sections were examined by starting with a random single-digit number and analyzing every 10th section thereafter; for example, beginning with section 7, sections 7, 17, and so on were examined until 10 sections were analyzed. The osteoblastic and osteoclastic counts in the tension and pressure sides, respectively, of these sections were calculated and multiplied by 10 to estimate the populations in each 4-mm-thick slice.
The SPSS 11.5 software program (IBM-SPSS Inc., Chicago, IL, USA) was used for statistical analyses. The Kolmogorov-Smirnov test was used to evaluate the data for normality. Because the assumption of normality was rejected (p < 0.005), the Kruskal-Wallis test was used to assess the differences among the groups. As significant differences were found, the Mann-Whitney U-test was used to determine the pairs of groups responsible for the significant differences. The data are presented as mean ± standard deviation.
Tooth movement was observed in all experimental groups (Table 2).
All pairs of groups except the positive control and intravenous PGE2 groups showed significant differences in this parameter (Table 3).
No significant differences in the initial serum calcium and phosphorus levels were observed among the groups (p > 0.05). However, the Mann-Whitney U-test revealed significant differences in the day-21 serum calcium levels between the negative control and the other groups. In particular, the differences between the negative control and the positive control, intravenous PGE2, and intraligamentous PGE2 groups were highly significant (p < 0.001), while the difference between the negative control and the submucosal PGE2 groups was less pronounced (p < 0.05).
The PDL width was within normal limits. Small foci of resorption and deposition on the alveolar bone and cement surfaces were observed. A few multinuclear osteoclasts and mononuclear osteoblasts were seen along the alveolar bone surface (Figure 2).
Narrowing of the PDL in the direction of tooth movement was observed. Neovascularization was noted in the PDL area adjacent to the alveolar bone. The new capillaries had irregularly formed endothelia and narrow lumens. The alveolar bone surface exhibited widespread resorption foci along which multinuclear osteoclasts were aligned. Both the focal resorption and the osteoclastic population were greater than those in the negative control group but less than those in the experimental groups. Resorption foci were also occasionally present in the cement surface adjacent to the PDL (Figure 3A and 3B).
Widening of the PDL was observed on the side opposed to the tooth movement. Capillaries with wide lumens and regular endothelia were seen in the PDL surface adjacent to the alveolar bone. The alveolar bone surface showed notably increased numbers of deposition foci and osteoblasts. The cement surface had increased resorption foci compared with that in the negative control group (Figure 3C and 3D).
The findings on this side were similar to those in the positive control group. However, a slightly higher number of osteoclasts and slightly intensive resorption foci were observed (Figure 4A and 4B).
The histologic changes on this side were also similar to those in the positive control group, although the numbers of osteoblasts and deposition foci were slightly increased (Figure 4C and 4D).
Marked histologic changes in the PDL and alveolar bone were noted on this side. Intense fibroblastic proliferation and a considerable increase in neovascularization in the PDL area adjacent to the alveolar bone were observed. Resorption foci in the alveolar bone were substantially increased and were surrounded by numerous osteoclasts. These changes were more marked than those in the control and intravenous PGE2 groups but were less intense than those in the intraligamentous group. However, resorption foci on the cement surface were not extensive (Figure 5A and 5B).
As with the pressure side, the tension side also showed a notable increase in the number of capillaries. Increased fibroblastic proliferation and bone deposition zones were obvious. The presence of young osteocytes in the new bone and a considerable increase in the number of osteoblasts along the alveolar bone surface were noted (Figure 5C and 5D).
The changes on this side were similar to but more intense than those in the submucosal PGE2 group. Considerable fibroblastic proliferation and very intense neovascularization were observed in the PDL area adjacent to the alveolar bone. Further, the extent of resorption and number of surrounding osteoclasts were substantially increased. Resorption foci on the cement surface were not extensive and were similar to those observed in the submucosal group (Figure 6A and 6B).
The changes on this side were also similar to but more marked than those in the submucosal PGE2 group. A clear increase in neovascularization and fibroblastic proliferation was seen in the PDL area adjoining the alveolar bone. Further, a marked increase in the number of osteoblasts was observed in the alveolar bone. The presence of young osteocytes in the new bone and large number of osteoblasts on its surface along the PDL were noted (Figure 6C and 6D).
Significant differences in the osteoblastic and osteoclastic populations were found in all comparisons (p ≤ 0.001). The intraligamentous PGE2 and negative control groups had the highest and lowest cell populations, respectively (Table 2).
Orthodontic tooth movement via the application of force proceeds by bone resorption and deposition. Various biochemical agents, including 1,25-dihydroxycholecalcife rol,18 nitrous oxide,11 thyroid hormones,19 cortisone,20 and PGs6,13,14,21-23 have been used to accelerate bone resorption and apposition. The idea of using PGs, especially PGE2, to accelerate tooth movement followed from the observation that the application of mechanical force stimulates the production of PGE2 in the PDL. Numerous studies have been conducted on the effect of exogenous PGE2 administered topically (submucosally) 13,14,21-23 or systemically (I.V.)24 on orthodontic tooth movement and bone metabolism. The results suggest that exogenous PGE accelerated orthodontic tooth movement by up to a factor of 2.5,6,21 However, a literature search failed to discover any report of intraligamentous administration of PGE2. The only study in which a biochemical agent was administered intraligamentally with the objective of accelerating orthodontic tooth movement was performed by Collins and Sinclair18 using Vitamin D.
In this study, the in vivo effects of different routes of PGE2 administration on orthodontic tooth movement and bone metabolism were investigated and compared by macroscopic, histologic, and biochemical evaluations. Intraligamentous and submucosal PGE2 administration substantially increased the osteoblastic and osteoclastic populations in the alveolar bone, considerably increasing orthodontic tooth movement; however, the intraligamentous route was more effective.
Rabbits were chosen as the experimental animals because of their tolerance of a 21-day experiment, ease of working in their mouths, sufficient PDL width for intraligamentous injection, and ease of intravenous injection via the auricular vein.
Many different doses of exogenous PGE2 have been used to study its effect on orthodontic tooth movement. Brudvik and Rygh23 used 0.1 mL of a 0.1 µg/mL PGE2 solution on days 0, 3, 5, and 7. Seifi et al.14 administered the same volume of a 1 mg/mL solution on days 0 and 7. Further, Boekenoogen et al.22 used doses of 0.1, 1.0, 5.0, and 10 µg. These doses were also administered by Leiker et al.,13 who reported that the 1 µg dose was the most effective for promoting orthodontic tooth movement and the higher doses increased the risk of root resorption. Moreover, they reported no difference in the effects between single and multiple injections. In the present study, 5 0.12-mL applications of the 10 µg/mL PGE2 solution were planned to isolate other environmental factors and better determine the effects of PGE2. Because the factor under investigation was the route of administration rather that the dose of PGE2, the same dose was administered in all experimental groups.
Local13 or intraligamentous25 administration of lidocaine has no negative effect on the periodontal tissues. Lidocaine might reduce the pain caused by the injection of PGE2, itself a pain mediator. The consensus in dentistry is that intraligamentous application of an active substance through a pressurized syringe is reliable and safe.25,26 The American Dental Association Council on Dental Materials, Instruments, and Equipment characterized intraligamentous injection as being "an effective method, sufficient for pulpal anesthesia in dental operations."25 Clinical studies have shown efficiencies of intraligamentous injection ranging from 81% to 98%.25 Although studies of its effects on the surrounding tissues have indicated that local tissue injury may occur,26 histologic examination has yielded little evidence of tissue injury and indicated reversible damage when injury occurs.27 Roahen and Marshall25 studied the effects of intraligamentous injection of 2% lidocaine containing 1:100,000 epinephrine on the pulp and periodontal tissues of the dog. They reported pulpal inflammation and external resorption foci on the root surface, both of which were reversible and most of which were no longer visible after 30 days. Further, Tagger et al.26 examined tooth movement following intraligamentous injection of a solution either with (1:100,000 epinephrine in 2% lidocaine and China ink) or without (3% mepivacaine and China ink) a vasoconstrictor in 12 dogs. They reported good penetration of China ink into the PDL and roots, with no apparent anatomic barriers, in both groups. They also observed that the ink diffused subperiosteally at the level of the alveolar bone crest, from where it proceeded along the vascular canals to reach the bone marrow.
The stereologic optical dissector method was used to determine the osteoblastic and osteoclastic populations. Stereology is the science of 3-dimensional interpretation of the properties of volume samples (e.g., biologic structures or metallurgical samples) based on data obtained from a 2-dimensional examination.28 Establishing the populations of units (e.g., osteoblasts and osteoclasts) within a biologic structure is indispensable to the examination of orthodontic tooth movement. Studies of such movement have used sections of 4 - 6 µm thickness.11,13,18 However, sections of this range can lead to incorrect counting of osteoblasts and osteoclasts, which have diameters of 10 to 40 µm. This is why sections of 40-µm thickness were used in the present study. A stereologic method was chosen to characterize the histologic findings of orthodontic tooth movement because of the conviction that this method would produce results with greater accuracy. This study is the first in the literature to use the optical dissector method for histologic examination of orthodontic tooth movement.
The extent of tooth movement is in agreement with previous findings.13,14 The Collins and Sinclair18 study with 1,25-dihydroxycholecalciferol seems to be the only study in which intraligamentous administration was used with the objective of increasing tooth movement. The authors reported that the application increased tooth movement without clinical side effects. Considering their and the present results, local administration of PGE2 increases tooth movement and intraligamentous administration is more effective than submucosal administration.
The histologic examination concerned the phenomena of resorption on the pressure side and deposition on the tension side, leading to tooth movement. The incisors of rodents such as rats and rabbits are subject to continuous enamel abrasion and eruption. Consequently, a physiologic remodeling process continually occurs in their alveolar sockets.1 In the present study, the low-level histologic changes observed in the negative control group were probably because of continuous enamel abrasion and eruption of the incisors.
PGE2 lacks systemic efficacy. One reason for this is the fact that the substance is produced within the same tissue and cannot be transported by the circulation because of its very short half-life.6 The intravenous administration of PGE2 had no significant effect on tooth movement macroscopically, although the results seemed to be better than those from the positive control group. One reason for this finding could be the direct release of PGE2, which is locally secreted and does not reach the arterial flow, into the circulation; however, despite this dilution, intravenous PGE2 administration achieved slight histologic effects. These results are compatible with those reported by Lee.24 The histologic changes were definitely marked in the submucosal and intraligamentous PGE2 groups than in the other groups, supporting the reports of increased histologic activity following local administration of PGE2.6,13,14,22 PDL cells and cells in the neighboring alveolar bone produce a considerable amount of PGE2 when subjected to mechanical stress.17 Intraligamentous application possibly supports a higher PGE2 concentration within the PDL than does submucosal administration, intensifying the histologic activity related to tooth movement. Limited resorption foci were found on the cement surface in all experimental groups and were not significantly different among these groups. These results are compatible with previous reports1,13,14,22,23 that exogenous PGE2 application during tooth movement could increase root resorption. In all of these reports, the authors indicated that the finding of increased root resorption in subjects treated with PGE2 application during orthodontic tooth movement, although not statistically significant, represents a risk; they also emphasized its reversibility. The consensus of studies of the histology of orthodontic tooth movement is that resorption foci, albeit limited, can be seen on the cement surface during every movement.1,6
PGs regulate bone metabolism locally and play an active role in mechanical stimulation-induced bone formation.5 Mechanical impacts are required for the anabolic activation of bone by PGE2. In vivo, PGE2 increases the populations of progenitor and mature osteoblasts.15 However, it accelerates bone resorption by increasing the osteoclastic population21 when a mechanical force is applied to bone. Therefore, the combined application of orthodontic force and exogenous PGE2 has a synergistic effect on alveolar bone, increasing not only bone resorption but also bone deposition.
The increase in the osteoblastic and osteoclastic populations in all experimental groups relative to the control groups was statistically significant, a finding compatible with earlier reports.2 In particular, these cell populations were substantially higher in the submucosal and intraligamentous PGE2 groups than in the intravenous PGE2 and control groups, a result that also agrees with previous findings.13,14,22 The more substantial increase in the cell populations in the intraligamentous PGE2 group may be attributable to both the increased PGE2 concentration in the PDL21,29 and the diffusion of the intraligamentally applied solution within the PDL,26 from where it passes subperiosteally at the level of the alveolar crest and proceeds along the vascular canals to reach and act directly on the bone marrow.
The number of blood vessels in the PDL increases with tooth movement.29 The greatest increase observed in the present study occurred in the intraligamentous PGE2 group, followed by the submucosal PGE2 group. This increase is caused by the inflammatory effects of PGEs on blood vessels.29 The most likely reason for the intense neovascularization in the intraligamentous PGE2 group is the more intense inflammation mediated by the increased concentration of PGE2 in the PDL.
Reitan30 reported that during orthodontic tooth movement, PGE2 increases the serum calcium concentration, which in turn plays an important role in the cellular response. Further, Yang et al.16 indicated that PGE2 affects bone resorption through increased cAMP and calcium levels, similar to the present findings. No differences in the serum calcium and phosphorous levels were found among the experimental groups, probably because a very low dose of PGE2 was applied and/or determination of the serum levels of these elements is a sensitive procedure. However, the extent of orthodontic tooth movement may not affect the serum calcium and phosphorus levels. Ersöz31 did not find significant differences in the serum calcium and phosphorus levels of rabbits subjected to forces of 20 and 60 g despite significant differences in the extent of tooth movement. The author proposed that the absence of a significant difference could be attributable to the fact that the area to which the force had been applied was a very small section of bone.
The intraligamentous route seems to be the most effective means of PGE2 administration to accelerate orthodontic tooth movement. However, detailed investigation of the effect of intraligamentous PGE2 administration on root resorption is required before widespread clinical application of this method.
Figures and Tables
Figure 1
The experimental appliance. Appearance of the spring (A), its insertion (B), and the subsequent tooth movement (C).
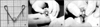
Figure 2
Hematoxylin and eosin-stained sample from the negative control group. Magnification: left, ×2.5; right, ×63. D, Dentin; PDL, periodontal ligament; AB, alveolar bone; O, osteocyte.
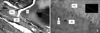
Figure 3
Hematoxylin and eosin-stained sample from the positive control group. The pressure (A, B) and tension (C, D) sides are shown. Magnification: A and C, ×2.5; B and D, ×63. D, Dentin; PDL, periodontal ligament; AB, alveolar bone; OB, osteoblast; OC, osteoclast; RF, resorption foci.
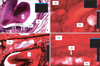
Figure 4
Hematoxylin and eosin-stained sample from the intravenous PGE2 group. The pressure (A, B) and tension (C, D) sides are shown. Magnification: A and C, ×2.5; B and D, ×63. D, Dentin; PDL, periodontal ligament; AB, alveolar bone; OB, osteoblast; OC, osteoclast; RF, resorption foci; MPS, median palatine suture.
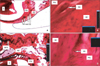
Figure 5
Hematoxylin and eosin-stained sample from the submucosal PGE2 group. The pressure (A, B) and tension (C, D) sides are shown. Magnification: A and C, ×2.5; B and D, ×63. D, Dentin; PDL, periodontal ligament; AB, alveolar bone; OB, osteoblast; OC, osteoclast; RF, resorption foci; C, capillary.
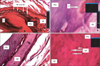
Figure 6
Hematoxylin and eosin-stained sample from the intraligamentous PGE2 group. The pressure (A, B) and tension (C, D) sides are shown. Magnification: A and C, ×2.5; B and D, ×63. D, Dentin; PDL, periodontal ligament; AB, alveolar bone; OB, osteoblast; OC, osteoclast; RF, resorption foci; MPS, median palatine suture; C, capillary.
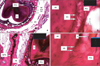
Notes
References
1. van de Velde JP, Kuitert RB, van Ginkel FC, Prahl-Andersen B. Histologic reactions in gingival and alveolar tissues during tooth movement in rabbits. Eur J Orthod. 1988. 10:296–308.


2. Persson M. A 100th anniversary: Sandstedt's experiments on tissue changes during tooth movement. J Orthod. 2005. 32:27–28.


3. Baumrind S, Buck DL. Rate changes in cell replication and protein synthesis in the periodontal ligament incident to tooth movement. Am J Orthod. 1970. 57:109–131.


4. Lilja E, Lindskog S, Hammarström L. Histochemistry of enzymes associated with tissue degradation incident to orthodontic tooth movement. Am J Orthod. 1983. 83:62–75.


5. Yamasaki K, Shibata Y, Imai S, Tani Y, Shibasaki Y, Fukuhara T. Clinical application of prostaglandin E1 (PGE1) upon orthodontic tooth movement. Am J Orthod. 1984. 85:508–518.


6. Yamasaki K, Shibata Y, Fukuhara T. The effect of prostaglandins on experimental tooth movement in monkeys (Macaca fuscata). J Dent Res. 1982. 61:1444–1446.


7. Vandevska-Radunovic V, Kvinnsland S, Kvinnsland IH. Effect of experimental tooth movement on nerve fibres immunoreactive to calcitonin gene-related peptide, protein gene product 9.5, and blood vessel density and distribution in rats. Eur J Orthod. 1997. 19:517–529.


8. Davidovitch Z, Nicolay OF, Ngan PW, Shanfeld JL. Neurotransmitters, cytokines, and the control of alveolar bone remodeling in orthodontics. Dent Clin North Am. 1988. 32:411–435.
9. Mohammed AH, Tatakis DN, Dziak R. Leukotrienes in orthodontic tooth movement. Am J Orthod Dentofacial Orthop. 1989. 95:231–237.


11. Akin E, Gurton AU, Olmez H. Effects of nitric oxide in orthodontic tooth movement in rats. Am J Orthod Dentofacial Orthop. 2004. 126:608–614.


12. Murray RK, Granner DK, Mayes PA, Rodwell VW. Chapter 16. Physiological İmportance of Lipids. Harper's Biochemistry. 1993. İzmir: BarışYayınevi.
13. Leiker BJ, Nanda RS, Currier GF, Howes RI, Sinha PK. The effects of exogenous prostaglandins on orthodontic tooth movement in rats. Am J Orthod Dentofacial Orthop. 1995. 108:380–388.


14. Seifi M, Eslami B, Saffar AS. The effect of prostaglandin E2 and calcium gluconate on ortho dontic tooth movement and root resorption in rats. Eur J Orthod. 2003. 25:199–204.


15. Reich KM, McAllister TN, Gudi S, Frangos JA. Activation of G proteins mediates flow-induced prosta glan din E2 production in osteoblasts. Endocrinology. 1997. 138:1014–1018.


16. Yang RS, Fu WM, Wang SM, Lu KS, Liu TK, Lin-Shiau SY. Morphological changes induced by prostaglandin E in cultured rat osteoblasts. Bone. 1998. 22:629–636.


17. Kanzaki H, Chiba M, Shimizu Y, Mitani H. Periodontal ligament cells under mechanical stress induce osteoclastogenesis by receptor activator of nuclear factor kappaB ligand up-regulation via prostaglandin E2 synthesis. J Bone Miner Res. 2002. 17:210–220.


18. Collins MK, Sinclair PM. The local use of vitamin D to increase the rate of orthodontic tooth movement. Am J Orthod Dentofacial Orthop. 1988. 94:278–284.


19. Soma S, Matsumoto S, Higuchi Y, Takano-Yamamoto T, Yamashita K, Kurisu K, et al. Local and chronic application of PTH accelerates tooth movement in rats. J Dent Res. 2000. 79:1717–1724.


20. Ashcraft MB, Southard KA, Tolley EA. The effect of corticosteroid-induced osteoporosis on orthodontic tooth movement. Am J Orthod Dentofacial Orthop. 1992. 102:310–319.


21. Saito M, Saito S, Ngan PW, Shanfeld J, Davidovitch Z. Interleukin 1 beta and prostaglandin E are involved in the response of periodontal cells to mechanical stress in vivo and in vitro. Am J Orthod Dentofacial Orthop. 1991. 99:226–240.


22. Boekenoogen DI, Sinha PK, Nanda RS, Ghosh J, Currier GF, Howes RI. The effects of exogenous prostaglandin E2 on root resorption in rats. Am J Orthod Dentofacial Orthop. 1996. 109:277–286.


23. Brudvik P, Rygh P. Root resorption aft er local injection of prostaglandin E2 during experimental tooth move-ment. Eur J Orthod. 1991. 13:255–263.


24. Lee WC. Experimental study of the effect of prostaglandin administration on tooth movement--with particular emphasis on the relationship to the method of PGE1 administration. Am J Orthod Dentofacial Orthop. 1990. 98:231–241.


25. Roahen JO, Marshall FJ. The effects of periodontal ligament injection on pulpal and periodontal tissues. J Endod. 1990. 16:28–33.


26. Tagger E, Tagger M, Sarnat H, Mass E. Periodontal ligament injection in the dog primary dentition: spread of local anaesthetic solution. Int J Paediatr Dent. 1994. 4:159–166.


27. Fuhs QM, Walker WA 3rd, Gough RW, Schindler WG, Hartman KS. The periodontal ligament injection: histological effects on the periodontium in dogs. J Endod. 1983. 9:411–415.


28. Odacı E, Yıldırım Ş, Bahadır A, Canan S, Şahin B, Baş O, et al. The possible error sources of new stereological methods and their solutions. Turkiye Klinikleri J Med Sci. 2004. 24:78–87.
29. Graber TM. Orthodontics: Current Principles and Techniques. 2000. 3rd ed. St. Louis: Mosby;193–257.
30. Reitan K. Clinical and histologic observations on tooth movement during and after orthodontic treatment. Am J Orthod. 1967. 53:721–745.


31. Ersöz M. The changes in the hormones playing a role in the bone resorption and deposition during orthodontic tooth movement [doctorate Tesis]. 2004. Erzurum: Atatürk University.