Abstract
The prevalence of obesity is increasing worldwide. Obesity can cause hyperlipidemia, hypertension, cardiovascular diseases, metabolic syndrome and non-alcoholic fatty liver disease (NAFLD). Many environmental or genetic factors have been suggested to contribute to the development of obesity, but there is no satisfactory explanation for its increased prevalence. This review discusses the latest updates on the role of the gut microbiota in obesity and NAFLD.
The prevalence of obesity is on the rise globally. It affected fewer than 10% of the general population in many countries before 1980, but today nearly half the people in many countries are overweight and 20-30% of those living in the member countries of the Organisation for Economic Co-operation and Development (OECD) are obese [1]. Similarly, obesity in pediatric and adolescent populations in Korea was more prevalent in the middle 2000s than it was in the late 1990s [2].
Obesity can cause hyperlipidemia, cardiovascular diseases, metabolic syndrome and non-alcoholic fatty liver disease (NAFLD). Obesity and associated chronic disorders that develop during childhood also can persist into adulthood [3]. There are thus many concerns about the public health impact of childhood obesity and its clinical consequences [4]. The prevalence of childhood obesity and associated metabolic disorders has been increasing in Korea [5,6].
Obesity develops primarily because energy intake exceeds energy expenditure, and many environmental and host factors interact in complex ways to contribute to its development [7]. Several genes contribute to weight gain by controlling appetite, energy expenditure and metabolism, but can only partially account for the development of obesity. Overall, the known contributing factors are limited in their ability to explain the rising prevalence of obesity [7].
A large number of microorganisms, collectively known as the "gut microbiota", live in the human intestine and their metabolic activities affect both the absorption of nutrients and energy homeostasis [8]. Many recent studies have reported that changes in the composition of the gut microbiota contribute to control of weight gain, development of obesity and related metabolic disorders [4,9-11]. This review discusses the relationships of the gut microbiota to obesity and NAFLD, and their proposed mechanisms.
There are more than 2,000 species of commensal bacteria, including anaerobes, within the adult human gut. The vast majority is in the large intestine, amount to about 1014 in number, and weigh about 1.5 kg [12]. The gut microbiota and humans have a mutualistic relationship. The bacteria obtain nutrients within the protected anoxic microenvironment of the human intestine. The host obtains vitamin K, benefits from the preservation and repair of the intestinal wall by the resident microbes, and gains carbon and energy that would otherwise not be available [13].
The fetal intestine is sterile. Microorganisms are introduced into the human gut after birth, and the microbial composition of the microbiota varies with delivery methods, feeding (i.e., breast vs. bottle), infant care environment and antibiotic use [14]. Our knowledge of the composition of the adult gut microbiota derives from microbial culture studies, but conventional culture techniques detect only a small number of the species of intestinal bacteria [15]. More recently, the diversity of gut microbiota has been revealed by genetic or metagenomic studies [16]. Metagenomic analysis and 16S ribosomal RNA gene sequencing, which is specific for prokaryotic ribosomes, have shown that Firmicutes, Bacteroidetes, Actinobacteria and Proteobacteria are the predominant bacterial phyla among the intestinal bacteria in adults [17,18]. Changes in the composition of the diverse gut microbiota (dysbiosis) are associated with several clinical conditions, including obesity, autoimmune diseases, and allergies [14].
Ley et al. [19] found, using 16S rRNA gene detection and analysis, that ob/ob mice had a 50% reduction in abundance of Bacteroidetes and a proportional increase in Firmucutes bacteria compared to lean sibling mice from C57BL/6J ob/+ mothers. As both groups of animals had been fed the same diet, the results indicated that obesity could affect the diversity of the gut microbiota. Obese human twins also have different gut microbiota composition compared to their lean twin, including reduced microbiological diversity and reduced levels of Bacteroidetes [20]. There are many animal and human studies of the relationships between gut microbial ecology and obesity or body weight [1,21]. Most have shown diverse changes in gut microbial composition with obesity, including a high ratio of Firmucutes to Bacteroidetes, known as an obese microbiota, which increases along with body weight or obesity. Obese children already have different gut microbiota compared to lean children [22-24]. This has led to suggestions that the composition of gut microbiota during early childhood is a predictor of obesity in later life, and that early modulation of the gut microbiota might prevent obesity [25,26]. How does the gut microbiota affect obesity?
First, the obese gut microbiota increases both the capacity to harvest energy from the diet and the accumulation of fat in adipose tissue and the liver by altering host metabolism. Studies in germ-free and conventionalized mice revealed that the microbiota promoted absorption of monosaccharides from the gut lumen and adipocyte hypertrophy by suppressing fasting-induced adipocyte factor (Fiaf) in the intestine, and suggest that the gut microbiota is an important environmental factor that affects energy harvest from the diet and energy storage in the host [13,27]. Germ-free mice were protected from diet-induced obesity by increased AMP-activated protein kinase (AMPK) activity. In conventionalized mice fed a high-fat, high sugar western diet, the gut microbiota suppressed AMPK activity in the gastrocnemius muscle and the liver [28]. Turnbaugh et al. [29] reported that the obese microbiome (i.e., the combined obese microbiota, their genetic elements and environmental interactions) has an increased capacity for dietary energy harvest because it is enriched in environmental gene tags encoding many enzymes involved in breaking down usually indigestible dietary polysaccharides.
Second, the gut microbiota regulates entero-endocrine cells, promoting the release of several gut hormones [30-33]. Gut microbiota-mediated increase in adiposity was blunted in conventionally raised G protein-coupled receptor 41 (Gpr41) deficient mice [30]. Gpr41 is a receptor for the binding of short chain fatty acids produced by fermentation of polysaccharides by gut microbes. According to Samuel et al. [30], expression of peptide YY (PYY) was lower in both Gpr41-deficient germ-free and conventionalized mice than in Gpr41 intact mice. Reduced expression of PYY resulted in increased intestinal transit time and reduced dietary energy harvest. Dietary fructo-oligo-saccharides increased the proportion of bifidobacteria in the distal intestine, leading to increased colonic fermentation and glucagon-like peptide 1 (GLP1) levels, and decreased food intake, fat mass, and hepatic steatosis [31-33].
Third, obese gut microbiota seem to provoke chronic low-grade inflammation in the host gut [34-36]. Chronic experimental metabolic endotoxemia-induced obesity, diabetes, and liver insulin resistance triggered the expression of inflammatory factors similar to those associated with a high-fat diet by a CD14-dependent mechanism [34]. According to de La Serre et al. [35], obesity in rats induced by a high-fat diet changed the gut microbiota and increased toll-like receptor 4 (TLR4) activation. They hypothesized that the activated TLR4 provoked gastrointestinal inflammation that was associated with the appearance of hyperphagia and an obese phenotype.
As the recent studies indicate, obesity and diet could be associated with altered gut microbiota characterized by a high Firmucutes to Bacteroidetes ratio and a dramatic fall in overall microbial diversity [37]. The altered gut microbiota could, in turn, alter the host metabolic potential. Therefore, the gut microbiota might contribute to obesity through increases in the harvest of energy from diet, gut permeability, and fat deposition in adipose tissue and the liver.
NAFLD is a multifactorial disease, the incidence of which is increasing, and the underlying mechanisms are incompletely understood. Various genetic, metabolic, inflammatory and environmental factors are thought to contribute to its pathogenesis [10]. Many recent human and animal studies have investigated possible relationships between the gut microbiota and NAFLD [27,34,38-43]. Animal studies suggest that the gut microbiota can initiate hepatic steatosis through an increase in monosaccharide absorption [27], bacterial hepatotoxic bioproducts [38], chronic low-grade metabolic inflammatory reactions [34,39], and modulation of bile acid metabolism [40].
The gut microbiota influences body fat deposition as an environmental factor [27]. Bäckhed et al. [27] reported that conventionalized mice had higher liver triglyceride contents than germ-free mice. In that study, the microbiota were associated with higher monosaccharide absorption from the intestinal lumen, promoting de novo fatty acid synthesis and triglyceride production, as confirmed by increased activity of acetyl-CoA carboxylase and fatty acid synthase. Synthesis of bacterial bioproducts, including ethanol, is increased in obese mice and may be involved in the pathogenesis of fatty liver disease [38]. As with obesity, chronic metabolic inflammatory reactions associated with CD14-dependent mechanisms, TLR4 signaling and hepatic Kuppfer cell activation seem to contribute to the pathogenesis of NAFLD [34,39].
Gut microbiota can also modulate bile acid metabolism. Swann et al. [40] reported that the microbiota can indirectly promote hepatic steatosis and lipoperoxidation through farnesoid X receptor stimulation changes in bile acid secretion.
Several human studies have also reported that gut microbiota contributed to NAFLD pathogenesis [41-43] and that bacterial overgrowth in obese patients was associated with hepatic steatosis [41]. Miele et al. [42] reported that NAFLD in humans was associated with increased gut permeability related to bacterial overgrowth in the small bowel and disruption of intestinal mucosa intercellular tight junctions. Consequently, small intestinal bacterial overgrowth in human NAFLD might contribute to hepatic fat deposition through increased intestinal permeability caused by disrupted intestinal tight junctions. Finally, as in NAFLD studies in animals, chronic endotoxemia in humans has been associated with the severity of NAFLD [43].
The available evidences show that bacterial proliferation and increased intestinal permeability contribute to the development of NAFLD through hepatotoxic bioproducts, modulation of bile acid metabolism, and chronic metabolic endotoxemia with involvement of bacterial lipopolysaccharide, CD14, TLR4 and Kuppfer cell-dependent mechanisms.
Obese subjects have a specific intestinal microbiota, which can harvest energy from the diet more effectively with greater synthesis of fatty acid by peripheral adipose tissue and the liver through several pathways (Fig. 1). Modulation of gut microbiota has been suggested as a treatment for obesity and NAFLD, using probiotics, prebiotics and synbiotics [45], but this needs further study.
Figures and Tables
Fig. 1
Schematic view of the possible mechanisms linking gut microbiota to obesity and nonalcoholic metabolic liver disease. Adapted from Tsukumo et al. Arq Bras Endocrinol Metabol 2009;53:139-44 [44].
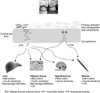
References
1. Conterno L, Fava F, Viola R, Tuohy KM. Obesity and the gut microbiota: does up-regulating colonic fermentation protect against obesity and metabolic disease? Genes Nutr. 2011. 6:241–260.


2. Oh K, Jang MJ, Lee NY, Moon JS, Lee CG, Yoo MH, et al. Prevalence and trends in obesity among Korean children and adolescents in 1997 and 2005. Korean J Pediatr. 2008. 51:950–955.


3. Cho SJ, Kim EY, Rho YI, Yang ES, Park YB, Moon KR, et al. The long-term follow-up studies of childhood obesity after puberty. Korean J Pediatr Gastroenterol Nutr. 2003. 6:47–53.


4. Frazier TH, DiBaise JK, McClain CJ. Gut microbiota, intestinal permeability, obesity-induced inflammation, and liver injury. JPEN J Parenter Enteral Nutr. 2011. 35:5 Suppl. 14S–20S.


5. Chang JH, Kim DH, Kim HS, Choi IK, Cheong MY, Kim DK. Prevalence of metabolic syndrome in obese children. Korean J Pediatr. 2004. 47:1149–1156.
6. Nho HN, Kim CR, Uhm JH, Kim JT, Jin SM, Seo JY, et al. The prevalence of obesity and metabolic abnormalities in Korean pediatric population. Korean J Pediatr Gastroenterol Nutr. 2009. 12:207–214.


8. Ley RE, Peterson DA, Gordon JI. Ecological and evolutionary forces shaping microbial diversity in the human intestine. Cell. 2006. 124:837–848.


9. Lyra A, Lahtinen S, Tiihonen K, Ouwehand AC. Intestinal microbiota and overweight. Benef Microbes. 2010. 1:407–421.


10. Abu-Shanab A, Quigley EM. The role of the gut microbiota in nonalcoholic fatty liver disease. Nat Rev Gastroenterol Hepatol. 2010. 7:691–701.


11. Machado MV, Cortez-Pinto H. Gut microbiota and nonalcoholic fatty liver disease. Ann Hepatol. 2012. 11:440–449.


13. Bäckhed F, Ley RE, Sonnenburg JL, Peterson DA, Gordon JI. Host-bacterial mutualism in the human intestine. Science. 2005. 307:1915–1920.


14. Clemente JC, Ursell LK, Parfrey LW, Knight R. The impact of the gut microbiota on human health: an integrative view. Cell. 2012. 148:1258–1270.


15. Krznarić Z, Vranešić Bender D, Kunović A, Kekez D, Stimac D. Gut microbiota and obesity. Dig Dis. 2012. 30:196–200.


16. Zoetendal EG, Vaughan EE, de Vos WM. A microbial world within us. Mol Microbiol. 2006. 59:1639–1650.


17. Diamant M, Blaak EE, de Vos WM. Do nutrient-gut-microbiota interactions play a role in human obesity, insulin resistance and type 2 diabetes? Obes Rev. 2011. 12:272–281.


18. Ottman N, Smidt H, de Vos WM, Belzer C. The function of our microbiota: who is out there and what do they do? Front Cell Infect Microbiol. 2012. 2:104.


19. Ley RE, Bäckhed F, Turnbaugh P, Lozupone CA, Knight RD, Gordon JI. Obesity alters gut microbial ecology. Proc Natl Acad Sci U S A. 2005. 102:11070–11075.


20. Turnbaugh PJ, Hamady M, Yatsunenko T, Cantarel BL, Duncan A, Ley RE, et al. A core gut microbiome in obese and lean twins. Nature. 2009. 457:480–484.


22. Kalliomäki M, Collado MC, Salminen S, Isolauri E. Early differences in fecal microbiota composition in children may predict overweight. Am J Clin Nutr. 2008. 87:534–538.


23. Balamurugan R, George G, Kabeerdoss J, Hepsiba J, Chandragunasekaran AM, Ramakrishna BS. Quantitative differences in intestinal Faecalibacterium prausnitzii in obese Indian children. Br J Nutr. 2010. 103:335–338.


24. Karlsson CL, Onnerfält J, Xu J, Molin G, Ahrné S, Thorngren-Jerneck K. The microbiota of the gut in preschool children with normal and excessive body weight. Obesity (Silver Spring). 2012. 20:2257–2261.


25. Luoto R, Kalliomäki M, Laitinen K, Delzenne NM, Cani PD, Salminen S, et al. Initial dietary and microbiological environments deviate in normal-weight compared to overweight children at 10 years of age. J Pediatr Gastroenterol Nutr. 2011. 52:90–95.


26. Luoto R, Kalliomäki M, Laitinen K, Isolauri E. The impact of perinatal probiotic intervention on the development of overweight and obesity: follow-up study from birth to 10 years. Int J Obes (Lond). 2010. 34:1531–1537.


27. Bäckhed F, Ding H, Wang T, Hooper LV, Koh GY, Nagy A, et al. The gut microbiota as an environmental factor that regulates fat storage. Proc Natl Acad Sci U S A. 2004. 101:15718–15723.


28. Bäckhed F, Manchester JK, Semenkovich CF, Gordon JI. Mechanisms underlying the resistance to diet-induced obesity in germ-free mice. Proc Natl Acad Sci U S A. 2007. 104:979–984.


29. Turnbaugh PJ, Ley RE, Mahowald MA, Magrini V, Mardis ER, Gordon JI. An obesity-associated gut microbiome with increased capacity for energy harvest. Nature. 2006. 444:1027–1031.


30. Samuel BS, Shaito A, Motoike T, Rey FE, Backhed F, Manchester JK, et al. Effects of the gut microbiota on host adiposity are modulated by the short-chain fatty-acid binding G protein-coupled receptor, Gpr41. Proc Natl Acad Sci U S A. 2008. 105:16767–16772.


31. Daubioul CA, Taper HS, De Wispelaere LD, Delzenne NM. Dietary oligofructose lessens hepatic steatosis, but does not prevent hypertriglyceridemia in obese zucker rats. J Nutr. 2000. 130:1314–1319.


32. Delzenne NM, Cani PD. A place for dietary fibre in the management of the metabolic syndrome. Curr Opin Clin Nutr Metab Care. 2005. 8:636–640.


33. Piche T, des Varannes SB, Sacher-Huvelin S, Holst JJ, Cuber JC, Galmiche JP. Colonic fermentation influences lower esophageal sphincter function in gastroesophageal reflux disease. Gastroenterology. 2003. 124:894–902.


34. Cani PD, Amar J, Iglesias MA, Poggi M, Knauf C, Bastelica D, et al. Metabolic endotoxemia initiates obesity and insulin resistance. Diabetes. 2007. 56:1761–1772.


35. de La Serre CB, Ellis CL, Lee J, Hartman AL, Rutledge JC, Raybould HE. Propensity to high-fat diet-induced obesity in rats is associated with changes in the gut microbiota and gut inflammation. Am J Physiol Gastrointest Liver Physiol. 2010. 299:G440–G448.


36. Cani PD, Possemiers S, Van de Wiele T, Guiot Y, Everard A, Rottier O, et al. Changes in gut microbiota control inflammation in obese mice through a mechanism involving GLP-2-driven improvement of gut permeability. Gut. 2009. 58:1091–1103.


37. Spencer MD, Hamp TJ, Reid RW, Fischer LM, Zeisel SH, Fodor AA. Association between composition of the human gastrointestinal microbiome and development of fatty liver with choline deficiency. Gastroenterology. 2011. 140:976–986.


38. Cope K, Risby T, Diehl AM. Increased gastrointestinal ethanol production in obese mice: implications for fatty liver disease pathogenesis. Gastroenterology. 2000. 119:1340–1347.


39. Rivera CA, Adegboyega P, van Rooijen N, Tagalicud A, Allman M, Wallace M. Toll-like receptor-4 signaling and Kupffer cells play pivotal roles in the pathogenesis of non-alcoholic steatohepatitis. J Hepatol. 2007. 47:571–579.


40. Swann JR, Want EJ, Geier FM, Spagou K, Wilson ID, Sidaway JE, et al. Systemic gut microbial modulation of bile acid metabolism in host tissue compartments. Proc Natl Acad Sci U S A. 2011. 108:Suppl 1. 4523–4530.


41. Sabaté JM, Jouët P, Harnois F, Mechler C, Msika S, Grossin M, et al. High prevalence of small intestinal bacterial overgrowth in patients with morbid obesity: a contributor to severe hepatic steatosis. Obes Surg. 2008. 18:371–377.


42. Miele L, Valenza V, La Torre G, Montalto M, Cammarota G, Ricci R, et al. Increased intestinal permeability and tight junction alterations in nonalcoholic fatty liver disease. Hepatology. 2009. 49:1877–1887.


43. Verdam FJ, Rensen SS, Driessen A, Greve JW, Buurman WA. Novel evidence for chronic exposure to endotoxin in human nonalcoholic steatohepatitis. J Clin Gastroenterol. 2011. 45:149–152.

