Abstract
Purpose
Zinc deficiency can induce serious clinical problems in the gastrointestinal (GI) system and immune system and can affect growth and development. It is more severe in younger patients. Chronic zinc deficiency is reflected more precisely in hair than in serum. We studied hair zinc levels and other hair and serum micronutrients in chronic malnourished children to identify which micronutrients are affected or correlated with the other ones.
Methods
Hair mineral analyses were performed in 56 children (age, 1-15 years) presenting with malnutrition, poor growth, poor appetite, anorexia, with/without other GI symptoms (diarrhea, abdominal pain, constipation) from August 2012 to March 2015. Biochemical studies for macronutrients and major micronutrients were also conducted.
Results
Hair zinc deficiency was diagnosed in 88%, and serum zinc deficiency was diagnosed in 55% of the children. There was no statistical correlation between serum and tissue zinc level. Hair zinc levels were highly correlated with serum vitamin D (r=−0.479, p=0.001), which also showed correlation with hair levels of magnesium and calcium. (r=0.564, 0.339, p=0.001, 0.011). Hair calcium level was correlated with serum pre-albumin (r=0.423, p=0.001). These correlations may explain the phenomenon that the major clinical manifestation of zinc deficiency is poor body growth. Clinical symptoms were resolved in most children after zinc supplementation.
According to the World Health Organization, malnutrition can be defined as “the cellular imbalance between supply of nutrients and energy and the body's demand for them to ensure growth, maintenance, and specific functions [1].” In addition to protein-energy malnutrition such as kwashiorkor and marasmus [2], children may be affected by micronutrient deficiencies, which also have a negative effect on growth and development. The most common and clinically significant micronutrient deficiencies in children are deficiencies of iron, iodine, zinc, and vitamin A [1].
The Academy of Nutrition and Dietetics and the American Society for Parenteral and Enteral Nutrition recently defined pediatric malnutrition (undernutrition) in the clinical setting as “an imbalance between nutrient requirements and intake that results in cumulative deficits of energy, protein or micronutrients that may negatively affect growth, development and other relevant outcomes [3].” This revised definition produces consideration on 5 aspects: anthropometrics, growth, chronicity, etiology, and impact on functional status.
Zinc is an essential micronutrient for physiologic metabolism. Zinc has 3 major basic functions: to catalyze enzyme activity, contribute to protein structure, and regulate gene expression [4]. Symptoms of zinc deficiency are nonspecific, such as growth retardation, delayed puberty, erectile dysfunction, diarrhea, alopecia, glossitis, nail dystrophy, decreased immunity, and hypogonadism in males [5]. The gastrointestinal (GI) tract is the major site for regulating zinc homeostasis, which adjustments are made in both zinc absorption and zinc elimination through feces [6]. Zinc concentration remains constant in hair, skin, heart and skeletal muscle, whereas that in plasma, liver, bone and testis easily fluctuates [7].
Over 25% of the world population has had risk of zinc deficiency [8]. There are only a few people in western or developed countries that suffer from zinc deficiency owing to their zinc-rich diet such as enhanced cereals, variant meats, and beans. On the other hand, in Asia or other developing countries, their traditional diet contains fewer zinc-rich meals, especially in infant foods. In addition, tests for zinc deficiency were not performed frequently, and zinc deficiency was only diagnosed by a small number of pediatricians concerned with zinc deficiency; therefore, there are more undiagnosed young patient than adults [8].
Hair mineral analysis is a major assessment method used in nutritional balancing. It is more recently being used to test for analyzing indicators of nutritional status. It has some advantages owing to its noninvasiveness, low cost and philosophical relevance of mineral balance. Hair mineral analysis does not measure the total body load of a mineral, but it infers information about the metabolism of minerals in cells. Hair mineral analysis represents an average rate of mineral accumulation in the sample for over 2-3 months before sampling [9].
We performed this study to investigate the prevalence rate of zinc deficiency from hair and serum levels, and that of other micronutrients deficiency in children presenting with delayed body growth, to identify which micronutrient deficiency affects or correlates with others, and to check the clinical improvement after supplementation of zinc in zinc-deficient children.
A total of 56 pediatric patients (28 males and 28 females) who visited the Department of Pediatrics at the Hanyang University Seoul Hospital between August 2012 and March 2015 for resolving their medical concerns such as malnutrition, poor growth, poor appetite, with/without other GI symptoms were included. Zinc deficiency was considered as the primary reason for their symptoms in 34 children. The 19 children with major malnutrition-inducing causes as well as zinc deficiency were excluded. These other causes which could lead to malnutrition were tuberculosis, heavy metal intoxication, severe anemia, child abuse and stresses. By laboratory data, zinc deficiency was defined as serum zinc level below 80 µg/dL [10] and/or tissue zinc level below 16 mg/dL. Children who had taken zinc supplements at diagnosis were followed-up in the out-patient department during this period.
This study was approved by the Institutional Review Board (IRB) of Hanyang University Seoul Hospital (IRB no. 2016-07-017).
Height was measured using manual scale stadiometer (Harpenden; Holtain, Crymych, UK) and weight, with a digital scale Health-o-Meter (DS-102; Jenix, Seoul, Korea) calibrated prior to each measurement. Body mass index (BMI) was calculated with known formula: BMI=weight/height2 (kg/m2).
The anthropometric percentiles were calculated using the 2007 Korean National Growth Charts [11].
The following evaluations were conducted: complete blood count, erythrocyte sedimentation rate, C-reactive protein levels, liver function tests, Mantoux test, iron, total iron-binding capacity, ferritin, thyroid function tests, total protein, albumin, calcium, phosphorus, alkaline phosphatase, zinc, vitamin D2, vitamin D3 electrolytes, immunoglobulins, urinalysis, stool examinations, Helicobacter pylori stool antigen, chest radiography, abdominal radiography, and wrist radiography. We investigated whether nutrient deficiency showed any correlation with hair mineral level.
Hair samples were from the occipito-nuchal region of the head, within 3 cm of the hair line. All samples were stored in chemical-free, polypropylene containers until analysis. Samples were washed according to the Trace Elements Inc. (Dallas, TX, USA) recommendations, that consists of washing each sample with acetone, water, dextran (1% v/v), and HNO3. The purpose of washing is to remove foreign materials, including oil and exogenous metals. After samples were washed, they were cut into small pieces with surgical scissors and dissolved in preconditioned acidic tube. The hair solution was analyzed by a Perkin-Elmer Mass Spectrometer (Sciex Elan 6100; Perkin-Elmer Corp., Foster City, CA, USA) [12]. All hair mineral levels were reported in mg/dL. With this method, it is possible to evaluate the hair content of calcium, magnesium, sodium, potassium, copper, zinc, phosphorus, iron, manganese, chrome, nickel, mercury, cadmium, lead, aluminum, arsenic, beryllium, antimony, barium, boron, cobalt, germanium, lithium, molybdenum, silver, selenium, silicon, stannum, vanadium, zirconium, platinum, titanium, tungsten, bismuth, rubidium, thallium, and uranium.
Zinc deficiency was diagnosed if serum or hair zinc levels were below normal range. We divided the children into two groups according to zinc deficiency: children with zinc deficiency and those without zinc deficiency. We compared basic anthropometric differences and clinical symptoms. We performed statistical analysis to identify the correlation between micronutrient level and zinc level of hair or serum.
Zinc supplementation was prescribed in the form of zinc sulfate monohydrate (0.5-1 mg of zinc/kg, 20 mg of zinc/10 mL solution) for two weeks to 23 of 34 zinc deficiency patients. In the children decreased serum zinc levels, serum zinc examinations were checked in two weeks, and for those who still showed decreased serum levels, repeat doses were prescribed. Clinical and anthropometric improvements were assessed in 2 months after the prescription of zinc supplementation, with checking improvements of their appetite, amount of meal intake, GI symptoms, and tiredness, and increase in weight or height.
The data were analyzed using mean±standard deviation and Spearman rank-order correlation coefficient IBM SPSS Statistics ver. 21.0 (IBM Co., Armonk, NY, USA). Spearman correlation assesses monotonic relationships (whether linear or not). In a sample with no repeated data values, a perfect Spearman correlation of +1 or −1 occurs when each of the variables is a perfect monotone function of the other. Therefore the Spearman correlation between two variables is expected to be high when observations have a similar (or identical for a correlation of 1) rank between the two variables, and low when observations have a dissimilar (or fully opposed for a correlation of −1) rank between the two variables. The standard score (z-score) is the signed number of standard deviations by which an observation or datum is above the mean. Z is calculated as χ−µ/σ (χ, raw score; µ, mean; σ, standard deviation). The absolute value of z represents the distance between the raw score and the population mean in units of the standard deviation. z is negative when the raw score is below the mean, positive when above. For all analyses, p<0.05 was considered statistically significant.
The general characteristics of the 56 subjects are listed in Table 1. Zinc deficiency was diagnosed in 88% (49/56) by hair analysis and 55% (31/56) by serum analysis among 56 subjects. Twenty-seven children showed zinc deficiencies both in hair mineral analyses and serum zinc levels. The mean ages of the children were younger in the zinc-deficient group (6.85±3.94 years) than the non-deficient group (7.05±4.42 years). There were more female children with zinc deficiency (male:female=16:18), but the sex ratio was inverse in children without zinc deficiency (male:female=12:10). The z-score of heights and weights were −0.79≤z≤2.78 and −0.61≤z≤ 3.43 in the zinc deficiency group and −0.73≤z≤ 2.18 and −1.00≤z≤2.90 in non-deficient group. Additionally, BMI z-scores were −0.93≤z≤3.06 in the zinc-deficient group and −0.96≤z≤2.26 in the non-deficient group. There was only minimal difference in height between the two groups (p>0.05). The serum and tissue zinc level were 74.86±7.37 µg/dL and 9.71±5.13 mg/dL in zinc deficiency group and 97.92±22.78 µg/dL and 10.64±4.38 mg/dL in the non-deficient group.
Clinical presentations of all subjects are shown in Table 2. There were no differences between the two groups. Both groups presented with poor weight and/or height gain and poor oral intake. However, GI symptoms were more common in among children with zinc deficiency (23.5% vs. 18.2%), with the rate of diarrhea and abdominal pain being very low in children with normal zinc levels (Table 2).
The zinc levels between serum and tissue had no statistical correlation (r=0.066, p=0.631), which meant there was no relationship between serum and hair zinc in the same individual (Fig. 1).
Hair zinc levels showed correlation with hair levels of magnesium (r=0.564, p<0.001) and calcium(r=0.339, p=0.011) with statistical significance, and with hair phosphorus despite no statistical significance (r=0.171, p=0.208). Hair zinc levels had a high negative-correlation with serum 25-hydroxy vitamin D (r=−0.479, p=0.001; Fig. 2).
We also investigated whether there may be correlations among the micronutrient levels measured in serum and hair such as pre-albumin, copper, hemoglobin, iron, protein, albumin, calcium, and phosphorus. There were correlations between serum pre-albumin level and tissue calcium (r=0.423, p=0.001), serum calcium and tissue phosphorus (r= −0.320, p=0.027), and serum pre-albumin and tissue phosphorus (r=0.114, p=0.408; Fig. 3).
Zinc-deficient children who took zinc supplements for two weeks (n=23) showed clinical improvements. They regained their body weight, grew more, ate well, and complained of no abdominal pain or diarrhea. Those with serum zinc deficiency achieved normal serum levels except 1 patient who took repeat dose after 2 weeks follow-up (Table 3).
The role of micronutrient deficiencies, especially zinc, in growth retardation has gained attention more recently [131415]. Attained height is a result of the interaction between genetic nature and nutritional availability during the growth period. Nutrition plays an important role in the control of linear growth through various mechanisms.
Studies performed on rats have shown results of decreased insulin-like growth factor I (IGF-I) concentrations with depletion of potassium, magnesium, or thiamine [16]. Vitamin D and calcium deficiencies also affect bone development, as manifested through rickets [17]. Deficiencies of other micronutrients such as iron, magnesium, and zinc may contribute indirectly to growth retardation by reducing the intake of other growth-limiting factors, such as energy and protein [1819]. In addition, several micronutrients, including zinc, iron, and vitamin A are associated with immune function and risk of morbidity, which affect growth in the long-term [20].
Zinc deficiency in rats causes not only growth retardation but also decreased concentrations of IGF-I plasma and growth hormone (GH) receptors [21]. Zinc deficiency is known to affect bone metabolism [22]. Zinc's contribution in growth may be explained through its participation in DNA synthesis [23]. Zinc has direct effects on the primary hormonal system (IGF-I/GH) that controls growth in the postnatal phase. This is when the majority of stunting occurs.
We should be cautious in interpreting the relation between serum zinc and tissue zinc, as both imply marginal stages of deficiency during different biological periods. Chronic zinc deficiency is thoroughly evaluated by hair zinc levels measurements. On the other hand, serum zinc levels properly assess current zinc storage in the body of a healthy subject [24].
Zinc was proven as an essential nutrient for rats and mice in the 1930s, for pigs in 1950s, and for humans in 1960s [25]. In the past 50 years, zinc's essential roles were discovered in biochemical and physiologic functions.
Zinc is ubiquitous in subcellular metabolism. Zinc is a catalyst for over 50 different enzymes [2]. Enzyme, classified as zinc metalloenzyme, decreases activity without effective form as zinc level, and enzyme activity can be recovered by restoring zinc storage level. Zinc plays a structural role in the form of zinc fingers [2]. Zinc fingers have 4 cysteines, which make up the general structure of the protein, and allows zinc to be bounded in a tetrahedral complex. Zinc fingers interact with variety of proteins, including those involved in cellular differentiation or proliferation, signal transduction, cellular adhesion, and transcription. The third basic function of zinc is regulation of gene expression [2]. Two basic components, consisting of a metal-binding transcription factor and a metal response element, are in the promoter of the regulated gene.
Physiologic signs of zinc depletion are explained with diverse biochemical functions rather than with a specific function, which makes it difficult to recognize biomarkers of zinc nutrition. Zinc is absorbed in the small intestine. Prolonged and severe decreases in zinc intake can significantly deplete the zinc stores in the body [26].
Although zinc is a dietary trace element, it is one of the most abundant element in the cell. Approximately 95% of the body zinc is in the cells. A complicated extensive system of cellular zinc homeostasis maintains cellular zinc concentrations within a narrow range [27]. Serum zinc level is not a reliable measure of zinc storage; therefore, it is not recommended for routine screening. A presumptive diagnosis of zinc deficiency should be taken in the context of zinc-deficiency symptoms, signs of malnutrition (e.g., underweight, hypoalbuminemia), or conditions commonly associated with zinc deficiency [4].
General mineral measurements include testing for contents in hair and body tissues such as liver, kidney, pancreas, blood and urine. These measurements show some differences [28]. Especially, studying hair mineral content is known to be a good method for measuring minerals absorbed in the body over a long period of time [29], and other reports say that it is inappropriate to choose blood as the sample because minerals stay in the blood circulation for a very short time [30]. Further, minerals absorbed in the body accumulate in various tissues through blood, which firmly connects with hair follicle proteins, as a result mineral content per body tissue (mass) can be accurately measured in hair [31]. Other study confirmed that there could be deficits of micronutrients in hair analysis which represents accumulative amount in body although micronutrients in blood were normal [32]. These factors which were described in previous studies supports our result that serum zinc level was not proportional to hair zinc level.
Vitamins can affect zinc status. Vitamin-induced suppression of zinc can occur either directly or indirectly. Vitamin D can suppress zinc absorption through increased absorption of calcium. Also, by decreasing thyroid activity and increasing parathyroid activity, vitamin D contributes to zinc deficiency by decreasing its absorption [33]. Similarly, in our study hair zinc level had negative correlationship to serum 25-hydroxy vitamin D.
Zinc could significantly affect magnesium absorption in a regular diet [34]. Even though it has been reported that magnesium is primarily absorbed in the distal intestine while zinc in the proximal parts of the intestine [3536], both minerals competed with the same transport mechanism in the small intestine. Calcium, especially in the presence of phytate, may interfere with zinc absorption [37]. Clinical data from balance and absorption studies in adults have been controversial with respect to adverse effects of calcium supplementation on zinc homeostasis [3839]. In our study, those two minerals (magnesium and calcium) were correlated to zinc in hair level. These results are different from previous studies, and could be attributed to different measuring methods (serum and hair).
Normally, zinc-supplementary therapy may be continued for 3 or 4 months. When initiated within 6 months after the onset of zinc deficiency, the positive response rate to therapy is 70% or higher. However, the response rate decreases if the therapy is delayed 6 months or longer, following the onset of zinc deficiency. Zinc overdose leads to inhibition of copper absorption in the small intestine, inducing copper deficiency, so occasional monitoring of serum zinc and copper is necessary during longterm zinc supplementation. Even though the therapy is considered as success, zinc supplementation sometimes can be continued for approximately 6 months or longer [40].
The limitations of our study are that it was a retrospective study and was performed in a small number of the subjects. Despite these limitations, we could gather diverse information concerning micronutrient deficiencies, especially zinc deficiency by hair mineral analysis and serum levels, and observe clinical improvements after zinc supplementation.
In summary, hair zinc and mineral analysis are useful to assess the clinical status nutrition-related problems in pediatric patients. Hair zinc levels were highly correlated with serum vitamin D, which also showed correlation with hair levels of magnesium and calcium, which are also essential nutrient for external growth. Additionally, clinical problems were improved in most children after zinc supplementation. There was no statistical correlation between serum and tissue zinc level. Chronic zinc deficiency was implied more precisely in hair zinc level than in serum.
Further studies are required to reestablish the mineral nutrition status of children who present with other symptoms and also to analyze other nutritional status by hair analysis.
Figures and Tables
Fig. 2
Hair zinc levels were highly correlated with serum 25-hydroxy vitamin D, which also showed correlation with hair levels of magnesium, calcium, and phosphorus. Zn: zinc, Mg: magnesium, Ca: calcium, P: phosphorus.
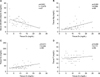
Fig. 3
Hair calcium, hair phosphorus, and serum pre-albumin were correlated with each other. Ca: calcium, P: phosphorus
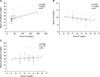
Table 1
Clinical Profiles and Anthropometric Data of Children with Zinc Deficiency and without Zinc Deficiency
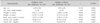
References
1. World Health Organization (WHO). Nutrition for health and development: a global agenda for combating malnutrition [Internet]. France: WHO;2000. cited 2016 Aug 9. Available from: http://apps.who.int/iris/bitstream/10665/66509/1/WHO_NHD_00.6.pdf.
2. Hendricks KM, Duggan C, Gallagher L, Carlin AC, Richardson DS, Collier SB, et al. Malnutrition in hospitalized pediatric patients. Current prevalence. Arch Pediatr Adolesc Med. 1995; 149:1118–1122.
3. Mehta NM, Corkins MR, Lyman B, Malone A, Goday PS, Carney LN, et al. Defining pediatric malnutrition: a paradigm shift toward etiology-related definitions. JPEN J Parenter Enteral Nutr. 2013; 37:460–481.
4. Cousins RJ. Zinc. In : Bowman BAB, Russell RM, editors. Present knowledge in nutrition. Washington, DC: ILSI Press;2006. p. 445–457.
5. King JC. Zinc. In : Shils ME, Shike M, editors. Modern nutrition in health and disease. 10th ed. Philadelphia: Lippincott Williams & Wilkins;2006. p. 271–285.
6. King JC, Shames DM, Woodhouse LR. Zinc homeostasis in humans. J Nutr. 2000; 130:5S Suppl. 1360S–1366S.


7. Tapiero H, Tew KD. Trace elements in human physiology and pathology: zinc and metallothioneins. Biomed Pharmacother. 2003; 57:399–411.


8. Maret W, Sandstead HH. Zinc requirements and the risks and benefits of zinc supplementation. J Trace Elem Med Biol. 2006; 20:3–18.


9. Wołowiec P, Michalak I, Chojnacka K, Mikulewicz M. Hair analysis in health assessment. Clin Chim Acta. 2013; 419:139–171.


10. Brown KH, Wuehler SE, Peerson JM. The importance of zinc in human nutrition and estimation of the global prevalence of zinc deficiency. Food Nutr Bull. 2001; 22:113–125.


11. Korea Centers for Disease Control and Prevention. Growth charts [Internet]. Cheongju: Korea Centers for Disease Control and Prevention;2007. cited 2016 Sep 13. Available from: http://cdc.go.kr/CDC/cms/cmsFile-Download.jsp?fid=28&cid=1235&fieldName=attachGrp&index=1.
12. Miekeley N, de Fortes Carvalho LM, Porto da Silveira CL, Lima MB. Elemental anomalies in hair as indicators of endocrinologic pathologies and deficiencies in calcium and bone metabolism. J Trace Elem Med Biol. 2001; 15:46–55.


13. Allen LH. Nutritional influences on linear growth: ageneral review. Eur J Clin Nutr. 1994; 48:Suppl 1. S75–S89.
14. Gibson RS, Hotz C. Martorell R, Haschke F, editors. Nutrition and growth. Philadelphia: Nestec Inc, Vevey/Lippincott, Williams & Wilkins;2001. p. 159–192.
15. Brown KH, Peerson JM, Rivera J, Allen LH. Effect of supplemental zinc on the growth and serum zinc concentrations of prepubertal children: a meta-analysis of randomized controlled trials. Am J Clin Nutr. 2002; 75:1062–1071.


16. Estívariz CF, Ziegler TR. Nutrition and the insulin-like growth factor system. Endocrine. 1997; 7:65–71.


18. Clausen T, Dørup I. Micronutrients, minerals and growth control. Bibl Nutr Dieta. 1998; (54):84–92.


19. Lawless JW, Latham MC, Stephenson LS, Kinoti SN, Pertet AM. Iron supplementation improves appetite and growth in anemic Kenyan primary school children. J Nutr. 1994; 124:645–654.


20. Rivera J, Martorell R. Nutrition, infection and growth. Part 1: effects of infection on growth. Clin Nutr. 1988; 7:156–162.
21. Dørup I, Clausen T. Effects of magnesium and zinc deficiencies on growth and protein synthesis in skeletal muscle and the heart. Br J Nutr. 1991; 66:493–504.


23. Loveridge N, Noble BS. Control of longitudinal growth: the role of nutrition. Eur J Clin Nutr. 1994; 48:75–84.
24. da Silva-Santana SC, da Silva Diniz A, de Feitas Lola MM, Oliveira RS, Silva SMM, de Oliveira SF, et al. Parameters of evaluation of zinc nutritional status: comparison between zinc hair rates and serum alkaline phosphatase in pre-scholars of the Municipality of João Pessoa, Paraíba. Rev Bras Saúde Mater Infant. 2002; 2:275–282.


25. O'Dell BL, Reeves PG. Zinc status and food intake. In : Mills CF, editor. Zinc in human biology. London: Springer-Verlag;1989. p. 173–181.
26. Cousins RJ. Systemic transport of zinc. In : Mills CF, editor. Zinc in human biology. New York: Springer-Verlag;1989. p. 79–93.
28. Delves HT. Assessment of trace element status. Clin Endocrinol Metab. 1985; 14:725–760.
29. Baumslag N, Yeager D, Levin L, Petering HG. Trace metal content of maternal and neonate hair. Zinc, copper, iron, and lead. Arch Environ Health. 1974; 29:186–191.
30. Petering HG, Yeager DW, Witherup SO. Trace metal content of hair. II. Cadmium and lead of human hair in relation to age and sex. Arch Environ Health. 1973; 27:327–330.
31. Son BS, Hong EJ, Kim YS. A study on trace metal levels in hair. Korean Ind Hyg Assoc J. 1997; 7:233–244.
32. Hong J, Lee JH, Lee R, Shin JY, Ko JS, Seo JK. Trace elements deficiency and the diagnostic usefulness of hair mineral analysis in children with chronic gastrointestinal disease. Korean J Pediatr Gastroenterol Nutr. 2008; 11:122–129.


33. Watts DL. The nutritional relationships of zinc. J Orthomol Med. 1988; 3:63–67.
34. Bohn T. Dietary factors influencing magnesium absorption in humans. Curr Nutr Food Sci. 2008; 4:53–72.


35. Hardwick LL, Jones MR, Brautbar N, Lee DB. Site and mechanism of intestinal magnesium absorption. Miner Electrolyte Metab. 1990; 16:174–180.
36. Hambidge KM, Miller LV, Tran CD, Krebs NF. Measurements of zinc absorption: application and interpretation in research designed to improve human zinc nutriture. Int J Vitam Nutr Res. 2005; 75:385–393.


37. Oberleas D, Muhrer ME, O'Dell BL. Dietary metal-complexing agents and zinc availability in the rat. J Nutr. 1966; 90:56–62.


38. McKenna AA, Ilich JZ, Andon MB, Wang C, Matkovic V. Zinc balance in adolescent females consuming a lowor high-calcium diet. Am J Clin Nutr. 1997; 65:1460–1464.